Open Access
REVIEW
Cancer stem cell specificity as new targets in breast tumor treatment
1 Department of Breast Surgery, The First Affiliated Hospital of Nanjing Medical University, Nanjing, 210029, China
2 Department of Pathology, Jiangsu Provincial People’s Hospital, Nanjing, 210029, China
3 Department of Gynaecology and Obstetrics, No. 908th Hospital of Chinese PLA Joint Logistic Support Force, Nanchang, 330000, China
4 First Clinical Medical College, Nanjing University of Chinese Medicine, Nanjing, 210023, China
5 Department of Outpatient, Eastern Theater General Hospital, Nanjing, 210016, China
6 Zhejiang ZhongNuoMei Biotech Limited Company, Hangzhou, 310000, China
7 School of Chinese Medicine, Nanjing University of Chinese Medicine, Nanjing, 210023, China
* Corresponding Authors: TIANSONG XIA. Email: ; BO XU. Email:
# These authors contributed equally to this work
(This article belongs to the Special Issue: Novel Targets and Biomarkers in Solid Tumors)
Oncology Research 2025, 33(4), 811-819. https://doi.org/10.32604/or.2024.050505
Received 08 February 2024; Accepted 15 July 2024; Issue published 19 March 2025
Abstract
Background: Breast cancer is a prevalent malignant tumor affecting females, with treatment options including surgery, radiotherapy, chemotherapy, and endocrine therapy. Methods: This review synthesizes existing literature on breast cancer stem cells and their applications in breast cancer treatment. PubMed, Web of Science, and other relevant databases were systematically searched using keywords such as “breast cancer stem cells,” “immunotherapy,” “gene therapy,” and “cell therapy.” Studies published in English were included, and their findings were analyzed to provide insights into the characteristics and therapeutic potential of breast cancer stem cells. Results: Breast cancer stem cells exhibit unique properties that contribute to tumor initiation, progression, recurrence, and therapy resistance. Immunotherapy targeting breast cancer stem cells shows promise in overcoming these challenges, but issues such as lack of specificity and drug resistance need to be addressed. Conclusions: Breast cancer stem cells represent promising targets for innovative therapeutic strategies aimed at improving treatment outcomes in breast cancer patients.Keywords
Breast cancer is one of the most common malignant tumors in women, and its incidence is rising all over the world [1]. Although there are a variety of treatment methods that can be used to treat breast cancer, all of these treatment effects still have shortcomings such as tumor recurrence [2], drug resistance, and other issues [3]. Research and exploration into breast cancer treatment is therefore particularly important [4,5].
Current traditional treatments for breast cancer primarily include surgical resection, radiotherapy, and chemotherapy [6], all of which target cancer cells for destruction directly. However, the discovery of the existence of breast cancer stem cells has brought challenges to traditional treatment methods because these cells have strong self-renewal capabilities and promote drug resistance and are thus prone to tumor recurrence and metastasis [7]. Therefore, it is necessary to explore novel treatment methods that can kill and eliminate the specific characteristics of breast cancer stem cells, in order to achieve better therapeutic outcomes [8]. Stem cell therapy is one such specific treatment method, and it kills and eliminates breast cancer stem cells by utilizing their own multi-directional differentiation and self-renewal abilities [9].
With the deepening development of stem cell research, more and more studies have shown that stem cell therapy can be used as a specific treatment strategy for breast cancer stem cells [10]. At present, however, the research on stem cell therapy for breast cancer stem cells is relatively limited, and more in-depth research and exploration of its mechanism, therapeutic effect, and safety are needed [7,11]. Thus, this article aims to review the role of breast cancer stem cell specificity in the treatment of breast cancer, analyze the application prospects and existing problems of stem cell therapy in the treatment of breast cancer, and provide a reference for the future treatment of breast cancer [12].
In this review, we propose to explore the role of breast cancer stem cell specificity as a novel target in breast cancer treatment. We hypothesize that understanding and targeting the unique characteristics of breast cancer stem cells could lead to more effective therapeutic interventions and improved patient outcomes. By critically evaluating the current state of stem cell therapy in breast cancer treatment, we aim to identify potential avenues for further research and development, ultimately contributing to the advancement of personalized treatment strategies for breast cancer patients.
Characteristics of Breast Cancer Stem Cells
Breast cancer stem cells (BCSCs) are stem cells with the abilities of self-renewal, self-repair, and multi-directional differentiation [13,14]. Compared to ordinary cancer cells, the characteristics of breast cancer stem cells include the following additional aspects, discussed below.
