Open Access
REVIEW
The effect of concomitant drugs on oncological outcomes in patients treated with immunotherapy for metastatic urothelial carcinoma: a narrative review
1 Medical Oncology Unit, University Hospital of Parma, Parma, 43126, Italy
2 Department of Medicine and Surgery, University of Parma, Parma, 43126, Italy
3 Radiotherapy Unit, University Hospital of Parma, Parma, 43126, Italy
4 Department of Urology, University of Modena and Reggio Emilia, Modena, 41124, Italy
5 Urology Unit, University Hospital of Parma, Parma, 43126, Italy
* Corresponding Author: GIULIA CLAIRE GIUDICE. Email:
# These two authors contributed equally to this work
(This article belongs to the Special Issue: Advances in Cancer Immunotherapy)
Oncology Research 2025, 33(4), 741-757. https://doi.org/10.32604/or.2024.057278
Received 13 August 2024; Accepted 04 November 2024; Issue published 19 March 2025
Abstract
Background: immune checkpoint inhibitors (ICIs) have revolutionized the treatment of metastatic urothelial carcinoma (mUC), significantly improving survival outcomes. However, a subset of patients do not respond to ICIs, prompting research into potential predictive factors. Commonly prescribed medications such as corticosteroids, proton-pump inhibitors (PPIs), antibiotics (Abs), antihypertensives, and analgesics may influence ICI effectiveness. Methods: we conducted a literature search on PubMed to investigate the impact of concomitant medications on the outcomes of patients with mUC, treated with ICIs. We selected the most relevant studies and performed a narrative review. Results: corticosteroids, PPIs and Abs have been associated with reduced survival in ICI-treated patients, including those with mUC. In contrast, antihypertensive agents like renin-angiotensin system inhibitors and beta-blockers may enhance ICI efficacy, though evidence remains inconclusive. The impact of other medications, such as statins, metformin, and analgesics, on ICI outcomes is less clear, with some data suggesting a detrimental impact on immune response. Conclusions: this narrative review synthesizes current evidence on how concomitant medications affect outcomes in mUC patients treated with ICIs.Keywords
In recent years, immune checkpoint inhibitors (ICIs) targeting the programmed cell death protein-1 (PD-1) and its ligand (PD-L1) have dramatically improved survival outcomes for patients with metastatic urothelial carcinoma (mUC) [1]. Currently, ICIs are approved for use as first-line therapy in platinum-ineligible patients [2], as second-line treatment following progression on platinum-based chemotherapy [2], and as maintenance therapy after a response (or stable disease) to platinum-based chemotherapy [3]. Recently, combinations of nivolumab plus chemotherapy and pembrolizumab plus enfortumab vedotin compared to standard platinum-chemotherapy have shown to improve survival in first-line setting [4,5].
Despite these advancements, a portion of patients do not respond to ICIs, leading to extensive research aimed at identifying clinical or biological biomarkers predictive of ICI response [6]. Among the clinical factors influencing ICI effectiveness, concomitant medications have garnered increasing interest. Drug interactions and the potential anticancer effects of commonly used drugs may impact oncological outcomes of patients with several solid tumors, including urothelial carcinoma (UC), treated with ICIs [7].
Oncologic patients often have significant comorbidities and symptoms related to metastatic disease or adverse effects from therapy, thus requiring different types of concurrent drugs. Commonly prescribed medications include proton-pump inhibitors (PPIs), corticosteroids, antibiotics (Abs), anti-hypertensives, and analgesics [7].
Corticosteroids are frequently used to manage cancer-related symptoms (such as fatigue, dyspnoea, cerebral oedema, and pain), immune-related adverse events (irAEs), and autoimmune comorbidities, although they can induce an immunosuppressive state, potentially reducing ICI effectiveness. Available data suggest that steroid use before or shortly after ICI initiation is associated with poorer clinical outcomes, whereas steroid use for irAEs does not completely diminish the antitumor response of ICIs [8,9].
PPIs are among the most commonly prescribed medications in both cancer and non-cancer patients for chronic gastritis, gastric protection during treatment with steroids or non-steroidal anti inflammatory drugs and gastro-esophageal reflux. PPIs have also been linked to shortened survival outcomes in patients receiving ICIs for different solid tumors, including UC [10–14]. The mechanisms behind this influence may be mediated by a PPIs-induced reduction of gut microbiota diversity, which may ultimately impair the immune microenvironment and diminish the effectiveness of ICIs [15,16].
With a similar mechanism, the concomitant use of Abs can negatively impact survival outcomes of patients with solid tumors treated with ICIs, including those affected by advanced UC [17–21].
Recent data suggested that the concomitant use of antihypertensive drugs could affect ICI efficacy, although with controversial evidence. Specifically, angiotensin-converting enzyme inhibitors (ACEIs) and angiotensin receptor blockers (ARBs), as well as beta-blockers, have been shown to improve survival outcomes in patients with mUC undergoing ICI treatment [22–24]. However, some studies have reported no significant differences in outcomes for cancer patients receiving these drugs [25,26]. The underlying mechanisms are not fully understood. The renin-angiotensin-aldosterone system (RAS) may promote an immunosuppressive environment, therefore the inhibition of this pathway could enhance ICI efficacy [26].
Finally, acetaminophen and opioids are commonly prescribed for relieving cancer pain and fever, although it has been reported that they may act as a potential suppressor of antitumor immunity, potentially decreasing ICIs effectiveness [27,28].
This narrative review aims to summarize the available evidence regarding the impact of concomitant drugs on survival outcomes and responses in patients treated with ICIs for mUC.
This is a narrative review. We performed a literature search on PubMed (https://pubmed.ncbi.nlm.nih.gov/) (accessed on 03 November 2024) using various combinations of the following keywords or their synonyms: ‘concomitant’ and (‘antibiotics’ or ‘proton pump inhibitors’ or ‘anti-hypertensives’ or ‘corticosteroids’ or ‘metformin’ or ‘hypoglycemics’ or ‘statin’ or ‘acetaminophen’ or ‘opioids’ or ‘drugs’ or ‘medications’) and (‘immunotherapy’ or ‘immune checkpoint inhibitors’) and (‘urothelial cancer/carcinoma’ or ‘bladder cancer/carcinoma’). We considered abstracts, reviews, metanalyses, and clinical and observational studies, and selected the most relevant works based on their level of evidence.
Role of proton-pump inhibitors in modulating immune response
PPIs negatively impact on survival outcomes in many advanced solid tumors when administered concomitantly with ICIs [10–14] Through their direct inhibition of H+/K+-adenosine triphosphate (ATP)ase pump, which in turn reduces gastric acidity, PPIs can induce gut dysbiosis, eventually leading to a detrimental effect on immunotherapy effectiveness [12]. In fact, several studies reported that intestinal microbiota had a significant impact on immune system and ICIs response. The influence of the intestinal microbiota on anticancer immune response can vary depending on microbial species: bacteria found in ICIs responders showed different types of immune modulations, such as Bifidobacterium fragilis, which activated T-helper1 cells and cross-reactivity between bacterial and tumor antigens [12]; it was also observed that gut microbiota could induce cluster differentiation (CD)8+ T cell activation and promote CD4+ T cell differentiation, while reducing Treg levels [29]. The population of bacteria associated with improved response to ICIs (e.g., Bifidobacterium sp, Ruminococcaceae, Akkermansia muciniphila and Alistipes sp) was found to be decreased by PPIs treatment; on the contrary, bacteria associated with resistance to ICIs, such as oral cavity microorganisms (e.g., Actinomyces spp., Rothia mucilaginosa, Rothia dentocariosa) and Bacteroidetes and Escherichia coli, were increased, due to the reduction of gastric acid secretion that would normally prevent translocation and accumulation of both ingested microbial pathogens and commensal microorganisms [12]. Tomita et al. retrospectively evaluated the beneficial role of a live biotherapeutic bacterial strain (Clostridium butyricum, CBM588) administered in 118 patients with advanced or recurrent non-small cell lung cancer (NSCLC) treated with ICIs and concomitant PPIs [30]. The addition of CBM588 strain led to an improvement on both progression-free survival (PFS) (median PFS: 250 days vs. 88 days, Hazard Ratio (HR) 0.52, p = 0.030) and overall survival (OS) (median OS, not reached (NR) vs. 208 days, HR 0.42, p = 0.030) [30].
Other studies exploring the potential role of PPIs in affecting ICIs response showed a direct impact in influencing tumor microenvironment (TME) in many different cancer types. In fact, both in vitro and in vivo studies demonstrated that PPIs can induce mitochondrial apoptosis and impair tumor growth through inhibition of V-ATPase activity, which regulates intracellular pH homeostasis [31,32]. In addition, a translational study conducted by Gao et al. on murine models, revealed that PPIs could promote PD-L1 protein expression and stability by inducing glycogen synthase kinase-(GSK)-3β phosphorylation, thus enhancing ICIs response [33].
Finally, PPIs may also directly impact inflammatory responses by reducing the secretion of adhesion molecules (e.g., intercellular adhesion molecule-1 and and vascular cell adhesion molecule-1), inhibiting cytokine production (e.g., interleukin-6 (IL-6) and tumor necrosis factor-α), and facilitating immune escape by increasing the translocation of PD-L1 to the tumor cell membrane [33,34]. Other preclinical studies showed that PPIs’ interference with the nuclear factor-κB (NF-κB) pathway in gastric mucosa cells could result in lower secretion of the potent neutrophil chemoattranct, IL-8 [35]. Moreover, PPIs could contrast neutrophils’ activity by inhibiting vacuolar (v-type) H+ATPases which are involved in the acidification of intracellular organelles like lysosomes, thus reducing the accumulation and release of reactive oxygen species (ROS) [36].
Role of proton-pump inhibitors in influencing survival outcomes in patients with metastatic urothelial carcinoma treated with immunotherapy
Many studies explored the impact of PPIs in patients with advanced UC treated with ICIs, Table 1.
A retrospective multicenter study by Fiala et al. analyzed data regarding concomitant PPIs in a cohort of 802 patients with mUC treated with pembrolizumab: the use of PPIs had a significantly detrimental effect on PFS (4.5 vs. 7.2 months, p = 0.002) and OS (8.7 vs. 14.1 months, p < 0.001) compared to non-use, even after adjustment in a multivariate Cox analysis [7]. The same results were confirmed in another retrospective study conducted on 1360 patients with advanced mUC treated with atezolizumab within the IMvigor210 (single-arm atezolizumab trial in first-line setting) [37] and IMvigor211 (phase III randomized trial of atezolizumab vs. chemotherapy in first-line setting) [38,39]. Interestingly, no association between PPI use and survival outcomes were found in the participants who received chemotherapy in IMvigor211 [39]. The detrimental effect of concomitant PPIs was further reported in a multicentre study conducted on 135 patients affected by mUC and treated with pembrolizumab or paclitaxel-gemcitabine after platinum-based chemotherapy [40].
