Open Access
ARTICLE
Nitrogen and Phosphorus Pollutants Removal from Rice Field Drainage with Ecological Agriculture Ditch: A Field Case
1 College of Agricultural Engineering, Hohai University, Nanjing, 210098, China
2 Key Laboratory of Lower Yellow River Channel and Estuary Regulation, Ministry of Water Resources, Zhengzhou, 450003, China
3 Key Laboratory of Integrated Regulation and Resource Development on Shallow Lake of Ministry of Education, College of Environment, Hohai University, Nanjing, 210098, China
4 College of Environment, Hohai University, Nanjing, 210098, China
5 Jiangsu Engineering Consulting Center, Nanjing, 210000, China
6 Yellow River Institute of Hydraulic Research, Zhengzhou, 450003, China
7Gaochun Water Resources Management Center, Nanjing, 211300, China
* Corresponding Author: Junyi Tan. Email:
Phyton-International Journal of Experimental Botany 2022, 91(12), 2827-2841. https://doi.org/10.32604/phyton.2022.024105
Received 24 May 2022; Accepted 10 August 2022; Issue published 29 August 2022
Abstract
Excessive nitrogen and phosphorus in agricultural drainage can cause a series of water environmental problems such as eutrophication of water bodies and non-point source pollution. By monitoring the water purification effect of a paddy ditch wetland in Gaochun, Nanjing, Jiangsu Province, we investigated the spatial and temporal distribution patterns of N and P pollutants in paddy drains during the whole reproductive period of rice. Then, the dynamic changes of nitrogen and phosphorus in time and space during the two processes of rainfall after basal fertilization and topdressing were analyzed after comparison. At last, the effect of the ditch wetland on nutrient purification and treatment mechanism, along with changing flow and concentration in paddy drains, was clarified. The results of this study showed that the concentrations of various nitrogen and phosphorus in the ditch basically reached the peak on the second and third days after the rainfall (5.98 mg/L for TN and 0.21 mg/L for TP), which provided a response time for effective control of nitrogen and phosphorus loss. The drainage can be purified by the ecological ditch, about 89.61%, 89.03%, 89.61%, 98.14%, and 79.05% of TN, NH4+-N, NO3−-N, NO2−-N, and TP decline. It is more effective than natural ditches for water purification with 80.59%, 40%, 12.07%, 91.06% and 18.42% removal rates, respectively. The results of the study can provide a theoretical basis for controlling agricultural non-point source pollution and improving the water environment of rivers and lakes scientifically.Keywords
Rice is an important source of food for most of the world’s population [1,2]. As the scale of rice cultivation continues to expand, the amount of fertilizer applied in the rice field also continues to increase. The intensive application of conventional fertilizers can cause severe environmental problems such as groundwater pollution, soil degradation, and water eutrophication [3]. Non-point source pollution of agricultural land is an important factor affecting the surface water environment. Storm water erosion and surface runoffs are important ways of fertilizer loss from agricultural fields and the major causes of nitrogen and phosphorus pollution [4,5], especially in southern China where rainfall is abundant. Taking paddy fields as an example, if nitrogen fertilizer is drained within 24 h after application to paddy fields, a considerable portion of the nitrogen is removed with the water. The disorganized paddy drainage has a great impact on the water quality of downstream water bodies [6].
In recent years, the important role of ditch wetland ecosystems has been widely recognized [7,8]. Ditches are the channels through which farmland drainage flows into rivers and lakes, and are the initial convergence point for agricultural non-point pollutants; at the same time, ditches, as an important part of farmland ecosystems, have a detaining and purifying effect on pollutants [9]. It has been shown that ecological ditches are significantly more effective than engineered ditch systems in intercepting and removing N migration from agricultural drainage [10]. Moreover, the ecological interception of pollutants in farmland by ditch wetlands can not only effectively reduce the pollution of downstream water bodies caused by N loss from farmland [11], but also improve the regional ecological environment; moreover, the ditch plants can also be covered and returned to the field to achieve the recycling of nutrient resources [12].