One of the characteristics of stem cells is their ability to self-renew. Self-renewal refers to the ability of stem cells to continuously divide and produce stem cells like themselves, thereby maintaining a stable population [15,16]. Furthermore, stem cells can also generate various types of other cells through their limited differentiation ability [17], and these other cells can participate in the tissue repair and regeneration process. However, research [18] described that breast cancer stem cells (BCSCs) from two cell lines have different proliferative capacities, which would postulate that they are not stable, and this quality would be important to elucidate in order to choose the cell line as a drug carrier or in case of replacement therapies. It has been reported that the CD44high phenotype stands out as a prominent CSC antigen, whereas the CD44high/CD24low phenotype serves as one of the key identifiers for these cells in invasive breast cancer [18]. This ability of self-renewal and differentiation gives stem cells broad application prospects in many fields, such as tissue engineering, regenerative medicine, and drug discovery [19].
Self-renewal also plays a key role in breast cancer [20]. Breast cancer stem cells can not only continually divide into stem cells but can also produce cancer cells of nonstem-cell type, thus promoting the formation, proliferation, and metastasis of tumors [21]. In this process, breast cancer stem cells show high sensitivity to a variety of signal pathways, such as Notch, Wnt, Hedgehog, and PI3K/Akt [22]. Each of these signal pathways can activate self-renewal and differentiation BCSCs, thereby maintaining and expanding tumor cell populations [23].
The role of BCSCs in immunotherapy is an area of major focus in current research. Immunotherapy can achieve the goal of treating breast cancer by activating the patient’s own immune system in order to attack and eliminate tumor cells. However, as the “seed” of tumor cells, BCSCs also play an important role in immunotherapy [24,25]. Research showed that BCSCs have certain immune escape capabilities [26]. They can evade immune attacks by inhibiting T cell activity and inhibiting tumor cell apoptosis. In addition, BCSCs can quickly and easily proliferate and regenerate, allowing them to recover and further expand after immunotherapy [27]. Therefore, when treating breast cancer, treating BCSCs directly is of paramount importance in maximizing efficacy [28].
In the quest to effectively combat breast cancer, understanding the intricate interplay between BCSCs and the immune system is paramount. This interaction serves as a critical determinant of tumor progression and therapeutic response. Therefore, a comprehensive approach encompassing various facets is necessary to address this challenge effectively. Firstly, delving into the interaction mechanism between BCSCs and immune cells is crucial. Research must focus on elucidating how BCSCs evade immune surveillance and establish an immunosuppressive microenvironment conducive to their survival and proliferation. By deciphering these intricate molecular pathways, novel therapeutic targets can be identified to reinvigorate the immune response against BCSCs. Strategies such as immune checkpoint inhibitors, chimeric antigen receptor (CAR) T-cell therapy, and cancer vaccines hold promise in activating immune cells to specifically target and eliminate BCSCs.
BCSCs possess inherent plasticity and can regenerate tumors even after conventional treatments. Therefore, efforts should be directed toward developing targeted therapies that disrupt the self-renewal pathways of BCSCs, rendering them susceptible to eradication. Small molecules, peptides, or nucleic acid-based therapeutics targeting key regulators of BCSC self-renewal, such as Notch, Wnt, or Hedgehog signaling pathways, present potential avenues for intervention. Additionally, combination therapies incorporating conventional cytotoxic agents with BCSC-targeted agents can synergistically enhance treatment efficacy by preventing tumor recurrence [29].
Investigating the role of BCSCs within the TME is essential. The TME provides a nurturing niche for BCSCs, promoting their survival, invasion, and metastasis. Understanding the dynamic crosstalk between BCSCs and stromal components within the TME is crucial for devising therapeutic strategies to disrupt this supportive milieu. Approaches such as targeting tumor-associated macrophages, cancer-associated fibroblasts, and angiogenic factors can remodel the TME, rendering it inhospitable for BCSC proliferation and dissemination [30].
In line with these research endeavors, immunotherapy emerges as a promising modality for BCSC eradication. By harnessing the power of the immune system, immunotherapeutic interventions can unleash a robust and durable anti-tumor response. Immune checkpoint inhibitors, such as programmed cell death protein 1 (PD-1) and cytotoxic T-lymphocyte-associated protein 4 (CTLA-4) inhibitors, can unleash T-cell-mediated cytotoxicity against BCSCs, thereby impeding tumor progression. Additionally, strategies aimed at activating the apoptosis pathway specifically in BCSCs hold immense therapeutic potential, leading to their selective elimination while sparing normal cells. Furthermore, stem-cell-specific targeted therapies represent a burgeoning field in BCSC eradication. Monoclonal antibodies and small-molecule inhibitors targeting surface markers or signaling pathways enriched in BCSCs offer precision and efficacy in tumor eradication. By exploiting BCSC-specific vulnerabilities, these targeted agents can disrupt crucial survival and proliferative pathways, leading to BCSC depletion and tumor regression. Moreover, combination regimens incorporating both immunotherapy and targeted therapy hold promise in overcoming treatment resistance and achieving durable responses in breast cancer patients [31].