In this study, PPIs usage was associated with significantly reduced PFS and OS in patients receiving pembrolizumab, although this effect was not observed in patients treated with chemotherapy, even when corrected for possible confounding factors in a multivariate analysis [40]. Of note, the negative impact of PPIs on ICIs efficacy seems to be influenced by patients’ characteristics: in particular, it was observed that survival outcomes were significantly decreased in younger and male patients [43].
Furthermore, other studies have evaluated the association between PPIs and Abs on survival outcomes in mUC patients treated with immunotherapy [41,47]. In a retrospective study, the objective response rate (ORR) was significantly reduced in patients using both PPIs and Abs (ORR = 12%, p = 0.004) or either one of them (ORR = 33%, p = 0.010). PFS after ICI therapy was significantly reduced in the double users (median 3.0 months) than in the non-users (median 37.0 months, p < 0.001) or single users (median 5.8 months, p = 0.035). OS after ICI therapy was significantly shorter in the double users (median 6.5 months) than in the non-users (median 50 months, p < 0.001) or single users (median 15 months, p = 0.015) [41]. Similar results were described in a Japanese retrospective study [47].
Furthermore, when compared with different medications used in chronic diseases (e.g., statins, metformin) PPIs were found to be the only concomitant therapy that negatively affected survival outcomes [7,46].
Interestingly, Sekito et al. compared histamine-2 receptor antagonists (H2RAs) with PPIs in a retrospective multicentre study including 404 patients. The authors observed that the use of PPIs was a negative prognostic factor for both OS (HR = 1.42, 95% CI 1.08–1.87, p = 0.011) and PFS (HR = 1.35, 95% CI 1.05–1.73, p = 0.020), while H2RAs was not associated with survival or ORRs, thus representing a valid alternative during ICIs administration [42].
Ultimately, the detrimental effect of PPIs on survival outcomes was confirmed in multiple meta-analyses comprising different solid tumors, including advanced UC [12,48–50]. Among these metanalyses, it is worth to mention that Zhang et al. [48] and Rizzo et al. [14] have taken into account only patients with mUC. Zhang et al. analysed data from six studies involving 1980 patients with advanced UC and found that concomitant PPIs were associated with an increased risk of progression and death of 50.7% (HR: 1.507, 95% CI: 1.327–1.711, p < 0.001) and 58.7% (HR: 1.587, 95% CI: 1.367–1.842, p < 0.001), respectively [48]. Furthermore, ORR was significantly reduced in mUC patients treated ICIs and PPIs (OR: 0.503, 95% CI: 0.360–0.703, p < 0.001). Rizzo et al. found similar results in their meta-analysis, which included two studies encompassing a total of 1015 mUC patients [14]. Both meta-analyses showed a low level of heterogeneity (I2 = 7.4% and I2 = 0.0% for PFS, I2 = 37.4% and I2 = 0.0% for OS, I2 = 47.0% for ORR, respectively). Other metanalyses also provide an interesting comparison with other solid tumors: Lopes et al. observed that PPIs would negatively affect OS and PFS in patients with advanced NSCLC receinving ICIs [12]. On the other hand, Chang et al.’s work showed limited or no detrimental effect on survival outcomes in advanced renal cell carcinoma (RCC), hepatocellular carcinoma (HCC), head and neck squamous cells carcinomas and melanoma, during concomitant immunotherapy [13].
Role of antibiotics in modulating immune response
Abs are one of the most commonly used drugs in oncological patients; especially, those affected by UC have a relatively high risk of urinary tract infections (UTI) due to urinary diversion [17]. Abs are known to have several adverse effects, including enterocolitis caused by damage to commensal gut microbiota, which plays an important role in regulating homeostasis and immune function, finally leading to a negative impact on systemic immune response [17,20]. At the same time, cancer can alter the composition of gut bacteria, which plays a role in regulating the TME and promoting immune suppression. As a result, cancer cells and self-reactive immune cells may potentially cross-react with bacterial populations [51]. Notably, the gut microbiota has gained recognition as a crucial factor influencing cancer treatment outcomes and is thought to contribute to the variability observed in patient responses to immunotherapy, especially ICIs [52]. One study reported that mice with a disruption of the microbiota exhibited inferior immune-mediated responses to medical therapies, suggesting that an intact microbiota is essential for an optimal response to cancer therapies, including chemotherapy and immunotherapy [53]. Moreover, fecal microbiota transplant (FMT) from responders (R-FMT) and nonresponders (NR-FMT) into germ-free (GF) mice confirmed the microbial modulation of antitumor immune responses. Compared with R-FMT mice, NR-FMT mice had more rapid tumor growth and poorer responses to anti-PD-1 therapy, which indicated that the gut microbiota could be a modulator of the responses to ICIs [54,55]. Abs use may impact the patient’s microbiota composition for a long time [56].
Thus, some researchers hypothesize that dysbiosis of the gut microbiota caused by Abs may be associated with ICIs resistance and have a negative impact on ICIs efficacy [57]. Several studies have demonstrated differences in the stool microbial composition in responders and non-responders to ICIs for lung, renal and melanoma patients [19]. However, the favorable gut microbiota composition and diversity that produces the most optimal response to ICI is yet to be elucidated [19]. There were some suggestions from metagenomic studies that high diversity and differential abundance of beneficial bacterial taxa in the gut, such as Ruminococcaceae, Akkermansia, Bifidobacterium, Bacteroides, Faecalibacterium, and Akkermansia muciniphilla may be closely linked to positive response to ICIs [18]. Their advantage arises from an active and dynamic interaction with the immune system, promoting enhanced dendritic cell maturation, improved priming, and increased accumulation of effector T-cells within the tumor microenvironment [52].
Some Abs may also have a direct impact on systemic inflammation and immune response: quinolones can lower the levels of pro-inflammatory cytokines, and macrolides can reduce the T cell response, resulting in a potential negative effect on response to ICIs [57]. These findings have led to a paradigm shift, with the gut microbiome now being regarded as a significant predictive biomarker for treatment response and a potential therapeutic target to enhance the effectiveness of immunotherapies. However, it is important to acknowledge that some studies have found no link between antibiotic use and response to ICIs, and findings from observational cohorts may be influenced by the overall health condition of patients requiring Abs use. In fact, patients who require Abs are more likely to have multiple comorbidities and moderate to severe infections [17,18]. These patients likely experience treatment interruption, with a potential impact on disease progression and poorer survival [18]. Ultimately, timing also represents a crucial element on the impact of Abs use on ICIs outcomes. In fact, the period including the month prior and the month following the start of ICIs, appeared to be the most vulnerable to Abs use, potentially for the impact of dysbiosis on the immune “priming” response [34].
With such assumptions, it becomes crucial to understand if the effects of Abs on the efficacy of ICIs is clinically relevant [17].
Prognostic impact of antibiotics use in influencing survival outcomes in patients with metastatic urothelial carcinoma treated with immunotherapy
Several meta-analyses demonstrated that administration of Abs either before or during treatment with ICIs, was associated with shorter PFS and OS in multiple solid tumors, including NSCLC [57], RCC [57], melanoma [57] and UC [17,18,20]. Also ORR resulted significantly reduced in patients treated with ICIs and concomitant Abs [21,57,58]. Furthermore, the detrimental effect on survival outcomes was independent from the type of cancer and the type of ICI used [21,57–59].
Patients with advanced NSCLC are particularly vulnerable to receive Abs during the course of their disease or in the months leading up to their cancer diagnosis, due to the median advanced age and the smoking status [60]. A meta-analysis of 23 studies on patients with NSCLC receiving ICIs, described a detrimental effect of concomitant Abs use in terms of OS (HR 1.69, 95%CI 1.25–2.29) and PFS (HR 1.47, 95%CI 1.13–1.90) [60]. Based on this evidence, it can be hypothesized that Abs negatively affect ICI efficacy and likely contribute to the development of resistance to anti-PD-1 antibodies. However, additional research is necessary to establish a definitive causal link between antibiotic use and resistance to ICI therapy.
Due to the high risk of urinary tract and upper respiratory infections, Abs are also frequently used in RCC patients. Luo et al. demonstrated that Abs exposure was significantly associated with worse PFS and OS in RCC patients receiving ICIs; however, no significant link was found between concomitant Abs use and increased risk of disease progression [61]. In contrast, a retrospective study involving 749 patients with melanoma who received Abs compared to 1856 non-exposed patients suggested that Abs use prior to anti-PD-1 treatment was not linked to poorer outcomes, either in terms of OS or time to treatment discontinuation [62]. Different studies have evaluated the prognostic role of Abs administration specifically in patients with mUC, Table 2.
Febriyanto et al. conducted a metanalysis on thirteen non-randomized studies, including a total of 5095 patients with mUC treated with ICIs, of which 1434 (28%) received Abs. The pooled HRs for OS and PFS in those who received Abs were 1.45 [95% CI 1.25−1.68] and 1.40 [95% CI 1.05−1.87], respectively, compared to those who did not receive Abs [18]. Again, the type of ICI used did not influence the effect of Abs on OS and PFS [18].
The optimal time window for Abs use remains a critical point to explore, in fact the time of Abs exposure might impact ICIs effectiveness [18,21,65]. The time it takes to restore the gut microbiota composition and mount an effective antitumoral immune response following antibiotic use remains unclear [18]. Abs appear to influence ICIs outcomes even after their withdrawal, with a possible deleterious effect over a long period [65]. Especially, Abs use in the 42 days before starting ICIs appears to have the most detrimental impact on outcome [17]. A previous study by Pinato et al. showed that Abs treatment administered within 30 days prior to ICI therapy was associated with significantly worse OS, but not with concurrent ICI therapy [66]. Of note, this was the only prospective study reported in literature, included 196 patients with different tumor types [66]. In another study by Khan et al., the maximal negative impact on the effectiveness of ICIs occurred when Abs were used in the first six weeks after initiating ICI [64]. Even the metanalyses by Huang et al. [67] and Yu et al. [21] showed an impact on the efficacy of ICIs within before or after two months, while a better PFS and OS was demonstrated concurrently with ICIs therapy [57].
The type of Abs used seems not to influence survival outcomes, although the use of broad-spectrum Abs was strongly associated with poor PFS [59].
Some retrospective studies have also studied the impact of Abs specifically on patients with mUC receiving ICIs. In 2020, Hopkins et al. performed a post-hoc analysis of IMvigor210 and IMvigor211 to study the association between Abs use within 30 days before and after ICIs initiation, and survival [20]. Interestingly, Abs use was associated with worse PFS and OS in patients treated with atezolizumab, but not with chemotherapy [20]. In 2021, Ishiyama et al. confirmed these findings in a cohort of 67 patients treated with pembrolizumab [17]. Of note, patients who were administrated Abs were also less likely to achieve response or disease control [17]. Accordingly, Agarwal et al. showed that concurrent Abs can influence outcomes and response in patients with mUC receiving both anti-PD1 and anti-PD-L1 agents [63].
Conversely, Khan et al. involved 130 patients with mUC treated with different type of ICIs. This was the only study showing that Abs use within 60 days before or after ICI initiation did not significantly impact OS and PFS [68].