Research and application of ditch wetland systems to improve water quality in agricultural runoff and protect environmental ecosystems have flourished in China and abroad. Ryan et al. [13] studied the spatial and temporal variation of water quality in agricultural drainage ditches and concluded that water quality varies not only with seasonal but also with spatial (ditch location) variation. David et al. [14] investigated the mechanism of nutrient removal from wetlands such as N and P, and laid the foundation for the study of ditch wetland systems. Braskerud [15] showed that wetlands can effectively remove pollutants such as organic nitrogen and phosphorus when farmland drainage carries a large amount of suspended matter. Gu [16] studied key technologies such as the construction of ecological ditch spatial form, and the improvement of self-purification capacity of water bodies and water quality improvement. Wang et al. [17] designed an ecological interception ditch system with a reduction rate of more than 40% for nitrogen and phosphorus pollutants, the ability of wetlands to remove pollutants was significantly correlated with the content of the major pollutants in the incoming water [18]. The efficient operation of wetlands is often based on specific pollutants loads as well as steady flows, but unsteady flows may lead to changes in the pollutant removal capacity of wetlands. Unsteady flow, i.e., incoming flow with uncertainty in both flow and source intensity, includes uncertainty in the timing of the occurrence of rainfall runoff, the amount of incoming water, and the major pollutants and concentrations of the input. Therefore, the removal capacity and mechanisms of nutrients, such as nitrogen and phosphorus for wetlands receiving uncertain flows from unorganized discharges, still need further research refinement.
In this paper, we analyze the removal effects of ecological ditches and natural ditch wetlands on nitrogen, and phosphorus pollutants, and analyze the removal mechanisms in view of the uncertainty characteristics of paddy field non-point source generation, starting from the source of paddy field non-point source generation, the loss process and the loss path. The results will be able to provide technical support for improving the purification effect of paddy field receding water.
The experimental rice field was located at the trial site in Xi Town (west of Gucheng Lake), Gaochun County, Nanjing City, Jiangsu Province. The area belongs to the transitional monsoon climate zone of the north and middle subtropics, with an average annual precipitation of about 1692.7 mm, an average annual temperature of 17.5°C, and an annual sunshine time of 1654.5 h. The soil is mainly cyan soil, white soil, and loess in paddy fields. The altitude of the area is only 5–10 m.
The experiment was conducted from June to October, during which the minimum and maximum temperatures were 16°C and 35°C. The paddy field was fertilized with the conventional application amount in this study. And the fertilizer application method was basal fertilizer and topdressing twice. The fertilizer application amount for each fertilization period of rice is shown in Table 1, such as basal fertilizer, tillering fertilizer and panicle fertilizer. The basal fertilizer was applied one week for all the experimental fields around June 04, with irrigation and planting carried out simultaneously. Rice was irrigated by using the conventional local basin irrigation regime, as shown in Table 2. Rainfall started on June 04, there was field drainage on June 05, and the rain stopped on June 06 with a rainfall of 22 mm. The first topdressing was completed on July 01. Rainfall started on July 03.
2.2 Ecological Agriculture Ditch Setting
A typical hydrological year, in which the annual runoff is close to the multi-year average, was selected to carry out a research experiment on the effect of the ditch wetland on N and P purification of paddy wastewater in a rice field. The total length of the ecological ditch in the test section was 404 m, and it was divided into three sections according to the field conditions, which were called Ditch I, Ditch II and Ditch III in order of water flow direction. Ditch I was 109 m long and 7.5 m wide, Ditch II was 150 m long and 7.5 m wide, Ditch III was 145 m long and 8 m wide, and the average water level was 1.5 m. G1, G2, and G3 represented Ditch I, Ditch II and Ditch III. G1-1, G1-2, G2-1, G2-2, G3-1, and G3-2 represented the G1, G2 and G3 ditch water quality sampling section. The ditch flow direction was Q direction, as shown in Fig. 1 arrow direction. The drainage of the paddies enters the ditches from both sides.
Figure 1: Location of ecological agriculture ditches
Rice was fertilized with basal fertilizer before planting, followed by two topdressing. In the experimental area, the base fertilizer was applied around June 04, and all the experimental fields were fertilized within one week, and irrigation and rice planting were carried out at the same time. The first topdressing was carried out around June 29, and the second topdressing was carried out around August 01. The month from August 05 to September 05 was selected as a control experiment with different plant amounts during the rice season when the plant coverage was 50% in section G1 and 90% in section G2. There were no agricultural activities during this period, and it was considered that there was no exogenous input, and the water bodies in the ditches remained static, and both G1 and G2 were wooden fence-type ecological ditches (Fig. 2).
Figure 2: Newly constructed ecological ditch
At the same time, the experiments of nitrogen and phosphorus removal in ecological and natural ditches were done. G1 and G2 were both wooden fence ecological ditches, and G3 was a natural ditch, which is a traditional earthen ditch that is not artificially modified. Seventy percent of the water surface was covered by plants on average in G1 and G2, and about 60% in G3, but the density of plants on the berms of G1 and G2 ditches was higher than that of G3, and the growth was better than that of G3 (Figs. 3 and 4). The average value of pollutant concentrations in sections G1 and G2 were used as the concentration values of the ecological ditch, and the monitoring data in section G3 were the concentration values of the natural ditch.