To begin, immunotherapy can treat breast cancer by activating T cells and attacking tumor stem cells [32]. In addition, immunotherapy can activate the apoptosis pathway for BCSCs [33]. Certain stem-cell-specific targeted therapies such as monoclonal antibodies and small-molecule targeted drugs can also kill BCSCs directly [34] and can also inhibit the regeneration and proliferation of BCSCs to a certain extent [35,36].
The role of BCSCs in the TME is also a major focus of current cancer research [37]. BCSCs can regulate the TME by secreting various cytokines and growth factors, thus promoting the growth and spread of tumors [38], and regulating the immune cells in the TME can thus help to inhibit the growth and proliferation of BCSCs [39,40]. Related targets of BCSCs are summarized in Table 1.
BCSCs are always the source of tumors. Therefore, gene therapy that targets BCSCs is an effective therapeutic strategy [51], and many genes have been found to inhibit the proliferation of BCSCs [52]. For example, the p53 gene is an important tumor suppressor gene, and its abnormal expression in breast cancer is closely related to tumor malignancy. By introducing the p53 gene into BCSCs, the proliferation of tumor stem cells can be effectively inhibited. Although gene therapy is a relatively new treatment method [53], it has already been widely discussed in the treatment of BCSCs [54]. In addition, miRNA is also an important gene therapy target. Studies have shown that miRNA plays an important role in BCSC self-renewal and proliferation, and crucially, introducing certain specific miRNAs into BCSCs has actually been found to inhibit their proliferation and differentiation [51]. Furthermore, some genes can also exert therapeutic effects on BCSCs by regulating the immune escape of tumor stem cells and enhancing tumor immune response (Table 2, Fig. 1).
Figure 1: Mechanisms of BCSCs. Therapeutic mechanisms for targeting BCSCs (BCSCs) include immunotherapy using Anti-CD47, Anti-PD-1, and Anti-PD-L1; blocking self-renewal and proliferation by targeting HER2, CD44, Notch, and Wnt pathways; and inducing apoptosis by activating p53.
Regarding target cells, BCSCs can be susceptible to various therapeutic cells, including self antigen-specific T cells and TATA-directed T cells, along with anti-tumor NK cells [60]. Cytotoxic T lymphocytes (CTLs) and natural killer cells (NK cells) are two common types of immune cells [52,61] that play an anti-tumor role by recognizing and killing tumor cells expressing certain antigens. Modified immune cells include transgenic T cells, and chimeric antigen receptor T cells (CAR-T) [60]. However, BCSCs themselves hold promise as potential therapeutic cells for targeting breast cancer. Research indicates that BCSCs possess inherent anti-tumor properties, including the expression of various anti-tumor factors and the inhibition of growth factors, which can lead to the induction of apoptosis in tumor cells [58]. Therefore, the extraction and expansion of BCSCs, followed by the optimization of their anti-tumor potency, is a promising approach in breast cancer therapy [61]. Therefore, extracting and expanding BCSCs, concentrating their anti-tumor potency, and transplanting them into patients [62], may become an effective breast cancer treatment (Table 3).
In addition to the specific treatment of BCSCs, the application of stem cells in other breast cancer treatments also has potential value [70].
Stem cell replacement therapy for breast cancer
Breast cancer stem cell replacement therapy is a method to treat breast cancer by using exogenous normal stem cells to replace cancer cells or abnormal stem cells in vivo [71]. There are two main methods of this alternative therapy: one is to transplant the patient’s own stem cells back into the body, and the other is to utilize exogenous stem cells [72]. Transplantation of a patient’s own stem cells can reduce the risk of rejection and infection, but because of the existence of BCSCs, the transplanted stem cells may also be replaced by malignant stem cells. The efficacy of this method is therefore somewhat limited. The application of exogenous stem cells can also effectively replace malignant stem cells in the body [73], but there are also risks of rejection and infection.
At present, stem cell replacement therapy for breast cancer is still in the research stage and has not been widely used in clinical practice [74]. However, with the growth in stem cell research and the continual progress of technology, we expect this method to become an effective treatment for breast cancer in the future [75].
BCSCs have been considered by many researchers to be potential drug carriers. Research shows that BCSCs have the ability to locate tumor tissue selectively and release drugs, thus improving the local concentration and therapeutic effect of the drugs and reducing the damage done to normal cells.
Genetic engineering technology, such as miRNAs, long noncoding RNAs (lncRNAs), and CRISPR-Cas, in which BCSCs are used as drug carriers, can transform drug-sensitive genes in BCSCs, causing them to release specific drugs. In addition, the chemical structure of drugs can be modified to interact better with BCSCs and allow for their release directly into tumor tissue [34] (Fig. 2).