Role of antihypertensive drugs in modulating immune response
Pre-clinical evidence highlighted a possible role of the RAS as a pro-inflammatory modulator in the TME [69–71]. Specifically, the RAS was associated with an enhanced immunosuppressive environment through upregulation of PD-L1 expression and the presence of immunoregulatory cells such as tumor-associated macrophages, myeloid-derived suppressor cells, and cancer-associated fibroblasts [72,73]. From a biological perspective, pre-clinical studies suggested that the inhibition of the RAS may down regulate transforming growth factor (TGF)-β, a transcription factor, mainly active in TME stromal and fibroblasts cells. TGF-β is a component of multiple signalling pathways, regulating proliferation, apoptosis, invasion, migration, immunosuppression, chemo-resistance and progression [74]. Additional evidence underline RAS ability of promoting angiogenesis via vascular endothelial growth factor (VEGF) synthesis [75]. The RAS inhibition may consequently have anti-fibrotic effects, decrease stromal collagen I, impact tumor perfusion and drug delivery [69,76]. Accordingly, various evidence proposed that a TGF-β increase was related to resistance to ICI [77], thus, inhibiting the RAS may enhance ICI responses [78]. Furthermore, pre-clinical evidence proposed a possible role of RAS inhibition in inducing a pro-inflammatory TME [69–71,79]. In details, RAS appears to enhance the TME PD-L1 expression, the immunosuppressive phenotype of tumor associated macrophages (TAMs) and myeloid-deprived suppressive cells (MDSCs), mainly through the production of immunosuppressive chemokine [72,73,79]. This hypothesis was also supported by evidence that ARBs could reverse ICI resistance in mouse models [79]. Nevertheless, the data remain controversial.
β-blockers are another widely used class of antihypertensive drugs, whose activity appears to be related to an immunosuppressive TME. Specifically, β-adrenergic receptors, which are present on lymphoid organs and immune cells [80], can down regulate T-cell proliferation and cytotoxicity, and stimulate immunosuppressive regulatory T cells [81]. The inhibition of β-adrenergic receptors enhanced CD8+ T cells recruitment and activation and PD-1 expression [82]. Additionally, pre-clinical data suggested a role of β-blockers in inhibiting tumor growth, when used in combination with ICIs [82,83].
Do antihypertensive drugs impact on ICIs effectiveness?
A retrospective study on patients affected by solid tumors receiving ICIs, including bladder, ovarian and prostate cancer, described improvements in terms of ORR, CR rate and OS, in those with concomitant ACEIs or ARBs [84]. Similarly, an observational analysis on advanced RCC correlated longer OS with RAS inhibitors use [85]. In contrast, a retrospective analysis identified a direct correlation between the use of ACE inhibitors and poorer ICI outcomes in patients with NSCLC [86]. Ultimately, retrospective evidence on patients with NSCLC [87] or on a larger cohort of patients with solid tumors (NSCLC, RCC or UC) receiving ICIs, highlighted no correlation between outcomes and RAS inhibitors use [26].
Modest data are available for patients with mUC. A retrospective analysis of 279 patients with UC treated with ICIs and concomitant ARBs or ACE inhibitors found a positive association with OS (Table 2) [22]. Additionally, a pooled analysis of seven studies was conducted, including 2539 patients receiving atezolizumab and concomitant antihypertensive treatments (RAS inhibitors, ACE inhibitors, ARBs, direct renin inhibitors, and beta blockers), about 35% of whom affected by UC (n = 888) [26]. This analysis noted no association between the use of anti-hypertensives and oncological outcomes in terms of OS and PFS [26]. The only statistically significant finding was a worse PFS associated with the concomitant use of ARBs (adjusted HR 1.16, 95% CI 1.01–1.33, p = 0.038) [26]. In order to further investigate the impact of RAS inhibitors on ICI outcomes, a meta-analysis of twelve retrospective or integrated post hoc studies was led. This meta-analysis included a total of 11,739 patients with UC, RCC, melanoma, and NSCLC, with 12%–58% of them receiving concomitant RAS inhibitors [24]. Significant heterogeneity was observed among the studies (I2 = 52.1%, p = 0.010 for OS and I2 = 61.0%, p = 0.012 for PFS). The metanalysis found a significantly better OS [pooled HR 0.85 (95% CI, 0.75–0.96; p = 0.009)] and a trend toward better PFS [pooled HR 0.91 (95% CI, 0.76–1.09; p = 0.296)] in RAS inhibitors users [24]. Furthermore, the subgroup analysis of UC patients confirmed a statistically significant benefit for OS (HR 0.53; 95% CI, 0.31–0.89; p = 0.018) but not for PFS [24]. The association was found only with the simultaneous use of antihypertensives, with no effect reported on the incidence of irAEs [24].
Regarding the impact of concomitant β-blockers use on ICI outcomes, the evidence remains controversial. Two similar observational studies on melanoma and NSCLC, suggested a potential survival benefit in patients treated with ICIs and concomitant β-blockers [83,88]. However this evidence was not confirmed from other retrospective data. For instance, a detrimental effect of concomitant β-blockers use in NSCLC patients receiving ICIs, was described [87]. Another a retrospective analysis was conducted involving 339 patients with solid tumors (melanoma, NSCLC, hepatocellular carcinoma, and UC) who were receiving ICIs [23]. This analysis compared patients treated with β-blockers (n = 109%, 32%) to those without concurrent β-blocker treatment. The study found a positive correlation between concurrent β-blockers and disease control rate (62% vs. 39%, odds ratio (OR) 2.79, p < 0.001) in the overall population, and with OS in UC patients (HR 0.24, p = 0.003) [23]. Additionally, a phase I trial testing the association of pembrolizumab and propranolol, a β-blocker, showed promising results, with ORR of 78% in patients affected by melanoma [89]. Ultimately, a systematic review and metanalysis of nine studies was conducted. This analysis evaluated 1364 patients with melanoma, NSCLC, RCC, and UC. No significant association between β-blockers use and either OS or PFS was found [pooled HR 0.99 for OS and 0.97 for PFS] [25].
Many other drugs are being studied for their potential role when used in combination with immunotherapy treatments.
The negative impact of corticosteroid use on ICIs responses is well-known [90] and was confirmed in a retrospective analysis of 8870 patients, 47.8% of whom were treated with concomitant steroids. In the subgroup of patients with UC (10.8%), corticosteroid use was associated with worse early progressive disease (OR 1.49 (1.11–2.01), p = 0.01) and OS (1.43 (1.19–1.72) p < 0.0001) [28]. Data suggest that steroid use before or shortly after ICI initiation is associated with poorer clinical outcomes [8,9,28,90].
The role of statins and metformin, two common concomitant drugs, has recently been explored in patients treated with ICIs [7].
In vitro evidence has shown that statins can inhibit tumor cell growth and invasion [91,92]. Specifically, the inhibition of 3-hydroxy-3-methylglutaryl coenzyme A (HMG-CoA) reductase, and the following depletion of isoprenoids, appear to prevent the G1 to S phase transition [93]. Furthermore, statins might induce cell apoptosis through the regulation of pro-apoptotic and anti-apoptotic factors [93]. Ultimately, statins were able to inhibit the metastatic ability of breast cancer cell [94]. A meta-analysis of 27 studies, including 163,005 patients with advanced-stage solid tumors, mainly lung, pancreatic and ovarian cancers, described longer OS (HR 0.74), CSS (HR 0.74) and PFS (HR 0.76), in concomitant statins users [95]. In relation to ICIs, statins have demonstrated a role in regulating T-cell activity and migration, antigen presentation, and cytokine production [96]. A retrospective study of 1510 patients with non-muscle invasive bladder cancer highlighted a potential impact of statins in reducing recurrence rates [97]. Furthermore, retrospective evidence of 2602 patients with NMIBC receiving intravesical bacille Calmette-Guérin described longer OS and CSS, with concomitant statins use [98]. A retrospective analysis of 219 patients with RCC receiving nivolumab showed longer OS and PFS in statin users (p = 0.017 and p = 0.013, respectively) [92]; accordingly, some evidence proposed a potential adjuvant benefit of concomitant statins, in terms of OS, in patients with HCC [99], NSCLC [100]. Statins were also related to better ORR, longer PFS and OS, in a retrospective cohort of patients with thoracic cancer (82 with malignant pleural mesothelioma and 179 with NSCLC) treated with PD-1 inhibitors [96]. However, this benefit was not observed in a population of patients with UC treated with ICIs [7,101].
A potential role of metformin in controlling cancer cell growth, both direct and indirect, and enhancing ICI responses has also been described [91]. Metformin may directly inhibit pathway related to cancer invasion and migration, including AMP-activated protein kinase (AMPK), epithelial-mesenchymal transition (EMT), VEGF and mammalian target of rapamycin (mTOR) [102], thus inhibiting cancer cell progression [103]. Moreover, its anti-inflammatory and hypoglycemic effects, represent protective factors against cancer development [104]. On a biological level, metformin might enhance natural killer (NK) and cytotoxic T cells, and decrease regolatory T cells and myeloid-derived suppressor cells (MDSCs) [105]. Intriguingly, pre-clinical evidence suggest metformin might inhibit both PD-1 and PD-L1, in the TIME, increasing ICIs responses [106,107]. However, the evidence remains controversial. Some observational studies on cohorts of patients with solid cancer suggested a potential benefit of concomitant ICIs and metformin, in terms of ORR, PFS and OS [91,108,109], whereas various other retrospective analyses did not confirm this evidence, neither in a cohort of metastatic RCC [110], nor in melanoma patients treated in adjuvant setting [111]. Conversely, metformin use appeared to be detrimental in a cohort of HCC patients, receiving atezolizumab and bevacizumab [112]. Regarding UC, in the real-world ARON-2 study, data from 802 patients with UC treated with ICIs were collected. The concomitant use of metformin (12% of patients) did not show any influence on ICI outcomes (PFS: 7.1 (95%CI 3.7–12.0) vs. 6.2 (95%CI 5.0–6.9) months, p = 0.630; OS: 12.4 (95%CI 7.8–16.0) vs. 10.5 (95%CI 9.0–13.3) months, p = 0.896) [7].
Recent data suggest a negative impact of acetaminophen on oncological response to ICIs. Specifically, pre-clinical studies have shown inhibition of immune cell proliferation and activity [113], as well as a decrease in interferon-induced responses [27]; this evidence is supported by recent data showing reduced vaccination response in patients receiving acetaminophen [114]. A retrospective analysis of the phase III trial CheckMate 025, which investigated nivolumab in patients with advanced RCC, noted that detectable levels of acetaminophen in the plasma were associated with significantly worse OS [27]. In the same study, this tendency was further confirmed in a broader population of patients with solid tumors, including those with UC (5%), receiving ICIs. Detectable levels of acetaminophen were again associated with worse OS (p < 0.0001) and PFS (p = 0.009) [27].
Opioids also appear to have a role in modulating ICIs response, worsening ICIs outcomes [115]. The biological rationale may lay in their immune suppression effect through T-cell modulation [116] and NK inhibition [117]. A real-world analysis of 8870 patients receiving ICIs, 10.8% of whom were affected by UC, highlighted the negative impact of opioids on both early progressive disease (OR 2.80; 95%CI 2.07–3.83) and OS (HR 1.68 (1.39–2.03) p < 0.0001) [28].