Figure 3: Ecological ditch after plant growth
Figure 4: Natural ditch
2.3 N, P Pollutants Measurement Methods
The measurement indexes of the water samples included total nitrogen (TN), nitrate nitrogen (NO3−-N), nitrite nitrogen (NO2−-N), ammonia nitrogen (NH4+-N), total phosphorus (TP), total dissolved phosphorus (TDP). The detailed measurement methods were referred to the “Analytical Methods for Water and Wastewater Monitoring”, prepared by the State Environmental Protection Administration [19]. The experimental measurement items and methods are shown in Table 3.
Statistical analysis of the mass concentration of pollutants in the paddy drainage from ditches was conducted using Origin2021.
3.1 Characterization of N and P Dynamics in Ecological Agriculture Ditch after Fertilization
One-way ANOVA was conducted on the measured pollutant concentration data of the three groups, and the results showed that the difference between the concentration data of each group was significant. The loss of nitrogen and phosphorus in the field surface drainage, produced by rainfall after rice fertilization, is severe. The average concentration of TN was 6.113 mg/L, NH4+-N was 3.827 mg/L, NO3−-N was 1.77 mg/L, NO2−-N was 0.265 mg/L, TP was 0.317 mg/L, and TDP was 0.123 mg/L from 9:00 a.m. on July 2 to 5:00 p.m. on July 04 after topdressing. It is clear that NH4+-N was the main form of nitrogen loss, accounting for 62.60% of the TN. NO3−-N and NO2−-N accounted for 28.95% and 4.34%, respectively. Granular phosphorus was the main form of this rainfall runoff loss, accounting for 61.20% of the TP content, and TDP accounted for 38.80%.
The line charts of each nitrogen in the ecological ditch under rainfall conditions after paddy fertilization showed (Fig. 5) that all forms of nitrogen showed an increasing trend during this fertilization rainfall process, which was due to a large amount of fertilizer loss in the paddy field with field surface water and underground seepage water discharged into the ditch. The numbers in the X-axis represent the date, e.g., 6.4 represents June 04. Specifically, TN in the three ditches increased from 0.40–2.48 mg/L at 5 p.m. on June 04 to 3.71–5.64 mg/L at 9 a.m. on June 05, and the TN concentration remained near 5.5 mg/L for three days after that, without decreasing trend. NH4+-N in the ditch reached the maximum value of about 4.7 mg/L on June 05, and the decrease in NH4+-N concentration was also not obvious in the following 3 days. And the peak of NO3−-N appeared at 9 a.m. on June 06, 2.95 mg/L for G1, 1.83 mg/L for G2, and 1.48 mg/L for G3. On the contrary, the concentration of NO3−-N decreased by June 08, still larger than the previous concentration of about 1.0 mg/L. The peak of NO3−-N appeared after the rainfall started for a longer time, due to the primary source of NO3−-N was the nitrification of NH4+-N and the input of underground seepage water. NO2−-N was rising from 5 p.m. on June 04 to 9 a.m. on June 08, with the highest concentration reaching 0.15 mg/L, the trend of concentration falling back was not obvious, and its concentration was 0.14 mg/L on June 08, it is the result of the concentration of NH4+-N still remained at a high level and the intensity of nitrification in the water body was greater. After the topdressing, only NO3−-N and NO2−-N showed a significant increase. Specifically, the TN concentration fluctuated with time, mainly caused by the unstable flow and field surface drainage water concentration that sank into the ditch. The NH4+-N in the ditch (1–3.5 mg/L) showed similar fluctuations as TN, and NH4+-N in the wetland remained around 0.7 mg/L. The peak of NO3−-N about 2.7 mg/L appeared at 3 p.m. on July 05. The rise of NO3−-N also appeared in the second half of the fertilization rainfall process, due to the conversion of NH4+-N into NO3−-N by nitrification and the input of underground seepage water. The overall trend of nitrate-nitrogen was from high to low and then rising again, with the highest concentration of 0.28 mg/L and the lowest concentration of 0.07 mg/L.