Figure 2: Applications of BCSCs in breast cancer treatment.
The development of stem cell therapy in the context of breast cancer treatment represents a significant advancement in the field, offering promising avenues for targeted intervention and improved patient outcomes. Stem cell therapy, with its ability to specifically target BCSCs, holds immense potential in mitigating tumor growth and recurrence. However, the practical application of stem cell therapy is accompanied by various challenges that necessitate further technological advancements, rigorous clinical trials, and cost-effective strategies to enhance its efficacy and accessibility. One of the primary challenges in the application of stem cell therapy lies in understanding and targeting the specificity of BCSCs. BCSCs possess unique characteristics, including self-renewal capacity and resistance to conventional therapies, which contribute to tumor heterogeneity and recurrence. Therefore, developing therapeutic approaches that specifically target and eliminate BCSCs is crucial for achieving long-term remission and improving patient survival rates. DNA methylation has emerged as a key regulatory mechanism underlying BCSC properties, influencing their self-renewal, differentiation, and response to therapy. Aberrant DNA methylation patterns can lead to the dysregulation of gene expression profiles associated with BCSC phenotypes, thereby driving tumor progression and therapeutic resistance [76].
Moreover, the translation of stem cell therapy from bench to bedside faces hurdles related to technology, clinical trials, and costs. Technological advancements are required to optimize the isolation, expansion, and delivery of therapeutic stem cells, ensuring their efficacy and safety in clinical settings. Additionally, rigorous clinical trials are essential to evaluate the safety, efficacy, and long-term outcomes of stem cell-based therapies in breast cancer patients. These trials should adhere to rigorous ethical standards and regulatory guidelines to safeguard patient welfare and ensure the validity of research findings [76].
Safety is a key issue for breast cancer stem cell therapy. Due to their self-renewal ability and differentiation potential, there are certain risks during the treatment process. Some studies have even shown that stem cell therapy may lead to tumor generation and metastasis [77]. In addition, stem cell therapy may also generate side effects such as immune responses and infections [78]. Therefore, it is necessary to monitor and control the treatment process closely, and strictly screen and identify the source, purity, and quality of stem cells. Furthermore, it is necessary to explore the optimal dosage and protocol for stem cell therapy in order to develop more effective and safe treatment methods [79].
Stem cell therapy requires selecting appropriate cell sources and effectively transferring and injecting them into the patient’s body, while also considering factors such as treatment dose and timing. However, the therapeutic effect of stem cell therapy needs to be further validated in clinical trials [80].
At present, surgical treatment is still the preferred treatment for breast cancer, although with the development of stem cell biology, researchers have found that hematopoietic stem cell transplantation can play an important role in the hematopoietic and immune reconstruction of malignant tumors after high-dose radiation and chemotherapy. Moreover, mesenchymal stem cells can act as gene carriers to inhibit the growth of tumor cells. However, stem cell therapy requires a large amount of human, material, and financial investment, and its high cost so far limits its promotion and popularization in clinical applications.
Breast cancer, a prevalent malignancy in females, presents treatment challenges despite available options like surgery, radiotherapy, chemotherapy, and endocrine therapy. Limitations include post-surgery recurrence, chemotherapy’s toxicity, and side effects. Breast cancer stem cells, pivotal in cancer occurrence, recurrence, and drug resistance, offer a focal point for targeted therapies such as gene therapy, immunotherapy, and cell therapy. Immunotherapy, particularly aimed at breast cancer stem cells, garners significant research attention, albeit facing obstacles like lack of specificity, drug resistance, and safety concerns. Moreover, utilizing breast cancer stem cells as drug carriers shows promise in enhancing treatment precision and efficacy.
The limitation of this review lies in the inherent challenge of translating preclinical findings of BCSC into clinical applications, as many studies are still in the experimental stage and clinical validation is limited. Furthermore, although this review emphasizes the potential of targeting BCSCs, it does not fully address the complexity of the tumor microenvironment and its role in treatment resistance and tumor progression. The variability of BCSC markers in different patients further complicates the development of universal treatment strategies. Potential safety issues, including off-target effects and immune responses, need to be rigorously evaluated through extensive clinical trials to ensure the feasibility and effectiveness of BCSC-targeted therapy.
This review highlights the potential of targeting breast cancer stem cells (BCSCs) to improve breast cancer treatment outcomes. By focusing on their unique properties, novel therapies like immunotherapy, gene therapy, and cell therapy show promise. Further research and clinical trials are essential to validate these approaches and address existing challenges.
Acknowledgement: None.
Funding Statement: This study was supported by Jiangsu Province “333” Project (BRA2020380).