Searching for clinical and biological biomarkers that can predict the response to ICIs is an open issue in the oncology field. Even more challenging is identifying simple and routine factors that can be used in daily clinical practice. Patients with mUC frequently have comorbidities or disease- and therapy-related symptoms that require pharmacological intervention. Concomitant drugs are often prescribed without considering their potential influence on the effectiveness of ICIs, although recent evidence has shown that commonly used drugs may affect oncological outcomes. Hence, the influence of concomitant drugs on immunotherapy has become an area of emerging interest. Among commonly prescribed drugs, corticosteroids, PPIs, Abs, antihypertensives, and analgesics have been shown to impact the prognosis of patients treated with ICIs.
In this review, we have analyzed the impact of the aforementioned medications on the survival of patients with cancer treated with ICIs, particularly mUC. The negative impact on PFS and OS of concomitant corticosteroids, PPIs and Abs has been widely demonstrated by different retrospective studies, and confirmed by several metanalyses. Of note, some post-hoc analyses of prospective studies strengthened this evidence for PPIs and Abs, although only regarding patients receiving atezolizumab, which is no more recommended by regulatory agencies in this setting [20,39]. Interestingly, as already mentioned some studies reported a differential impact of concomitant PPIs and Abs between ICIs and chemotherapy, suggesting a possible predictive role [20,39,40].
The mechanism behind this effect can be mediated by the influence of PPIs and Abs on the gut microbiota. Data suggest that gut microbes may impact antitumor immunity via several mechanisms, including interaction of microbial components or products with antigen-presenting cells (APCs) and innate effectors such as Toll-like receptors, which help prime an adaptive immune response; induction of cytokine production by APCs or lymphocytes; and local or distant effects of microbial metabolites [40]. PPIs and Abs can influence the composition of the gut microbiota promoting the growth of typically oral bacteria, diminishing gut microbiota diversity and impairing the immune environment [15,16,30,57]. Recently, additional interest is emerging on intratumoral microbiota, as shown by evidence linking intratumoral Escherichia with improved survival in NSCLC patients treated with single-agent ICIs [118].
In light of this, modulation of gut and intratumoral microbiota could be an area of promising research. Intriguingly, clinical studies have reported that fecal microbiota transplantation using stool collected from ICIs responders, allows patients to overcome resistance to ICIs [32,41,44,46]. However, it is relevant to take into account that several mechanisms can alter the gut microbiota, including irAEs, such as diarrhoea, which have been cited as a positive predictor of survival in patients undergoing ICIs [17].
Differently from PPIs and Abs, ACEIs and ARBs, as well as beta-blockers, have shown to improve survival outcomes of patients with mUC undergoing ICIs, while the effect of concomitant administration of other drugs, such as statins and metformin, is not yet fully understood. Both of them can interact with the immune system, although their impact on oncological outcomes remains controversial.
Finally, analgesic drugs are frequently used in oncological patients. Interestingly, acetaminophen and opioids have been shown to reduce the immune response, thus worsening the survival of patients with solid tumors treated with ICIs. However, patients receiving analgesics for major pain might be in worse condition than other patients, thus influencing the prognosis. It is worth noting that the studies we reported are limited by possible confounding factors, among the others, the different populations, type of ICI used, and polypharmacotherapy.
Moreover, we described the impact on ICIs outcomes for each class of drugs separately. In this light, some authors have evaluated the prognostic role of polytherapy (PPIs, Abs and corticosteroids), developing and validating a “drug score” in patients with NSCLC treated with ICIs [119]. Recently, this score has been validated on a cohort of patients with mUC treated with ICIs, demonstrating that a higher number of concomitant drugs was associated with worse response and survival [47].
The mechanisms underlying the interaction between concomitant drugs and immunotherapy remain hypothetical and warrant further validation with preclinical studies and possibly with prospective trials. In recent years, considerable efforts have been made in this direction.
With respect to the possible influence of PPIs and Abs on the gut microbiota and the efficacy of immunotherapy, various mouse models have provided insights. These studies demonstrated that the efficacy of anti-CTLA4 was initially suppressed by Abs but subsequently restored through fecal transplantation or by directly feeding mice with Bacteroides isolates or probiotics [54,120].
This approach was later applied in several human studies. In the TACITO trial, patients with mRCC receiving immunotherapy combinations were randomized to receive either placebo or a fecal transplant from an immunotherapy responder [121]. In other trials, patients undergoing nivolumab and ipilimumab or cabozantinib treatment for mRCC were randomized to receive either CBM or placebo, with significant improvements in oncological outcomes [122,123].
A different approach has been used by Medik et al., who reported favorable outcomes in mice treated with anti-CTLA-4 ICI and metronidazole [124]. Using antibiotics to modulate immune responses through microbiota alterations could be a promising strategy for enhancing ICI efficacy [125]: a phase II trial led by Monge et al. is currently assessing the combination of nivolumab, oral vancomycin, and tadalafil in hepatocellular carcinoma (HCC) and liver-dominant metastatic digestive cancers (NCT03785210) [126].
Many efforts have been made also to understand the interaction between other compounds and immunotherapy. Metformin have shown to lead to the degradation of membrane PD-L1 in breast tumor mouse models as well as in patient samples, and to enhance PD-1 inhibitor efficacy in lung cancer [127,128]. Phase I and II trials are currently assessing the potential of metformin in combination with anti-PD-1 and anti-PD-L1 therapies for various cancers, including melanoma (NCT04114136), NSCLC (NCT03048500), and colorectal cancer (NCT03800602) [129,130].
A phase I study by Gandhi et al. established a dosing regimen of propranolol with pembrolizumab in melanoma patients, demonstrating satisfactory safety [89]. Ongoing phase II trials are further exploring the positive effects of beta-blockers in cancers such as triple-negative breast cancer (NCT05741164), UC (NCT04848519), and melanoma (NCT03384836, NCT05968690).
In another study, Chauhan et al. developed a tumor-selective ARB, which enhanced ICI efficacy in animal models without lowering blood pressure [74]. However, prospective clinical studies are needed to confirm these findings and establish clinical benefits.
Hopefully, the ongoing trials will expand our understanding of this pivotal field and provide some guidance for physicians’ choices in everyday practice.
A key limitation of our work is that it is not a systematic review, meaning that only the most relevant studies were included, rather than considering all available evidence. Additionally, the majority of the data analyzed are from retrospective studies, which are subject to inherent biases like selection bias, confounding factors, and incomplete information. These issues restrict the ability to draw firm conclusions regarding causality. The heterogeneity across studies, with differences in methodologies, patient populations, and treatment protocols, further complicates the consistent interpretation of results and their application to specific clinical scenarios. Moreover, the lack of randomized controlled trials (RCTs) specifically designed to assess the impact of concomitant drugs on ICI outcomes weakens the overall strength of the findings, as RCTs are considered the gold standard for establishing a cause-effect relationship. Lastly, unmeasured confounding factors, such as patient comorbidities and disease severity, may account for some of the observed associations, as these factors were not uniformly reported in the studies analyzed.
In conclusion, considering all the evidences available regarding the prognostic significance of concomitant drugs in patients receiving immunotherapy, it could be state that medications should be prescribed with caution and only when clinically necessary, especially before the initiation of ICI therapy.
Acknowledgement: None.
Funding Statement: The authors received no specific funding for this study.
Author Contributions: The authors confirm contribution to the paper as follows: study conception and design: Michele Maffezzoli, Giulia Claire Giudice, Sebastiano Buti; data collection: Michele Maffezzoli, Giulia Claire Giudice, Giacomo Iovane, Martina Manini, Elena Rapacchi, Nicola Simoni, Davide Campobasso; analysis and interpretation of results: Michele Maffezzoli, Giulia Claire Giudice, Giuseppe Caruso, Stefania Ferretti, Sebastiano Buti; draft manuscript preparation: Michele Maffezzoli, Giulia Claire Giudice, Giacomo Iovane, Martina Manini, Elena Rapacchi, Stefano Puliatti, Giuseppe Caruso, Nicola Simoni, Sebastiano Buti. All authors reviewed the results and approved the final version of the manuscript.
Availability of Data and Materials: Data sharing not applicable to this article as no datasets were generated or analyzed during the current study.
Ethics Approval: Not applicable.
Conflicts of Interest: The authors declare no conflicts of interest to report regarding the present study.