Figure 5: Characteristics of N after basal fertilization and topdressing
The curves of each phosphorus in the ecological agriculture ditch under rainfall conditions after paddy fertilization showed (Fig. 6) that TP and TDP in the ditch system reached the maximum value of 0.26 and 0.21 mg/L after 24 h of the rainfall beginning. Then it would gradually decrease to about 0.06 and 0.02 mg/L for TP and TDP on June 8. In addition, TP and TDP in the ditches were the same over time, indicating that the paddy drainage ditch system also has a good retention effect on elemental phosphorus. Its retention pattern is not affected by spatial location. The peak concentration of each phosphorus was basically reached on the 2nd and 3rd day after rainfall. After the topdressing, the TP in the ditch system was parabolic like throughout the process, with the highest point being about 0.23 mg/L. The TP in the dissolved state mainly fluctuated between 0.02 and 0.08 mg/L. The phosphorus that entered the ditch during the rainfall process was primarily granular, accounting for 61.20% of the TP, probably because of the field topsoil erosion during the rainy day. Similarly, this pattern provided the best control time for effective phosphorus loss control. After the application of fertilizer, the indicators of the surface flow wetland did not show substantial changes, which is due to the relatively large area of the surface flow wetland and the sampling section at the outlet of the wetland. The water in the ecological agriculture ditch has a certain residence time into the surface flow wetland, which has a better purification effect on water quality. So, the monitoring data at the outlet of the surface flow wetland has a slight variation.
Figure 6: Characteristics of P after basal fertilization and topdressing
3.2 Water Purification Effect of Ecological Agriculture Ditch under Different Phytomass
The ability of ecological ditches to reduce nitrogen and phosphorus in paddy drainage was monitored simultaneously during the experiment under different plant cover conditions, with 50% plant cover in section G1% and 90% plant cover in section G2. It can be considered there is no exogenous input because there was no agricultural activity during this period. To explore the effect of plant amount on ditch purification efficiency, the concentrations of each pollutant on August 05 and September 05 were calculated and one-way ANOVA was conducted, and the results showed that there was a significant difference in the concentration change of each pollutant under 50% plant cover and 90% plant cover, except for TP on September 05.
The comparison of the changes of each nitrogen with different plant amounts showed ((a) to (d) in Fig. 7) that the concentration of TN, NH4+-N, NO3−-N, and NO2−-N decreased in the canal sections with different plant cover during the experiment. The 8.05 and 9.05 in the Figure represent the dates August 05 and September 05. Overall, TN decreased by 2.741 mg/L in the canal section with 50% cover and decreased by 3.488 mg/L in the canal section with 90% plant cover. Additionally, the plants have a greater demand for nitrogen due to growth needs in canal sections so TN reduction is stronger with a large cover. The decrease of NH4+-N was 0.036 mg/L in the canal section with 50% coverage and 0.050 mg/L in the canal section with 90% plant coverage. NO3−-N was reduced by 1.28 mg/L with 50% coverage and 1.55 mg/L in the canal section with 90% plant coverage in the canal section. However, NO2−-N was reduced by 0.104 mg/L in canals with 50% cover and by 0.053 mg/L in canals with 90% plant cover. Its reduction was lower in canals with large plant cover than in canals with small ones, mainly because many plants become slow to grow when the cover reaches 90%.
Figure 7: Comparison of N and P changes in different plant amounts
The comparison of the changes of each phosphorus with different plant amounts showed ((e) and (f) in Fig. 7) that the concentration of TP decreased in the canal sections with additional plant cover. But the concentration of dissolved TP increased. Specifically, TP decreased by 0.026 mg/L in the canal section with 50% coverage and 0.068 mg/L in the canal section with 90% plant coverage. The removal capacity of TP in the canal section with an extensive range was greater than that in the canal section with small coverage, which may be controlled by the fact that more plants can reduce the disturbance of the water body. The particulate phosphorus was easy to set because of the many more system obstacles. TDP increased by 0.0013 mg/L in the canal section with 50% coverage and 0.002 mg/L in the canal section with 90% plant coverage, both of which were small. Due to the low concentration of TDP in August, the particulate phosphorus would release from the sediment to achieve the dynamic equilibrium between particulate phosphorus and TDP in the water column.
3.3 Analysis of the Effect of Different Types of Ditches on Water Treatment
In order to study the effect of different types of ditches on the removal rate of pollutants, the removal rate of each pollutant in the ecological ditch and the natural ditch was calculated, and one-way ANOVA was conducted. The result showed that the removal rate of pollutants was significantly different in ecological ditches and natural ditches. And the histogram was plotted, i.e., Fig. 8. The comparison of the decontamination capacity of the ecological ditch with wooden fence wall and ecological ditch with soil wall in the rice season (Fig. 8) showed that the wooden fence ecological ditch had better pollutants removal capacity than the ecological soil ditch for different nitrogen and phosphorus except for TDP. The removal rate of TN was 89.61% in the ecological ditch and 80.59% in the natural ditch. The removal rate of NH4+-N was 89.03% and 40% in the wooden fence ecological ditch and soil ecological ditch, respectively. The removal rate of NO3−-N, NO2−-N, TP, TDP was 89.61% and 12.07%, 98.14% and 91.06%, 79.05% and 18.42%, −29.17% and −4.6%, for ecological ditch with wooden fence wall or soil wall respectively. In general, the wooden fence ecological ditch had a better water purification effect than the ecological soil ditch in the rice season. The reduction of NH4+-N, NO3−-N, and TP was more prominent.