Author Contributions: All authors contributed to this study. Tiansong Xia and Bo Xu conceived and designed the study; Zhizheng Zhang and Tao Li wrote the manuscripts; Yuan Li, Xi Wang, Hao Liu, Xinyu Shen, and Ann Xu revised the manuscript. All authors reviewed the results and approved the final version of the manuscript.
Availability of Data and Materials: No primary data is included in this review.
Ethics Approval: None.
Conflicts of Interest: The authors declare no conflicts of interest to report regarding the present study.
References
1. Bahrami B, Hosseini A, Talei AR, Ghaderi A, Razmkhah M. Adipose derived stem cells exert immunomodulatory effects on natural killer cells in breast cancer. Cell J. 2017;19(1):137–45. [Google Scholar] [PubMed]
2. Balic M, Rapp N, Stanzer S, Lin H, Strutz J, Szkandera J, et al. Novel immunofluorescence protocol for multimarker assessment of putative disseminating breast cancer stem cells. Appl Immunohistochem Mol Morphol. 2011;19(1):33–40. doi:10.1097/PAI.0b013e3181ebf4e8 . [Google Scholar] [PubMed] [CrossRef]
3. Aboulkheyr ESH, Bigdeli B, Zhand S, Aref AR, Thiery JP, Warkiani ME. Mesenchymal stem cells induce PD-L1 expression through the secretion of CCL5 in breast cancer cells. J Cell Physiol. 2021;236(5):3918–28. doi:10.1002/jcp.30135 . [Google Scholar] [PubMed] [CrossRef]
4. Acuna RA, Varas-Godoy M, Herrera-Sepulveda D, Retamal MA. Connexin46 expression enhances cancer stem cell and epithelial-to-mesenchymal transition characteristics of human breast cancer MCF-7 cells. Int J Mol Sci. 2021;22(22):12604. doi:10.3390/ijms222212604 . [Google Scholar] [PubMed] [CrossRef]
5. Agur Z, Kirnasovsky OU, Vasserman G, Tencer-Hershkowicz L, Kogan Y, Harrison H, et al. Dickkopf1 regulates fate decision and drives breast cancer stem cells to differentiation: an experimentally supported mathematical model. PLoS One. 2011;6(9):e24225. doi:10.1371/journal.pone.0024225 . [Google Scholar] [PubMed] [CrossRef]
6. Ahmad A, Mondal SK, Mukhopadhyay D, Banerjee R, Alkharfy KM. Development of liposomal formulation for delivering anticancer drug to breast cancer stem-cell-like cells and its pharmacokinetics in an animal model. Mol Pharm. 2016;13(3):1081–8. doi:10.1021/acs.molpharmaceut.5b00900 . [Google Scholar] [PubMed] [CrossRef]
7. Albarenque SM, Zwacka RM, Mohr A. Both human and mouse mesenchymal stem cells promote breast cancer metastasis. Stem Cell Res. 2011;7(2):163–71. doi:10.1016/j.scr.2011.05.002 . [Google Scholar] [PubMed] [CrossRef]
8. Al-Ejeh F, Smart CE, Morrison BJ, Chenevix-Trench G, Lopez JA, Lakhani SR, et al. Breast cancer stem cells: treatment resistance and therapeutic opportunities. Carcinogenesis. 2011;32(5):650–8. doi:10.1093/carcin/bgr028 . [Google Scholar] [PubMed] [CrossRef]
9. Alraouji NN, Hendrayani SF, Ghebeh H, Al-Mohanna FH, Aboussekhra A. Osteoprotegerin (OPG) mediates the anti-carcinogenic effects of normal breast fibroblasts and targets cancer stem cells through inhibition of the β-catenin pathway. Cancer Lett. 2021;520:374–84. doi:10.1016/j.canlet.2021.08.013 . [Google Scholar] [PubMed] [CrossRef]
10. Alraouji NN, Al-Mohanna FH, Ghebeh H, Arafah M, Almeer R, Al-Tweigeri T, et al. Tocilizumab potentiates cisplatin cytotoxicity and targets cancer stem cells in triple-negative breast cancer. Mol Carcinog. 2020;59(9):1041–51. doi:10.1002/mc.23234 . [Google Scholar] [PubMed] [CrossRef]
11. Anwar TE, Kleer CG. Tissue-based identification of stem cells and epithelial-to-mesenchymal transition in breast cancer. Hum Pathol. 2013;44(8):1457–64. doi:10.1016/j.humpath.2013.01.005 . [Google Scholar] [PubMed] [CrossRef]