References
1. Taguchi S, Kawai T, Nakagawa T, Miyakawa J, Kishitani K, Sugimoto K, et al. Improved survival in real-world patients with advanced urothelial carcinoma: a multicenter propensity score-matched cohort study comparing a period before the introduction of pembrolizumab (2003–2011) and a more recent period (2016–2020). Int J Urol. 2022;29(12):1462–9. doi:10.1111/iju.v29.12. [Google Scholar] [CrossRef]
2. Balar AV, Castellano DE, Grivas P, Vaughn DJ, Powles T, Vuky J, et al. Efficacy and safety of pembrolizumab in metastatic urothelial carcinoma: results from KEYNOTE-045 and KEYNOTE-052 after up to 5 years of follow-up. Ann Oncol. 2023;34(3):289–99. doi:10.1016/j.annonc.2022.11.012. [Google Scholar] [PubMed] [CrossRef]
3. Powles T, Park SH, Caserta C, Valderrama BP, Gurney H, Ullén A, et al. Avelumab first-line maintenance for advanced urothelial carcinoma: results from the JAVELIN bladder 100 trial after ≥2 years of follow-up. J Clin Oncol. 2023;41(19):3486–92. doi:10.1200/JCO.22.01792. [Google Scholar] [PubMed] [CrossRef]
4. Van der Heijden MS, Sonpavde G, Powles T, Necchi A, Burotto M, Schenker M, et al. Nivolumab plus gemcitabine-cisplatin in advanced urothelial carcinoma. New Engl J Med. 2023;389(19):1778–89. doi:10.1056/NEJMoa2309863. [Google Scholar] [PubMed] [CrossRef]
5. Powles T, Valderrama BP, Gupta S, Bedke J, Kikuchi E, Hoffman-Censits J, et al. Enfortumab vedotin and pembrolizumab in untreated advanced urothelial cancer. New Engl J Med. 2024;390(10):875–88. doi:10.1056/NEJMoa2312117. [Google Scholar] [PubMed] [CrossRef]
6. Hopkins AM, Rowland A, Kichenadasse G, Wiese MD, Gurney H, McKinnon RA, et al. Predicting response and toxicity to immune checkpoint inhibitors using routinely available blood and clinical markers. Br J Cancer. 2017;117(7):913–20. doi:10.1038/bjc.2017.274. [Google Scholar] [PubMed] [CrossRef]
7. Fiala O, Buti S, Takeshita H, Okada Y, Massari F, Palacios GA, et al. Use of concomitant proton pump inhibitors, statins or metformin in patients treated with pembrolizumab for metastatic urothelial carcinoma: data from the ARON-2 retrospective study. Cancer Immunol, Immunother. 2023;72(11):3665–82. doi:10.1007/s00262-023-03518-z. [Google Scholar] [PubMed] [CrossRef]
8. Goodman RS, Johnson DB, Balko JM. Corticosteroids and cancer immunotherapy. Clin Cancer Res. 2023;29(14):2580–7. doi:10.1158/1078-0432.CCR-22-3181. [Google Scholar] [PubMed] [CrossRef]
9. Buti S, Bersanelli M, Perrone F, TIseo M, Tucci M, Adamo V, et al. Effect of concomitant medications with immune-modulatory properties on the outcomes of patients with advanced cancer treated with immune checkpoint inhibitors: development and validation of a novel prognostic index. Eur J Cancer. 2021;142:18–28. doi:10.1016/j.ejca.2020.09.033. [Google Scholar] [PubMed] [CrossRef]
10. Liu C, Guo H, Mao H, Tong J, Yang M, Yan X, et al. An up-to-date investigation into the correlation between proton pump inhibitor use and the clinical efficacy of immune checkpoint inhibitors in advanced solid cancers: a systematic review and meta-analysis. Front Oncol. 2022;12:753234. doi:10.3389/fonc.2022.753234. [Google Scholar] [PubMed] [CrossRef]
11. Chen B, Yang C, Dragomir MP, Chi D, Chen W, Horst D, et al. Association of proton pump inhibitor use with survival outcomes in cancer patients treated with immune checkpoint inhibitors: a systematic review and meta-analysis. Ther Adv Med Oncol. 2022;14:17588359221111703. doi:10.1177/17588359221111703. [Google Scholar] [PubMed] [CrossRef]
12. Lopes S, Pabst L, Dory A, Klotz M, Gourieux B, Michel B, et al. Do proton pump inhibitors alter the response to immune checkpoint inhibitors in cancer patients? A meta-analysis. Front Immunol. 2023;14:1070076. doi:10.3389/fimmu.2023.1070076. [Google Scholar] [PubMed] [CrossRef]
13. Chang Y, Lin WY, Chang YC, Huang C-H, Tzeng HE, Abdul-Lattif E, et al. The association between baseline proton pump inhibitors, immune checkpoint inhibitors, and chemotherapy: a systematic review with network meta-analysis. Cancers. 2023;15(1):284. doi:10.3390/cancers15010284. [Google Scholar] [PubMed] [CrossRef]
14. Rizzo A, Santoni M, Mollica V, Ricci A-D, Calabrò C, Cusmai A, et al. The impact of concomitant proton pump inhibitors on immunotherapy efficacy among patients with urothelial carcinoma: a meta-analysis. J Pers Med. 2022;12(5):842. doi:10.3390/jpm12050842. [Google Scholar] [PubMed] [CrossRef]
15. Jackson MA, Goodrich JK, Maxan M-E, Freedber DE, Abrams JA, Poole AC, et al. Proton pump inhibitors alter the composition of the gut microbiota. Gut. 2016;65(5):749–56. doi:10.1136/gutjnl-2015-310861. [Google Scholar] [PubMed] [CrossRef]
16. Vich Vila A, Collij V, Sanna S, Sinha T, Imhann F, Bourgonje AR, et al. Impact of commonly used drugs on the composition and metabolic function of the gut microbiota. Nat Commun. 2020;11(1):362. doi:10.1038/s41467-019-14177-z. [Google Scholar] [PubMed] [CrossRef]
17. Ishiyama Y, Kondo T, Nemoto Y, Kobari Y, Ishihara H, Tachibana H, et al. Antibiotic use and survival of patients receiving pembrolizumab for chemotherapy-resistant metastatic urothelial carcinoma. Urol Oncol: Semin Original Investig. 2021;39(12):834.e21–8. doi:10.1016/j.urolonc.2021.05.033. [Google Scholar] [PubMed] [CrossRef]
18. Febriyanto T, Muhammad F, Wijaya W, Oey O, Simadibrata EM. Antibiotic use reduces the efficacy of immune checkpoint inhibitors in patients with urothelial carcinoma: a systematic review and meta-analysis. Urol Oncol: Semin Original Investig. 2024;42(5):160.e11–23. doi:10.1016/j.urolonc.2023.11.017. [Google Scholar] [PubMed] [CrossRef]
19. Wilson BE, Routy B, Nagrial A, Chin VT. The effect of antibiotics on clinical outcomes in immune-checkpoint blockade: a systematic review and meta-analysis of observational studies. Cancer Immunol Immunother. 2020;69(3):343–54. doi:10.1007/s00262-019-02453-2. [Google Scholar] [PubMed] [CrossRef]
20. Hopkins AM, Kichenadasse G, Karapetis CS, Rowland A, Sorich MJ. Concomitant antibiotic use and survival in urothelial carcinoma treated with atezolizumab. Eur Urol. 2020;78(4):540–3. doi:10.1016/j.eururo.2020.06.061. [Google Scholar] [PubMed] [CrossRef]
21. Yu Y, Zheng P, Gao L, Li H, Tao P, Wang D, et al. Effects of antibiotic use on outcomes in cancer patients treated using immune checkpoint inhibitors: a systematic review and meta-analysis. J Immunother. 2021;44(2):76–85. doi:10.1097/CJI.0000000000000346. [Google Scholar] [PubMed] [CrossRef]
22. Jain RK, Skelton IVWP, Pond GR, Naqvi M, Kim Y, Curran C, et al. Angiotensin blockade modulates the activity of PD1/L1 inhibitors in metastatic urothelial carcinoma. Clin Genitourin Cancer. 2021;19(6):540–6. doi:10.1016/j.clgc.2021.04.002. [Google Scholar] [PubMed] [CrossRef]
23. Mellgard G, Patel VG, Zhong X, Joshi H, Qin Q, Wang B, et al. Effect of concurrent beta-blocker use in patients receiving immune checkpoint inhibitors for advanced solid tumors. J Cancer Res Clin Oncol. 2023;149(7):2833–41. doi:10.1007/s00432-022-04159-y. [Google Scholar] [PubMed] [CrossRef]
24. Shen J, Hou H, Liang B, Guo X, Chen L, Yang Y, et al. Effect of renin-angiotensin-aldosterone system inhibitors on survival outcomes in cancer patients treated with immune checkpoint inhibitors: a systematic review and meta-analysis. Front Immunol. 2023;14:1155104. doi:10.3389/fimmu.2023.1155104. [Google Scholar] [PubMed] [CrossRef]
25. Kennedy OJ, Neary MT. Brief communication on the impact of β-blockers on outcomes in patients receiving cancer immunotherapy. J Immunother. 2022;45(7):303–6. doi:10.1097/CJI.0000000000000433. [Google Scholar] [PubMed] [CrossRef]
26. Kichenadasse G, Miners JO, Mangoni AA, Rowland A, Sorich MJ, Hopkins AM, et al. Effect of concomitant use of antihypertensives and immune check point inhibitors on cancer outcomes. J Hypertens. 2021;39(7):1274–81. doi:10.1097/HJH.0000000000002799. [Google Scholar] [PubMed] [CrossRef]
27. Bessede A, Marabelle A, Guégan JP, Danlos FX, Cousin S, Peyraud F, et al. Impact of acetaminophen on the efficacy of immunotherapy in cancer patients. Ann Oncol. 2022;33(9):909–15. doi:10.1016/j.annonc.2022.05.010. [Google Scholar] [PubMed] [CrossRef]
28. Hong S, Lee JH, Heo JY, Suh KJ, Kim SH, Kim YJ, et al. Impact of concurrent medications on clinical outcomes of cancer patients treated with immune checkpoint inhibitors: analysis of health insurance review and assessment data. J Cancer Res Clin Oncol. 2024;150(4):186. doi:10.1007/s00432-024-05728-z. [Google Scholar] [PubMed] [CrossRef]
29. Colard-Thomas J, Thomas QD, Viala M. Comedications with immune checkpoint inhibitors: involvement of the microbiota, impact on efficacy and practical implications. Cancers. 2023;15(8):2276. doi:10.3390/cancers15082276. [Google Scholar] [PubMed] [CrossRef]
30. Tomita Y, Goto Y, Sakata S, Imamura K, Minemura A, Oka K, et al. Clostridium butyricum therapy restores the decreased efficacy of immune checkpoint blockade in lung cancer patients receiving proton pump inhibitors. Oncoimmunology. 2022;11(1):2081010. doi:10.1080/2162402X.2022.2081010. [Google Scholar] [PubMed] [CrossRef]
31. Geeviman K, Babu D, Prakash Babu P. Pantoprazole induces mitochondrial apoptosis and attenuates NF-κB signaling in glioma cells. Cell Mol Neurobiol. 2018;38(8):1491–504. doi:10.1007/s10571-018-0623-4. [Google Scholar] [PubMed] [CrossRef]
32. Bridoux M, Simon N, Turpin A. Proton pump inhibitors and cancer: current state of play. Front Pharmacol. 2022;13:798272. doi:10.3389/fphar.2022.798272. [Google Scholar] [PubMed] [CrossRef]