Figure 8: Comparison of pollution removal capacity of ditches with different material
4.1 Patterns of N and P Loss in Rice Field Drainage under Rainfall Conditions after Fertilizer Application
Rainfall was a violent force of surface soil erosion, especially in the early stages of rice growth, the crop’s root system was not developed holding weak attachment. So, a large amount of nitrogen and phosphorus was lost because a large amount of inorganic state nitrogen, organic state nitrogen, phosphorus was adsorbed on the soil’s surface. A large amount of dissolved NO3−-N and NH4+-N loss in the field surface water drains into the ditch was bad for downstream water quality [20]. The removal of phosphorus from ditch systems was accomplished by combining several aspects, including plant uptake, microbial accumulation, and substrate adsorption [21]. Some phosphorus in water bodies was soluble and readily absorbed by soil particles. Therefore, wetlands reduce phosphorus concentration in water by retaining sediments. In the case of rainfall, the transformation of phosphorus was complicated by the confluence of rainfall-runoff and subsurface seepage water and the instability of the ditch system, which resulted in a constant change in concentration. The gradual increase in phosphorus absorption by particles and substrate in the water and the impact-resistant therapeutic properties of the ditch system allowed for a rapid recovery of phosphorus transformation, which resulted in a concentration decrease for TP and TDP.
Similar conclusions have been obtained by other scholars. Li et al. [22] studied the characteristics of nitrogen mass concentration changes in typical rainfall-rice field drainage. The results showed that its maximum value usually appeared in a relatively short period of time after larger rainfall, and then its mass concentration gradually decreased and stabilized due to the effect of runoff dilution, similar to the results of this study. Jiao et al. [23] studied the characteristics of nitrogen loss in paddy field rainfall runoff water under fertilizer application conditions, and the results showed that rainfall loss after initial fertilizer application significantly reduced the mass concentration of nitrogen in paddy field drainage water. In that case, nitrogen was mainly lost as water-soluble NH4+-N. In this study, NH4+-N also had the largest proportion in paddy field drainage water, but the N concentration increased after rainfall, probably due to the difference in rainfall amount. This may be due to the difference in the amount of rainfall that caused the difference in the amount of nitrogen loss in the drainage water of the paddy field. In conclusion, the peak of nitrogen mass concentration in drainage water usually occurred in the early period after rainfall. Therefore, based on the prediction results of rainfall by meteorological forecasts, avoiding fertilizer application before the onset of rainfall and reasonably adjusting the drainage time at the early stage after rainfall. These measures will help to mitigate agricultural non-point source pollution emissions.
4.2 Effect of Plant Cover on the Purification Effect of Ditches
The absorption of nutrients by plants was the main way of purification. The huge surface area of plant roots and robust microorganisms were both major contributors to pollutant removal. At the same time, the anaerobic zone away from the root system was rich in dead debris, containing a large amount of available carbon sources and providing denitrification conditions. During the plant growing season, aquatic plants need to absorb some inorganic phosphorus during the plant growing season to maintain their life activities. Phosphorus was a limiting factor for plant growth. Activated microbial degradation of organic phosphorus was caused by vigorous plants and microorganisms [24]. The removal of NO3−-N in the canal section with a high range was greater than that in the canal section with low coverage, mainly because NO3−-N removal was primarily by denitrification, plant uptake, and infiltration. Denitrification was the only natural process that permanently removes nitrogen from wetland ecosystems as a major NO3− ion removal pathway [25].
Shi et al. [26] studied the effect of plant volume on the purification effect in wetlands, and the results showed that the plant volume of the same species was positively correlated with the removal rate of N-pollutants, while it was not significantly correlated with the removal rate of P-pollutants, which was basically consistent with the results of this study. Plants are important for maintaining the normal operation of the artificial wetland system, steadily removing pollutants, and creating ecological and landscape functions [27]. However, unscientific management and untimely harvesting of plants can lead to excessive biomass increase, which can cause problems such as clogging and affect hydraulic transport and reduce the performance of the wetland system [28]. Wu et al. [29] found that the removal rates of TN, NH4+-N, TP, and PO43−-P in the harvested artificial wetland system (40.0%, 56.6%, 35.7%, and 39.4%) were significantly higher than those in the unharvested one (34.6%, 51.0%, 29.1%, and 29.5%). Therefore, it was important to harvest the plants that have matured or even started to decay in the ditches in a timely manner so that the ditch purification efficiency was always maintained at a high level.