12. An H, Kim JY, Lee N, Cho Y, Oh E, Seo JH. Salinomycin possesses anti-tumor activity and inhibits breast cancer stem-like cells via an apoptosis-independent pathway. Biochem Biophys Res Commun. 2015;466(4):696–703. doi:10.1016/j.bbrc.2015.09.108 . [Google Scholar] [PubMed] [CrossRef]
13. Bano A, Stevens JH, Modi PS, Gustafsson JA, Strom AM. Estrogen receptor β4 regulates chemotherapy resistance and induces cancer stem cells in triple negative breast cancer. Int J Mol Sci. 2023;24(6):5867. doi:10.3390/ijms24065867 . [Google Scholar] [PubMed] [CrossRef]
14. Bhattacharya A, Mukherjee S, Khan P, Banerjee S, Dutta A, Banerjee N, et al. SMAR1 repression by pluripotency factors and consequent chemoresistance in breast cancer stem-like cells is reversed by aspirin. Sci Signal. 2020;13(654):eaay6077. doi:10.1126/scisignal.aay6077 . [Google Scholar] [PubMed] [CrossRef]
15. Bhuvanalakshmi G, Basappa, Rangappa KS, Dharmarajan A, Sethi G, Kumar AP, et al. Breast cancer stem-like cells are inhibited by diosgenin, a steroidal saponin, by the attenuation of the Wnt β-catenin signaling via the Wnt antagonist secreted frizzled related protein-4. Front Pharmacol. 2017;8:124. doi:10.3389/fphar.2017.00124 . [Google Scholar] [PubMed] [CrossRef]
16. Boo L, Ho WY, Ali NM, Yeap SK, Ky H, Chan KG, et al. MiRNA transcriptome profiling of spheroid-enriched cells with cancer stem cell properties in human breast MCF-7 cell line. Int J Biol Sci. 2016;12(4):427–45. [Google Scholar] [PubMed]
17. Borzone FR, Giorello MB, Sanmartin MC, Yannarelli G, Martinez LM, Chasseing NA. Mesenchymal stem cells and cancer-associated fibroblasts as a therapeutic strategy for breast cancer. Br J Pharmacol. 2024;181(2):238–56. [Google Scholar] [PubMed]
18. Bose B, Sen U, Shenoy PS. Breast cancer stem cell therapeutics, multiple strategies versus using engineered mesenchymal stem cells with notch inhibitory properties: possibilities and perspectives. J Cell Biochem. 2018;119(1):141–9. [Google Scholar] [PubMed]
19. Liang YJ, Ding Y, Levery SB, Lobaton M, Handa K, Hakomori SI. Differential expression profiles of glycosphingolipids in human breast cancer stem cells vs. cancer non-stem cells. Proc Natl Acad Sci U S A. 2013;110(13):4968–73. [Google Scholar] [PubMed]
20. Montales MT, Rahal OM, Kang J, Rogers TJ, Prior RL, Wu X, et al. Repression of mammosphere formation of human breast cancer cells by soy isoflavone genistein and blueberry polyphenolic acids suggests diet-mediated targeting of cancer stem-like/progenitor cells. Carcinogenesis. 2012;33(3):652–60. [Google Scholar] [PubMed]
21. Nahas GR, Patel SA, Bliss SA, Rameshwar P. Can breast cancer stem cells evade the immune system? Curr Med Chem. 2012;19(35):6036–49. doi:10.2174/0929867311209066036 . [Google Scholar] [CrossRef]
22. Nguyen M, Osipo C. Targeting breast cancer stem cells using naturally occurring phytoestrogens. Int J Mol Sci. 2022;23(12):6813. doi:10.3390/ijms23126813 . [Google Scholar] [PubMed] [CrossRef]
23. Muntimadugu E, Kumar R, Saladi S, Rafeeqi TA, Khan W. CD44 targeted chemotherapy for co-eradication of breast cancer stem cells and cancer cells using polymeric nanoparticles of salinomycin and paclitaxel. Colloids Surf B Biointerfaces. 2016;143:532–46. doi:10.1016/j.colsurfb.2016.03.075 . [Google Scholar] [PubMed] [CrossRef]
24. Liu YY, Gu RJ, Gao MR, Wei YW, Shi Y, Wang X, et al. Emerging role of substance and energy metabolism associated with neuroendocrine regulation in tumor cells. Front Endocrinol. 2023;14:1126271. doi:10.3389/fendo.2023.1126271 . [Google Scholar] [PubMed] [CrossRef]
25. Moradi-Gharibvand N, Setayeshmehr M, Kazemi M, Safaee A, Khorsandi LS, Nejad DB, et al. Pomegranate seed extract enhances the inhibitory effect of adipose-derived mesenchymal stem cells on breast cancer cell line in co-culture conditions. Res Pharm Sci. 2022;17(4):372–82. doi:10.4103/1735-5362.350238 . [Google Scholar] [PubMed] [CrossRef]