33. Gao L, Liu Y, Liu J, Li J, Liu Y, Meng F, et al. Proton pump inhibitors stabilize the expression of PD-L1 on cell membrane depending on the phosphorylation of GSK3β. Cancer Med. 2024;13(10):e7083. doi:10.1002/cam4.v13.10. [Google Scholar] [CrossRef]
34. Hussain N, Naeem M, Pinato DJ. Concomitant medications and immune checkpoint inhibitor therapy for cancer: causation or association? Hum Vaccin Immunother. 2021;17(1):55–61. doi:10.1080/21645515.2020.1769398. [Google Scholar] [PubMed] [CrossRef]
35. Handa O, Yoshida N, Fujita N, Tanaka Y, Ueda M, Takagi T, et al. Molecular mechanisms involved in anti-inflammatory effects of proton pump inhibitors. Inflamm Res. 2006;55(11):476–80. doi:10.1007/s00011-006-6056-4. [Google Scholar] [PubMed] [CrossRef]
36. Kedika RR, Souza RF, Spechler SJ. Potential anti-inflammatory effects of proton pump inhibitors: a review and discussion of the clinical implications. Dig Dis Sci. 2009;54(11):2312–7. doi:10.1007/s10620-009-0951-9. [Google Scholar] [PubMed] [CrossRef]
37. Balar AV, Galsky MD, Rosenberg JE, Powles T, Petrylak DP, Bellmunt J, et al. Atezolizumab as first-line treatment in cisplatin-ineligible patients with locally advanced and metastatic urothelial carcinoma: a single-arm, multicentre, phase 2 trial. The Lancet. 2017;389(10064):67–76. doi:10.1016/S0140-6736(16)32455-2. [Google Scholar] [PubMed] [CrossRef]
38. Powles T, Durán I, Van der Heijden MS, Loriot Y, Vogelzang NJ, De Giorgi U, et al. Atezolizumab versus chemotherapy in patients with platinum-treated locally advanced or metastatic urothelial carcinoma (IMvigor211a multicentre, open-label, phase 3 randomised controlled trial. The Lancet. 2018;391(10122):748–57. doi:10.1016/S0140-6736(17)33297-X. [Google Scholar] [PubMed] [CrossRef]
39. Hopkins AM, Kichenadasse G, Karapetis CS, Rowland A, Sorich MJ. Concomitant proton pump inhibitor use and survival in urothelial carcinoma treated with atezolizumab. Clin Cancer Res. 2020;26(20):5487–93. doi:10.1158/1078-0432.CCR-20-1876. [Google Scholar] [PubMed] [CrossRef]
40. Tomisaki I, Harada M, Sakano S, Terado M, Hamasuna R, Harada S, et al. Differential impact of proton pump inhibitor on survival outcomes of patients with advanced urothelial carcinoma treated with chemotherapy versus pembrolizumab. Int J Urol. 2023;30(9):738–45. doi:10.1111/iju.v30.9. [Google Scholar] [CrossRef]
41. Okuyama Y, Hatakeyama S, Numakura K, Narita T, Tanaka T, Miura Y, et al. Prognostic impact of proton pump inhibitors for immunotherapy in advanced urothelial carcinoma. BJUI Compass. 2022;3(2):154–61. doi:10.1002/bco2.118. [Google Scholar] [PubMed] [CrossRef]
42. Sekito T, Bekku K, Katayama S, Watanabe T, Tsuboi I, Yoshinaga K, et al. Effect of antacids on the survival of patients with metastatic urothelial carcinoma treated with pembrolizumab. Clin Genitourin Cancer. 2024;22(4):102097. doi:10.1016/j.clgc.2024.102097. [Google Scholar] [PubMed] [CrossRef]
43. Fukuokaya W, Kimura T, Komura K, Uchimoto T, Nishimura K, Yanagisawa T, et al. Effectiveness of pembrolizumab in patients with urothelial carcinoma receiving proton pump inhibitors. Urol Oncol: Semin Original Investig. 2022;40(7):346.e1–8. doi:10.1016/j.urolonc.2022.02.020. [Google Scholar] [PubMed] [CrossRef]
44. Kunimitsu Y, Morio K, Hirata S, Yamamoto K, Omura T, Hara T, et al. Effects of proton pump inhibitors on survival outcomes in patients with metastatic or unresectable urothelial carcinoma treated with pembrolizumab. Biol Pharm Bull. 2022;45(5):b21–00939. doi:10.1248/bpb.b21-00939. [Google Scholar] [PubMed] [CrossRef]
45. Iida K, Naiki T, Etani T, Nagari T, Sugiyama Y, Isobe T, et al. Proton pump inhibitors and potassium competitive acid blockers decrease pembrolizumab efficacy in patients with metastatic urothelial carcinoma. Sci Rep. 2024;14(1):97. doi:10.1038/s41598-024-53158-1. [Google Scholar] [PubMed] [CrossRef]
46. Ruiz-Bañobre J, Molina-Díaz A, Fernández-Calvo O, Fernández-Núñez N, Medina-Colmenero A, Santomé L, et al. Rethinking prognostic factors in locally advanced or metastatic urothelial carcinoma in the immune checkpoint blockade era: a multicenter retrospective study. ESMO Open. 2021;6(2):100090. doi:10.1016/j.esmoop.2021.100090. [Google Scholar] [PubMed] [CrossRef]
47. Taguchi S, Kawai T, Buti S, Bersanelli M, Uemura Y, Kishitani K, et al. Validation of a drug-based score in advanced urothelial carcinoma treated with pembrolizumab. Immunotherapy. 2023;15(11):827–37. doi:10.2217/imt-2023-0028. [Google Scholar] [PubMed] [CrossRef]
48. Zhang L, Chen C, Chai D, Li C, Kuang T, Liu L, et al. Effects of PPIs use on clinical outcomes of urothelial cancer patients receiving immune checkpoint inhibitor therapy. Front Pharmacol. 2022;13:1413. doi:10.3389/fphar.2022.1018411. [Google Scholar] [PubMed] [CrossRef]
49. Cao X, Wang Y, Huang W, Li P, Guo C, Li Y. The impact of concomitant proton pump inhibitors therapy on clinical outcome of cancer patients treated with immune checkpoint inhibitors. Am J Clin Oncol. 2023;46(4):142–9. doi:10.1097/COC.0000000000000991. [Google Scholar] [PubMed] [CrossRef]
50. Qin BD, Jiao XD, Zhou XC, Shi B, Wang J, Liu K, et al. Effects of concomitant proton pump inhibitor use on immune checkpoint inhibitor efficacy among patients with advanced cancer. Oncoimmunology. 2021;10(1):8:e001361. doi:10.1080/2162402X.2021.1929727. [Google Scholar] [PubMed] [CrossRef]
51. Zhou C-B, Zhou Y-L, Fang J-Y. Gut microbiota in cancer immune response and immunotherapy. Trends Cancer. 2021;7(7):647–60. doi:10.1016/j.trecan.2021.01.010. [Google Scholar] [PubMed] [CrossRef]
52. Gopalakrishnan V, Sellman BR, Cohen TS, Dennis PA. Antibiotics and immunotherapy: too much of anything is bad!. Eur Urol. 2020;78(4):544–5. doi:10.1016/j.eururo.2020.07.027. [Google Scholar] [PubMed] [CrossRef]
53. Iida N, Dzutsev A, Stewart CA, Smith L, Bouladoux N, Weingarten RA, et al. Commensal bacteria control cancer response to therapy by modulating the tumor microenvironment. Science. 2013;342(6161):967–70. doi:10.1126/science.1240527. [Google Scholar] [PubMed] [CrossRef]
54. Vétizou M, Pitt JM, Daillère R, Lepage P, Waldschmitt N, Flament C, et al. Anticancer immunotherapy by CTLA-4 blockade relies on the gut microbiota. Science. 2015;350(6264):1079–84. doi:10.1126/science.aad1329. [Google Scholar] [PubMed] [CrossRef]
55. Routy B, Le Chatelier E, Derosa L, Duong CPM, Tidjani Alou M, Daillère R, et al. Gut microbiome influences efficacy of PD-1-based immunotherapy against epithelial tumors. Science. 2018;359(6371):91–7. doi:10.1126/science.aan3706. [Google Scholar] [PubMed] [CrossRef]
56. Jakobsson HE, Jernberg C, Andersson AF, Sjölund-Karlsson M, Jansson JK, Engstrand L. Short-term antibiotic treatment has differing long-term impacts on the human throat and gut microbiome. PLoS One. 2010;5(3):e9836. doi:10.1371/journal.pone.0009836. [Google Scholar] [PubMed] [CrossRef]
57. Wu Q, Liu J, Wu S, Xie X. The impact of antibiotics on efficacy of immune checkpoint inhibitors in malignancies: a study based on 44 cohorts. Int Immunopharmacol. 2021;92:107303. doi:10.1016/j.intimp.2020.107303. [Google Scholar] [PubMed] [CrossRef]
58. Crespin A, Le Bescop C, de Gunzburg J, Vitry F, Zalcman G, Cervesi J, et al. A systematic review and meta-analysis evaluating the impact of antibiotic use on the clinical outcomes of cancer patients treated with immune checkpoint inhibitors. Front Oncol. 2023;13:1065004. [Google Scholar]
59. Zhou J, Huang G, Wong W-C, Hu D-H, Zhu J-W, Li R, et al. The impact of antibiotic use on clinical features and survival outcomes of cancer patients treated with immune checkpoint inhibitors. Front Immunol. 2022;13:968729. doi:10.3389/fimmu.2022.968729. [Google Scholar] [PubMed] [CrossRef]
60. Lurienne L, Cervesi J, Duhalde L, de Gunzburg J, Andremont A, Zalcam G, et al. NSCLC immunotherapy efficacy and antibiotic use: a systematic review and meta-analysis. J Thorac Oncol. 2020;15(7):1147–59. doi:10.1016/j.jtho.2020.03.002. [Google Scholar] [PubMed] [CrossRef]
61. Luo Z, Hao S, Li Y, Zhou X, Gunes EG, Liu S, et al. The negative effect of antibiotics on RCC patients with immunotherapy: a systematic review and meta-analysis. Front Immunol. 2022;13:1065004. doi:10.3389/fimmu.2022.1065004. [Google Scholar] [PubMed] [CrossRef]
62. Poizeau F, Kerbrat S, Balusson F, Tattevin P, Revest M, Cattoir V, et al. The association between antibiotic use and outcome among metastatic melanoma patients receiving immunotherapy. J Nat Cancer Inst. 2022;114(5):686–94. doi:10.1093/jnci/djac019. [Google Scholar] [PubMed] [CrossRef]
63. Agarwal A, Pond GR, Curran C, Nassar AH, Nuzzo PV, Kumar V, et al. Impact of concurrent medications on outcomes with PD1/PD-L1 inhibitors for metastatic urothelial carcinoma. J Clin Oncol. 2019;37(7_suppl):435–5. doi:10.1200/JCO.2019.37.7_suppl.435. [Google Scholar] [CrossRef]
64. Khan MS, Radakovich N, Ornstein M, Gupta S. 778P Concomitant antibiotic use and its effect on immune-checkpoint inhibitor efficacy in patients with advanced urothelial carcinoma. Ann Oncol. 2020;31:S597. doi:10.1016/j.annonc.2020.08.850. [Google Scholar] [CrossRef]
65. Derosa L, Hellmann MD, Spaziano M, Halpenny D, Fidelle M, Rizvi, et al. Negative association of antibiotics on clinical activity of immune checkpoint inhibitors in patients with advanced renal cell and non-small-cell lung cancer. Ann Oncol. 2018;29(6):1437–44. doi:10.1093/annonc/mdy103. [Google Scholar] [PubMed] [CrossRef]