4.3 Effect of Ditch Type on the Removal Effect of Nitrogen and Phosphorus Pollutants
The reason why the wood fence ecological ditch had better treatment effect was that the berm of wood fence ecological ditch was similar to the composite berm. Plants’ mass on the berm was more than that in the natural ditch. The ecological ditch had a fixed berm, which could reduce berm sediment loss. In terms of application, ecological ditches did not additionally occupy land. The berm plants on the ditch walls could absorb nutrients from the side waters. The traditional type of soil ditch had poor soil retention capacity and was prone to soil erosion and ditch wall collapse. Although the modern concrete ditch modified some problems, the water speed was faster, and the soil and nutrients carried by the flowing water cannot be removed.
Other scholars have also investigated the interception effect of different types of ditches on nitrogen and phosphorus in farmland runoff water. Tian et al. [30] tested 3 types of agricultural drainage ditches (ecological interception ditch, simple soil drainage ditch, and concrete slabs type ditch), and the results showed that the average removal rate of total nitrogen and total phosphorus in ecological interceptor ditches reached 54.18% and 58.21%, respectively; the average removal rate of total nitrogen and total phosphorus in simple soil ditches of the same length in the same period were 31.91% and 38.46%, respectively, and 38.46% for TN and TP, respectively. While the average removal rate of concrete slab ditches for total N and total P were only 11.03% and 15.38%, which were similar to the results of this study. Wu [31] also found that the reduction of nitrogen in ecological ditches was stronger than that in concrete ditches as time increased; for phosphorus, the runoff phosphorus concentration in concrete ditches was higher than that in ecological ditches in the early runoff stage due to the scouring effect of runoff. Plants at the bottom of the ditch absorb nutrients in the water flow and facilitate the sedimentation of sediment and particulate matter carried in the water flow [32,33]. The degree of plant uptake and microbial degradation was different for different plants at different growth stages, and further research was needed to investigate the purification and interception mechanism of pollutants in runoff water by different plants and different plant growth stages.
When it rained after the basal fertilizer, applied in the rice season, the concentration of TN and TP in the ditch reached their peak after 2–3 days, with 5.98 and 0.21 mg/L, respectively. Nitrogen and phosphorus did not show a single decrease with time and space changing. Various transformations of nitrogen and phosphorus are restricted at the early stage. But, with the increase of time, this effect gradually decreases because the ditch system has a certain impact-resistant restoration effect.
After the first topdressing, the varying pattern of nitrogen and phosphorus along the course was not obvious once the rainfall occurred in the rice season. TN varied between 3.13–5.31 mg/L, and TP vary between 0.09–0.21 mg/L. These were mainly affected by the low concentration of exogenous sources of pollutants in the ditch.
Ecological ditch showed a significant removal effect for non-point pollutants. The purification effect of ecological ditches with wooden fence walls was better than that of the ecological ditch with soil wall. The removal efficiencies for TN, NH4+-N, NO3−-N, NO2−-N, and TP were 89.61%, 89.03%, 89.61%, 98.14%, and 79.05% in the ecological agriculture ditch with a wooden fence wall, and 80.59%, 40%, 12.07%, 91.06% and 18.42% in the ecological agriculture ditch using soil wall, respectively. Ecological agriculture ditch has a marked effect on drainage treatment than agriculture ditch in the rice field, especially for NH4+-N, NO3−-N, and TP removal with more than 50% increase. Totally, the ecological ditch not only plays the function of drainage and irrigation, but also reduces the loss of nutrients such as nitrogen and phosphorus from farmland, and has a good landscape effect, which has a greater potential for promotion.
There are many problems in the construction, operation and maintenance management of ecological ditches in engineering applications, as well as in the planting and harvesting of plants and the substrate cleaning in ecological ditches. Improper treatment can reduce the removal efficiency of ecological ditches and cause problems such as secondary pollution, so further research is still needed.
Funding Statement: The authors received no specific funding for this study.
Conflicts of Interest: The authors declare that they have no conflicts of interest to report regarding the present study.