26. Velasco-Velazquez MA, Homsi N, De La Fuente M, Pestell RG. Breast cancer stem cells. Int J Biochem Cell Biol. 2012;44(4):573–7. [Google Scholar] [PubMed]
27. Wang B, Xi C, Liu M, Sun H, Liu S, Song L, et al. Breast fibroblasts in both cancer and normal tissues induce phenotypic transformation of breast cancer stem cells: a preliminary study. PeerJ. 2018;6:e4805. [Google Scholar] [PubMed]
28. Jiao F, Hu H, Han T, Yuan C, Wang L, Jin Z, et al. Long noncoding RNA MALAT-1 enhances stem cell-like phenotypes in pancreatic cancer cells. Int J Mol Sci. 2015;16(4):6677–93. [Google Scholar] [PubMed]
29. Wang D, Lu P, Zhang H, Luo M, Zhang X, Wei X, et al. Correction: oct-4 and Nanog promote the epithelial-mesenchymal transition of breast cancer stem cells and are associated with poor prognosis in breast cancer patients. Oncotarget. 2021;12(10):1024–5. [Google Scholar] [PubMed]
30. Wang L, He M, Fu L, Jin Y. Exosomal release of microRNA-454 by breast cancer cells sustains biological properties of cancer stem cells via the PRRT2/Wnt axis in ovarian cancer. Life Sci. 2020;257:118024. [Google Scholar] [PubMed]
31. Wu M, Zhang X, Zhang W, Yan L, Liu X, Zhang M, et al. Paracrine secretion of IL8 by breast cancer stem cells promotes therapeutic resistance and metastasis of the bulk tumor cells. Cell Commun Signal. 2023;21(1):59. doi:10.1186/s12964-023-01068-6 . [Google Scholar] [PubMed] [CrossRef]
32. Xun J, Wang D, Shen L, Gong J, Gao R, Du L, et al. JMJD3 suppresses stem cell-like characteristics in breast cancer cells by downregulation of Oct4 independently of its demethylase activity. Oncotarget. 2017;8(13):21918–29. doi:10.18632/oncotarget.15747 . [Google Scholar] [PubMed] [CrossRef]
33. Yan X, Yu Y, Li L, Chen N, Song W, He H, et al. Friend leukemia virus integration 1 is a predictor of poor prognosis of breast cancer and promotes metastasis and cancer stem cell properties of breast cancer cells. Cancer Med. 2018;7(8):3548–60. doi:10.1002/cam4.1589 . [Google Scholar] [PubMed] [CrossRef]
34. Yang N, Wang C, Wang Z, Zona S, Lin SX, Wang X, et al. FOXM1 recruits nuclear Aurora kinase A to participate in a positive feedback loop essential for the self-renewal of breast cancer stem cells. Oncogene. 2017;36(24):3428–40. doi:10.1038/onc.2016.490 . [Google Scholar] [PubMed] [CrossRef]
35. Buhler H, Hoberg C, Fakhrian K, Adamietz IA. Zoledronic acid inhibits the motility of cancer stem-like cells from the human breast cancer cell line MDA-MB 231. In Vivo. 2016;30(6):761–8. doi:10.21873/invivo.10992 . [Google Scholar] [PubMed] [CrossRef]
36. Cabuk D, Yetimoglu E, Simsek T, Gacar G, Subasi C, Canturk Z, et al. The distribution of CD44+/CD24- cancer stem cells in breast cancer and its relationship with prognostic factors. J BUON. 2016;21(5):1121–8. [Google Scholar] [PubMed]
37. Cao S, Guo J, He Y, Alahdal M, Tang S, Zhao Y, et al. Nano-loaded human umbilical cord mesenchymal stem cells as targeted carriers of doxorubicin for breast cancer therapy. Artif Cells Nanomed Biotechnol. 2018;46(sup1):642–52. doi:10.1080/21691401.2018.1434185 . [Google Scholar] [PubMed] [CrossRef]
38. Germain AR, Carmody LC, Morgan B, Fernandez C, Forbeck E, Lewis TA, et al. Identification of a selective small molecule inhibitor of breast cancer stem cells. Bioorg Med Chem Lett. 2012;22(10):3571–4. doi:10.1016/j.bmcl.2012.01.035 . [Google Scholar] [PubMed] [CrossRef]
39. Carpenter RL, Sirkisoon S, Zhu D, Rimkus T, Harrison A, Anderson A, et al. Combined inhibition of AKT and HSF1 suppresses breast cancer stem cells and tumor growth. Oncotarget. 2017;8(43):73947–63. doi:10.18632/oncotarget.18166 . [Google Scholar] [PubMed] [CrossRef]