66. Pinato DJ, Howlett S, Ottaviani D, Urus H, Patel A, Mineo T, et al. Association of prior antibiotic treatment with survival and response to immune checkpoint inhibitor therapy in patients with cancer. JAMA Oncol. 2019;5(12):1774–8. doi:10.1001/jamaoncol.2019.2785. [Google Scholar] [PubMed] [CrossRef]
67. Huang L, Chen X, Zhou L, Xu Q, Xie J, Zhan P, Lv T, et al. Antibiotic exposure windows and the efficacy of immune checkpoint blockers in patients with cancer: a meta-analysis. Ann Palliat Med. 2021;10(3):2709–22. doi:10.21037/apm. [Google Scholar] [CrossRef]
68. Khan U, Ho K, Hwang EK, Peña C, Brouwer J, Hoffman K, et al. Impact of use of antibiotics on response to immune checkpoint inhibitors and tumor microenvironment. Am J Clin Oncol: Cancer Clin Trials. 2021;44(6):247–53. doi:10.1097/COC.0000000000000813. [Google Scholar] [PubMed] [CrossRef]
69. Pinter M, Jain RK. Targeting the renin-angiotensin system to improve cancer treatment: implications for immunotherapy. Sci Transl Med. 2017;9(410):eaan5616. doi:10.1126/scitranslmed.aan5616. [Google Scholar] [PubMed] [CrossRef]
70. Vallejo-Ardila DL, Fifis T, Burrell LM, Walsh K, Christophi. Renin-angiotensin inhibitors reprogram tumor immune microenvironment: a comprehensive view of the influences on anti-tumor immunity. Oncotarget. 2018;9(84):35500–11. doi:10.18632/oncotarget.v9i84. [Google Scholar] [CrossRef]
71. Xie G, Cheng T, Lin J, Zhang L, Liu Y, Xie G, et al. Local angiotensin II contributes to tumor resistance to checkpoint immunotherapy. J Immunother Cancer. 2018;6(1):88. doi:10.1186/s40425-018-0401-3. [Google Scholar] [PubMed] [CrossRef]
72. Yang K, Zhou J, Chen Y, Chen Y, Chen L, Zhang P, et al. Angiotensin II contributes to intratumoral immunosuppression via induction of PD-L1 expression in non-small cell lung carcinoma. Int Immunopharmacol. 2020;84:106507. doi:10.1016/j.intimp.2020.106507. [Google Scholar] [PubMed] [CrossRef]
73. Nakamura K, Kiniwa Y, Okuyama R. CCL5 production by fibroblasts through a local renin-angiotensin system in malignant melanoma affects tumor immune responses. J Cancer Res Clin Oncol. 2021;147(7):1993–2001. doi:10.1007/s00432-021-03612-8. [Google Scholar] [PubMed] [CrossRef]
74. Chauhan VP, Chen IX, Tong R, Rosa NM, Martin JD, Naxerova K, et al. Reprogramming the microenvironment with tumor-selective angiotensin blockers enhances cancer immunotherapy. Proc Nat Acad Sci. 2019;116(22):10674–80. doi:10.1073/pnas.1819889116. [Google Scholar] [PubMed] [CrossRef]
75. George AJ, Thomas WG, Hannan RD. The renin-angiotensin system and cancer: old dog, new tricks. Nat Rev Cancer. 2010;10(11):745–59. doi:10.1038/nrc2945. [Google Scholar] [PubMed] [CrossRef]
76. Chauhan VP, Martin JD, Liu H, Lacorre DA, Jain SR, Kozin SV, et al. Angiotensin inhibition enhances drug delivery and potentiates chemotherapy by decompressing tumour blood vessels. Nat Commun. 2013;4(1):2516. doi:10.1038/ncomms3516. [Google Scholar] [PubMed] [CrossRef]
77. Mariathasan S, Turley SJ, Nickles D, Castiglioni A, Yuen K, Wang Y, et al. TGFβ attenuates tumour response to PD-L1 blockade by contributing to exclusion of T cells. Nature. 2018;554(7693):544–8. doi:10.1038/nature25501. [Google Scholar] [PubMed] [CrossRef]
78. Liu H, Naxerova K, Pinter M, Incio J, Lee H, Kohei S, et al. Use of angiotensin system inhibitors is associated with immune activation and longer survival in nonmetastatic pancreatic ductal adenocarcinoma. Clin Cancer Res. 2017;23(19):5959–69. doi:10.1158/1078-0432.CCR-17-0256. [Google Scholar] [PubMed] [CrossRef]
79. Nakamura K, Yaguchi T, Ohmura G, Kobayashi A, Kawamura N, Iwata T, et al. Involvement of local renin-angiotensin system in immunosuppression of tumor microenvironment. Cancer Sci. 2018;109(1):54–64. doi:10.1111/cas.2018.109.issue-1. [Google Scholar] [CrossRef]
80. Qiao G, Chen M, Bucsek MJ, Repasky EA, Hylander BL. Adrenergic signaling: a targetable checkpoint limiting development of the antitumor immune response. Front Immunol. 2018;9:164. doi:10.3389/fimmu.2018.00164. [Google Scholar] [PubMed] [CrossRef]
81. Cole SW, Sood AK. Molecular pathways: beta-adrenergic signaling in cancer. Clin Cancer Res. 2012;18(5):1201–6. doi:10.1158/1078-0432.CCR-11-0641. [Google Scholar] [PubMed] [CrossRef]
82. Bucsek MJ, Qiao G, MacDonald CR, Giridharan T, Evans L, Niedzwecki B, et al. β-adrenergic signaling in mice housed at standard temperatures suppresses an effector phenotype in CD8+ T cells and undermines checkpoint inhibitor therapy. Cancer Res. 2017;77(20):5639–51. doi:10.1158/0008-5472.CAN-17-0546. [Google Scholar] [PubMed] [CrossRef]
83. Kokolus KM, Zhang Y, Sivik JM, Schmeck C, Zhu J, Repasky EA, et al. Beta blocker use correlates with better overall survival in metastatic melanoma patients and improves the efficacy of immunotherapies in mice. Oncoimmunology. 2018;7(3):e1405205. doi:10.1080/2162402X.2017.1405205. [Google Scholar] [PubMed] [CrossRef]
84. Strauss J, Rajan A, Apolo A, Lee JM, Thomas A, Chen A, et al. Impact of angiotensin II pathway inhibition on tumor response to anti PD(L)1 based therapy. J Immunother Cancer. 2020;8(Suppl 3):A276–7. [Google Scholar]
85. Nuzzo PV, Adib E, Weise N, Curran C, Stewart T, Freeman D, et al. Impact of renin-angiotensin system inhibitors on outcomes in patients with metastatic renal cell carcinoma treated with immune-checkpoint inhibitors. Clin Genitourin Cancer. 2022;20(4):301–6. doi:10.1016/j.clgc.2022.04.012. [Google Scholar] [PubMed] [CrossRef]
86. Medjebar S, Truntzer C, Perrichet A, Limagne E, Fumet JD, Richard C, et al. Angiotensin-converting enzyme (ACE) inhibitor prescription affects non-small-cell lung cancer (NSCLC) patients response to PD-1/PD-L1 immune checkpoint blockers. Oncoimmunology. 2020;9(1):1836766. doi:10.1080/2162402X.2020.1836766. [Google Scholar] [PubMed] [CrossRef]
87. Oren O, Yang EH, Molina JR, Bailey KR, Blumenthal RS, Kopecky SL. Cardiovascular health and outcomes in cancer patients receiving immune checkpoint inhibitors. Am J Cardiol. 2020;125(12):1920–6. doi:10.1016/j.amjcard.2020.02.016. [Google Scholar] [PubMed] [CrossRef]
88. Oh MS, Guzner A, Wainwright DA, Mohindra NA, Chae YK, Behdad A, et al. The impact of beta blockers on survival outcomes in patients with non-small-cell lung cancer treated with immune checkpoint inhibitors. Clin Lung Cancer. 2021;22(1):e57–62. doi:10.1016/j.cllc.2020.07.016. [Google Scholar] [PubMed] [CrossRef]
89. Gandhi S, Pandey MR, Attwood K, Ji W, Witkiewicz AK, Knudsen ES, et al. Phase I clinical trial of combination propranolol and pembrolizumab in locally advanced and metastatic melanoma: safety, tolerability, and preliminary evidence of antitumor activity. Clin Cancer Res. 2021;27(1):87–95. doi:10.1158/1078-0432.CCR-20-2381. [Google Scholar] [PubMed] [CrossRef]
90. Kalfeist L, Galland L, Ledys F, Ghiringhelli F, Limagne E, Ladoire S. Impact of glucocorticoid use in oncology in the immunotherapy era. Cells. 2022;11(5):770. doi:10.3390/cells11050770. [Google Scholar] [PubMed] [CrossRef]
91. Ciccarese C, Iacovelli R, Buti S, Primi F, Astore S, Massari F, et al. Concurrent Nivolumab and Metformin in diabetic cancer patients: is it safe and more active? Anticancer Res. 2022;42(3):1487–93. doi:10.21873/anticanres.15620. [Google Scholar] [PubMed] [CrossRef]
92. Santoni M, Massari F, Matrana MR, Basso U, De Giorgi U, Autilio G, et al. Statin use improves the efficacy of nivolumab in patients with advanced renal cell carcinoma. Eur J Cancer. 2022;172:191–8. doi:10.1016/j.ejca.2022.04.035. [Google Scholar] [PubMed] [CrossRef]
93. Gauthaman K, Fong C, Bongso A. Statins, stem cells, and cancer. J Cell Biochem. 2009;106(6):975–83. doi:10.1002/jcb.22092. [Google Scholar] [PubMed] [CrossRef]
94. Farina HG, Bublik DR, Alonso DF, Gomez ED. Lovastatin alters cytoskeleton organization and inhibits experimental metastasis of mammary carcinoma cells. Clin Exp Metastasis. 2002;19(6):551–60. doi:10.1023/A:1020355621043. [Google Scholar] [PubMed] [CrossRef]
95. Zhou Q, Jiao Z, Liu Y, Devreotes PN, Zhang Z. The effects of statins in patients with advanced-stage cancers—a systematic review and meta-analysis. Front Oncol. 2023;13:144. doi:10.3389/fonc.2023.1234713. [Google Scholar] [PubMed] [CrossRef]
96. Cantini L, Pecci F, Hurkmans DP, Belderbos RA, Lanese A, Copparoni C, et al. High-intensity statins are associated with improved clinical activity of PD-1 inhibitors in malignant pleural mesothelioma and advanced non-small cell lung cancer patients. Eur J Cancer. 2021;144:41–8. doi:10.1016/j.ejca.2020.10.031. [Google Scholar] [PubMed] [CrossRef]
97. Ferro M, Marchioni M, Lucarelli G, Vartolomei MD, Soria F, Terraciano D, et al. Association of statin use and oncological outcomes in patients with first diagnosis of T1 high grade non-muscle invasive urothelial bladder cancer: results from a multicenter study. Minerva Urol Nephrol. 2022;73(6):796–802. [Google Scholar]
98. Liu K, Nicoletti R, Zhao H, Chen X, Chiu PK-F, Ng C-F, et al. The potential benefits of concomitant statins treatment in patients with non-muscle-invasive bladder cancer. BJU Int. 2024;73:796. doi:10.1111/bju.16493. [Google Scholar] [PubMed] [CrossRef]
99. Yu Z, Guo J, Liu Y, Wang M, Liu Z, Gao Y, et al. Nano delivery of simvastatin targets liver sinusoidal endothelial cells to remodel tumor microenvironment for hepatocellular carcinoma. J Nanobiotechnol. 2022;20(1):9. doi:10.1186/s12951-021-01205-8. [Google Scholar] [PubMed] [CrossRef]