References
1. Elshayb, O. M., Nada, A. M., Sadek, A. H., Ismail, S. H., Shami, A. et al. (2022). The integrative effects of biochar and ZnO nanoparticles for enhancing rice productivity and water use efficiency under irrigation deficit conditions. Plants, 11(11), 1416. DOI 10.3390/plants11111416. [Google Scholar] [CrossRef]
2. Rashecd, A., Seleiman, M., Nawaz, M., Mahmood, A., Anwar, M. R. et al. (2021). Agronomic and genetic approaches for enhancing tolerance to heat stress in rice: A review. Notulae Botanicae Horti Agrobotanici Cluj-Napoca, 49(4), 12501. DOI 10.15835/nbha49412501. [Google Scholar] [CrossRef]
3. Badawy, S. A., Zayed, B. A., Bassiouni, S. M. A., Mahdi, A., Majrashi, A. et al. (2021). Influence of nano silicon and nano selenium on root characters, growth, ion selectivity, yield, and yield components of rice (Oryza sativa L.) under salinity conditions. Plants, 10(8), 1657. [Google Scholar]
4. Sun, Y., Yu, S. G., Chen, J., Xiao, M., Wang, N. (2014). Changes of nitrogen and phosphorus concentrations in surface drainage from paddy field under different irrigation and drainage modes. Journal of Hohai University (Natural Sciences), 42(5), 455–459. [Google Scholar]
5. Zhang, Z. L., Liu, F., Hou, T. Y. (2019). Current status of nitrogen and phosphorus losses and related factors in Chinese paddy fields: A review. Chinese Journal of Applied Ecology, 30(10), 3292–3302. [Google Scholar]
6. Li, J. Y., Wei, Q., Wang, H. Y., Chen, P., Cheng, H. et al. (2020). Nitrogen loss with rainfall leaching from paddy field. Journal of Irrigation and Drainage, 39(12), 97–102. [Google Scholar]
7. Yin, L. M., Zhang, S. N., Li, B. Z., Duan, L. Z., Liu, F. (2014). Progress of the ditch wetland technologies in research mechanism and application of removing nitrogen from agricultural surface runoff. Research of Agricultural Modernization, 35(5), 583–587. [Google Scholar]
8. Kumwimba, M. N., Zhu, B., Muyembe, D. K. (2017). Estimation of the removal efficiency of heavy metals and nutrients from ecological drainage ditches treating town sewage during dry and wet seasons. Environmental Monitoring and Assessment, 189(9), 434. DOI 10.1007/s10661-017-6136-7. [Google Scholar] [CrossRef]
9. Zhang, S. N., Xiao, R. L., Liu, F., Wu, J. S. (2015). Interception effect of vegetated drainage ditch on nitrogen and phosphorus from drainage ditches. Environmental Science, 36(12), 4516–4522. [Google Scholar]
10. Wang, Y., Wang, J. G., Li, W. (2009). Comparison on removal of nitrogen and phosphorus form hibernal farmland drainage by three kinds of ditches. Soil, 41(6), 902–906. [Google Scholar]
11. You, H. L., Wu, Y. M., Liu, L. Z., Deng, M., Zhang, F. F. et al. (2020). Intercepting effect of ecological ditch on non-point source pollution in small rural watersheds. Environmental Science and Technology, 43(4), 130–138. [Google Scholar]
12. Wang, H. D. (2021). Study on the removal effect of ecological ditch on farmland runoff pollutants and the characteristics of biological community (Master Thesis). East China University of Science and Technology, China. [Google Scholar]
13. Ryan, E. C., Taylor, M. D., Stephen, J. K. (2001). Mechanisms of nutrient attenuation in a subsurface flow riparian wetland. Journal of Environmental Quality, 30(5), 1732–1737. DOI 10.2134/jeq2001.3051732x. [Google Scholar] [CrossRef]
14. David, A. K., David, M. B., Gentry, L. E. (2000). Effectiveness of constructed wetlands in reducing nitrogen and phosphorus export from agriculture tile drainage. Journal of Environmental Quality, 29, 1262–1274. DOI 10.2134/jeq2000.00472425002900040033x. [Google Scholar] [CrossRef]
15. Braskerud, B. C. (2002). Factors affecting nitrogen retention in small constructed wetlands treating agricultural non-point source pollution. Ecological Engineering, 18(3), 351–370. DOI 10.1016/S0925-8574(01)00099-4. [Google Scholar] [CrossRef]
16. Gu, B. J. (2006). Research on the construction technology of ecological ditch morphology in irrigation areas. China Rural Water Conservancy and Hydropower, 48(10), 4–6. [Google Scholar]