100. Rossi A, Filetti M, Taurelli Salimbeni B, Piras M, Rizzo F, Giusti R, et al. Statins and immunotherapy: togetherness makes strength The potential effect of statins on immunotherapy for NSCLC. Cancer Rep. 2021;4(4):e1368. doi:10.1002/cnr2.v4.4. [Google Scholar] [CrossRef]
101. Haimerl L, Strobach D, Mannell H, Stief CG, Buchner A, Karl A, et al. Retrospective evaluation of the impact of non-oncologic chronic drug therapy on the survival in patients with bladder cancer. Int J Clin Pharm. 2022;44(2):339–47. doi:10.1007/s11096-021-01343-x. [Google Scholar] [PubMed] [CrossRef]
102. Chen YC, Li H, Wang J. Mechanisms of metformin inhibiting cancer invasion and migration. Am J Transl Res. 2020;12(9):4885–901. [Google Scholar] [PubMed]
103. Wang K, Chen Y, Zhang M, Wang S, Yao S, Gong Z, et al. Metformin suppresses gastric cancer progression by disrupting the STAT1-PRMT1 axis. Biochem Pharmacol. 2024;226:116367. doi:10.1016/j.bcp.2024.116367. [Google Scholar] [PubMed] [CrossRef]
104. Samuel S, Varghese E, Kubatka P, Triggle CR, Büsselberg D. Metformin: the answer to cancer in a flower? Current knowledge and future prospects of metformin as an anti-cancer agent in breast cancer. Biomolecules. 2019;9(12):846. doi:10.3390/biom9120846. [Google Scholar] [PubMed] [CrossRef]
105. Petrovic A, Jovanovic I, Stojanovic B, Stojanovic MD, Stojanovic BS, Jurisevic M, et al. Harnessing metformin’s immunomodulatory effects on immune cells to combat breast cancer. Int J Mol Sci. 2024;25(11):5869. doi:10.3390/ijms25115869. [Google Scholar] [PubMed] [CrossRef]
106. Park SH, Lee J, Yun HJ, Kim S-H, Lee J-H. Metformin suppresses both PD-L1 expression in cancer cells and cancer-induced PD-1 expression in immune cells to promote antitumor immunity. Ann Lab Med. 2024;44(5):426–36. doi:10.3343/alm.2023.0443. [Google Scholar] [PubMed] [CrossRef]
107. Li Y, Liu B, Cao Y, Cai L, Zhou Y, Yang W, et al. Metformin-induced reduction of CCR8 enhances the anti-tumor immune response of PD-1 immunotherapy in glioblastoma. Eur J Pharmacol. 2024;964:176274. doi:10.1016/j.ejphar.2023.176274. [Google Scholar] [PubMed] [CrossRef]
108. Lin H, Yiu DC-Y, Chin S, Liu K, Yip TC-F. Metformin in patients with hepatocellular carcinoma receiving immunotherapy. J Hepatol. 2023;78(5):e180–2. doi:10.1016/j.jhep.2022.12.011. [Google Scholar] [PubMed] [CrossRef]
109. Wang J, Lin J, Guo H, Wu W, Yang J, Mao J, et al. Prognostic impact of metformin in solid cancer patients receiving immune checkpoint inhibitors: novel evidences from a multicenter retrospective study. Front Pharmacol. 2024;15:108889. doi:10.3389/fphar.2024.1419498. [Google Scholar] [PubMed] [CrossRef]
110. Santoni M, Molina-Cerrillo J, Myint ZW, Massari F, Buchler T, Buti S, et al. Concomitant use of statins, metformin, or proton pump inhibitors in patients with advanced renal cell carcinoma treated with first-line combination therapies. Target Oncol. 2022;17(5):571–81. doi:10.1007/s11523-022-00907-9. [Google Scholar] [PubMed] [CrossRef]
111. Kennedy OJ, Kicinski M, Valpione S, Gandini S, Suciu S, Blank CU, et al. Prognostic and predictive value of metformin in the European organisation for research and treatment of cancer 1325/KEYNOTE-054 phase III trial of pembrolizumab versus placebo in resected high-risk stage III melanoma. Eur J Cancer. 2023;189:112900. doi:10.1016/j.ejca.2023.04.016. [Google Scholar] [PubMed] [CrossRef]
112. Rimini M, Montes M, Amadeo E, Vitiello F, Kudo M, Tada T, et al. Impact of metformin, statin, aspirin and insulin on the prognosis of uHCC patients receiving first line Lenvatinib or Atezolizumab plus Bevacizumab. Sci Rep. 2024;14(1):20200. doi:10.1038/s41598-024-70928-z. [Google Scholar] [PubMed] [CrossRef]
113. Yamaura K, Ogawa K, Yonekawa T, Nakamura T, Yano S, Ueno K. Inhibition of the antibody production by acetaminophen independent of liver injury in mice. Biol Pharm Bull. 2002;25(2):201–5. doi:10.1248/bpb.25.201. [Google Scholar] [PubMed] [CrossRef]
114. Falup-Pecurariu O, Man SC, Neamtu ML, Chicin G, Baciu G, Pitic C, et al. Effects of prophylactic ibuprofen and paracetamol administration on the immunogenicity and reactogenicity of the 10-valent pneumococcal non-typeable Haemophilus influenzae protein D conjugated vaccine (PHiD-CV) co-administered with DTPa-combined vaccines in children: an open-label, randomized, controlled, non-inferiority trial. Hum Vaccin Immunother. 2017;13(3):649–60. [Google Scholar] [PubMed]
115. Botticelli A, Cirillo A, Pomati G, Cerbelli B, Scagnoli S, Roberto M, et al. The role of opioids in cancer response to immunotherapy. J Transl Med. 2021;19(1):119. doi:10.1186/s12967-021-02784-8. [Google Scholar] [PubMed] [CrossRef]
116. Prasetya RA, Metselaar-Albers M, Engels F. Concomitant use of analgesics and immune checkpoint inhibitors in non-small cell lung cancer: a pharmacodynamics perspective. Eur J Pharmacol. 2021;906:174284. doi:10.1016/j.ejphar.2021.174284. [Google Scholar] [PubMed] [CrossRef]
117. Maher DP, Walia D, Heller NM. Suppression of human natural killer cells by different classes of opioids. Anesth Analg. 2019;128(5):1013–21. doi:10.1213/ANE.0000000000004058. [Google Scholar] [PubMed] [CrossRef]
118. Elkrief A, Montesion M, Sivakumar S, Hale C, Bowan AS, Bektaş AB, et al. Intratumoral escherichia is associated with improved survival to single-agent immune checkpoint inhibition in patients with advanced non-small-cell lung cancer. J Clin Oncol. 2024;42:3339–49. doi:10.1200/JCO.23.01488. [Google Scholar] [PubMed] [CrossRef]
119. Buti S, Bersanelli M, Perrone F, Bracarda S, Di Maio M, Giusti R, et al. Predictive ability of a drug-based score in patients with advanced non-small-cell lung cancer receiving first-line immunotherapy. Eur J Cancer. 2021;150:224–31. doi:10.1016/j.ejca.2021.03.041. [Google Scholar] [PubMed] [CrossRef]
120. Sivan A, Corrales L, Hubert N, Williams JB, Hubert N, Williams JB, et al. Commensal Bifidobacterium promotes antitumor immunity and facilitates anti-PD-L1 efficacy. Science. 2015;350(6264):1084–9. doi:10.1126/science.aac4255. [Google Scholar] [PubMed] [CrossRef]
121. Ciccarese C, Porcari S, Buti S, Fornarini G, Primi F, Giudice GC, et al. LBA77 Fecal microbiota transplantation (FMT) versus placebo in patients receiving pembrolizumab plus axitinib for metastatic renal cell carcinoma: preliminary results of the randomized phase II TACITO trial. Ann Oncol. 2024;35:S1264. doi:10.1016/j.annonc.2024.08.2320. [Google Scholar] [CrossRef]
122. Dizman N, Meza L, Bergerot P, Alcantara M, Dorff T, Lyou Y, et al. Nivolumab plus ipilimumab with or without live bacterial supplementation in metastatic renal cell carcinoma: a randomized phase 1 trial. Nat Med. 2022;28(4):704–12. doi:10.1038/s41591-022-01694-6. [Google Scholar] [PubMed] [CrossRef]
123. Ebrahimi H, Dizman N, Meza L, Malhotra J, Li X, Dorff T, et al. Cabozantinib and nivolumab with or without live bacterial supplementation in metastatic renal cell carcinoma: a randomized phase 1 trial. Nat Med. 2024;30(9):2576–85. doi:10.1038/s41591-024-03086-4. [Google Scholar] [PubMed] [CrossRef]
124. Medik YB, Zhou Y, Kahn LM, Patel B, Babcock R, Chrisikos T, et al. Outcome of concurrent treatment with a-CTLA4 and metronidazole in murine model of colon adenocarcinoma. J Clin Oncol. 2021;39(15_suppl):e14566. doi:10.1200/JCO.2021.39.15_suppl.e14566. [Google Scholar] [CrossRef]
125. Cortellini A, Facchinetti F, Derosa L, Pinato DJ. Antibiotic exposure and immune checkpoint inhibitors in patients with NSCLC: the backbone matters. J Thorac Oncol. 2022;17(6):739–41. doi:10.1016/j.jtho.2022.03.016. [Google Scholar] [PubMed] [CrossRef]
126. Monge BMC, Xie C, Mabry-Hrones D, Wood BJ, Steinberg SM, Kleiner DE, et al. Phase II study of nivolumab (anti-PD1tadalafil, and oral vancomycin in patients with refractory primary hepatocellular carcinoma or liver dominant metastatic cancer from colorectal or pancreatic cancers. J Clin Oncol. 2020;38(15_suppl):TPS4656. doi:10.1200/JCO.2020.38.15_suppl.TPS4656. [Google Scholar] [CrossRef]
127. Cha J-H, Yang W-H, Xia W, Wei Y, Chan L-C, Lim S-O, et al. Metformin promotes antitumor immunity via endoplasmic-reticulum-associated degradation of PD-L1. Mol Cell. 2018;71(4):606–620.e7. doi:10.1016/j.molcel.2018.07.030. [Google Scholar] [PubMed] [CrossRef]
128. Wang Z, Lu C, Zhang K, Lin C, Wu F, Tang X, et al. Metformin combining PD-1 inhibitor enhanced anti-tumor efficacy in STK11 mutant lung cancer through AXIN-1-dependent inhibition of STING ubiquitination. Front Mol Biosci. 2022;9:780200. doi:10.3389/fmolb.2022.780200. [Google Scholar] [PubMed] [CrossRef]
129. Liu W, Wang Y, Luo J, Liu M, Luo Z. Pleiotropic effects of metformin on the antitumor efficiency of immune checkpoint inhibitors. Front Immunol. 2021;11:586760. doi:10.3389/fimmu.2020.586760. [Google Scholar] [PubMed] [CrossRef]
130. Kubo T, Ninomiya T, Hotta K, Kozuki T, Toyooka S, Okada H, et al. Study protocol: phase-Ib trial of Nivolumab combined with metformin for refractory/recurrent solid tumors. Clin Lung Cancer. 2018;19(6):e861–4. doi:10.1016/j.cllc.2018.07.010. [Google Scholar] [PubMed] [CrossRef]
Cite This Article
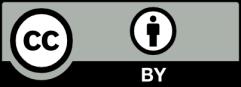
This work is licensed under a Creative Commons Attribution 4.0 International License , which permits unrestricted use, distribution, and reproduction in any medium, provided the original work is properly cited.