17. Wang, T., Zhu, B., Zhou, M. (2019). Ecological ditch system for nutrient removal of rural domestic sewage in the hilly area of the Central Sichuan Basin, China. Journal of Hydrology, 570, 839–849. DOI 10.1016/j.jhydrol.2019.01.034. [Google Scholar] [CrossRef]
18. Yang, S. B., Zheng, Y. C., Dzakpasu, M. W., Wang, X. C., Wang, W. D. (2020). Influence mechanism of pollutant loading on the performance of horizontal flow constructed wetlands for wastewater treatment. Industrial Water Treatment, 40(6), 48–50. [Google Scholar]
19. State Environmental Protection Administration (2002). Analytical methods for water and wastewater monitoring. 4th edition. Beijing, China: Environmental Science Press. [Google Scholar]
20. Guo, Z. H., Liu, Y., Huang, Y. M., Yan, J. T., Teng, F. et al. (2017). Effects of rainfall and fertilization on water quality of the Yujia river watershed in the Northern Qinling mountains. Journal of Agro-Environment Science, 36(1), 158–166. [Google Scholar]
21. Chang, X. Y., Zhang, S. N., Zhang, M. M., Xiao, B. R., Chen, J. L. (2021). Phosphorus removal in Myriophyllum aquaticum ditch under low-temperature conditions. Journal of Agro-Environment Science, 40(4), 852–858. [Google Scholar]
22. Li, J. Y., Qi, W., Wang, H. Y. (2020). Nitrogen loss with rainfall leaching from paddy field. Journal of Irrigation and Drainage, 39(12), 97–102. [Google Scholar]
23. Jiao, S. J., Hu, X. M., Pan, G. X. (2007). Effects of fertilization on nitrogen and phosphorus run-off loss from Qingzini paddy soil in Taihu lake region during rice growth season. Chinese Journal of Ecology, 26(4), 495–500. [Google Scholar]
24. Wang, P. F., Lou, M. Y., Qian, J., Hu, B. (2020). Analysis of purification effect and mechanism of pollutant by the farmland drainage wetland. Water Resources Protection, 36(5), 1–10. [Google Scholar]
25. He, P. (2021). Denitrification mechanism and its influencing factors in ponds of tianmu lake basin in the upper reaches of Taihu Lake (Master Thesis). Zhengzhou University, China. [Google Scholar]
26. Shi, L., Yang, X. (2010). Phytomass and its impact on the pollutant removal ability of constructed wetland. Journal of Ecology and Environment, 19(1), 28–33. [Google Scholar]
27. Guo, S. L., Ye, C., Li, C. H. (2015). A review of wetland ecosystem regulation research. Proceedings of the 2015 Annual Academic Conference of the Chinese Society of Environmental Sciences, no. 2, pp. 1958–1964. Shenzhen, China. [Google Scholar]
28. Li, C., Li, S. F. (2012). Research progress of artificial wetland plants. Municipal Technology, 4(30), 95–97. [Google Scholar]
29. Wu, X. Y., Wang, X. N., Guo, J., Zhao, Y. Q. (2021). Effects of plants harvesting in constructed wetland on pollutants removal and their utilization status. Proceedings of the 2021 Annual Scientific and Technical Conference of the Chinese Society of Environmental Science-Environmental Engineering Technology Innovation and Application Session (I), pp. 113–119. Tianjin, China. [Google Scholar]
30. Tian, S., Sha, Z. M., Yue, Y. B., Yuan, J., Lu, L. F. et al. (2016). Interception effect of different types of ditches on nitrogen and phosphorus loss from farmland. Jiangsu Agricultural Science, 44(4), 361–365. [Google Scholar]
31. Wu, X. N. (2013). Interception and removal effects of the different types of agriculture drainage ditches on nitrogen and phosphorus in Dianchi Lake watershed (Master Thesis). Yunnan University, China. [Google Scholar]
32. Xu, H., Su, G. J., Yuan, Y. K., Wu, J. Q., Tang, H. (2016). Impact of different layout of ecological ditches on pollutant removal efficiency from agricultural runoff. Yangtze River, 47(21), 9–14. [Google Scholar]
33. Ye, Y. M., Wu, C. F., Yu, J. (2011). Ecological design of irrigation and drainage ditches in agricultural land consolidation. Transactions of the Chinese Society of Agricultural Engineering, 27(10), 148–153. [Google Scholar]
Cite This Article
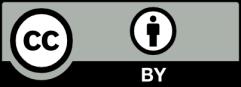
This work is licensed under a Creative Commons Attribution 4.0 International License , which permits unrestricted use, distribution, and reproduction in any medium, provided the original work is properly cited.