40. Castiello L, Sestili P, Schiavoni G, Dattilo R, Monque DM, Ciaffoni F, et al. Disruption of IFN-I signaling promotes HER2/Neu tumor progression and breast cancer stem cells. Cancer Immunol Res. 2018;6(6):658–70. doi:10.1158/2326-6066.CIR-17-0675. [Google Scholar] [PubMed] [CrossRef]
41. Falvo P, Orecchioni S, Hillje R, Raveane A, Mancuso P, Camisaschi C, et al. Cyclophosphamide and vinorelbine activate stem-like CD8+ T cells and improve anti-PD-1 efficacy in triple-negative breast cancer. Cancer Res. 2021;81(3):685–97. [Google Scholar] [PubMed]
42. Peng W, Chang L, Li W, Liu Y, Zhang M. OCT4 and SOX2 specific cytotoxic T cells exhibit not only good efficiency but also Synergize PD-1 inhibitor (Nivolumab) in treating breast cancer stem-like cells and drug-resistant breast cancer mice. Front Oncol. 2022;12:781093. [Google Scholar] [PubMed]
43. Zhang H, Lu H, Xiang L, Bullen JW, Zhang C, Samanta D, et al. HIF-1 regulates CD47 expression in breast cancer cells to promote evasion of phagocytosis and maintenance of cancer stem cells. Proc Natl Acad Sci USA. 2015;112(45):E6215–23. [Google Scholar] [PubMed]
44. Tan W, Tang H, Jiang X, Ye F, Huang L, Shi D, et al. Metformin mediates induction of miR-708 to inhibit self-renewal and chemoresistance of breast cancer stem cells through targeting CD47. J Cell Mol Med. 2019;23(9):5994–6004. [Google Scholar] [PubMed]
45. Han X, Saengow C, Ju L, Ren W, Ewoldt RH, Irudayaraj J. Exosome-coated oxygen nanobubble-laden hydrogel augments intracellular delivery of exosomes for enhanced wound healing. Nat Commun. 2024;15(1):3435. [Google Scholar] [PubMed]
46. Gaio E, Conte C, Esposito D, Reddi E, Quaglia F, Moret F. CD44 targeting mediated by polymeric nanoparticles and combination of Chlorine TPCS2a-PDT and Docetaxel-chemotherapy for efficient killing of breast differentiated and stem cancer cells in vitro. Cancers. 2020;12(2):278. [Google Scholar] [PubMed]
47. Ithimakin S, Day KC, Malik F, Zen Q, Dawsey SJ, Bersano-Begey TF, et al. HER2 drives luminal breast cancer stem cells in the absence of HER2 amplification: implications for efficacy of adjuvant trastuzumab. Cancer Res. 2013;73(5):1635–46. [Google Scholar] [PubMed]
48. Chaudhary P, Yadav K, Lee HJ, Kang KW, Mo J, Kim JA. siRNA treatment targeting integrin α11 overexpressed via EZH2-driven axis inhibits drug-resistant breast cancer progression. Breast Cancer Res. 2024;26(1):72. [Google Scholar] [PubMed]
49. D’Angelo RC, Ouzounova M, Davis A, Choi D, Tchuenkam SM, Kim G, et al. Notch reporter activity in breast cancer cell lines identifies a subset of cells with stem cell activity. Mol Cancer Ther. 2015;14(3):779–87. [Google Scholar] [PubMed]
50. Lamb R, Ablett MP, Spence K, Landberg G, Sims AH, Clarke RB. Wnt pathway activity in breast cancer sub-types and stem-like cells. PLoS One. 2013;8(7):e67811. [Google Scholar] [PubMed]
51. Yi H, Wu M, Zhang Q, Lu L, Yao H, Chen S, et al. Reversal of HER2 negativity: an unexpected role for lovastatin in triple-negative breast cancer stem cells. J Cancer. 2020;11(13):3713–6. [Google Scholar] [PubMed]
52. Ahn YT, Kim MS, Kim YS, An WG. Astaxanthin reduces stemness markers in BT20 and T47D breast cancer stem cells by inhibiting expression of pontin and mutant p53. Mar Drugs. 2020;18(11):577. [Google Scholar] [PubMed]
53. Ashour F, Awwad MH, Sharawy HEL, Kamal M. Estrogen receptor positive breast tumors resist chemotherapy by the overexpression of p53 in cancer stem cells. J Egypt Natl Canc Inst. 2018;30(2):45–8. [Google Scholar] [PubMed]
54. Hermawan A, Ikawati M, Khumaira A, Putri H, Jenie RI, Angraini SM, et al. Bioinformatics and in vitro studies reveal the importance of p53, PPARG and notch signaling pathway in inhibition of breast cancer stem cells by hesperetin. Adv Pharm Bull. 2021;11(2):351–60. [Google Scholar] [PubMed]
55. Inoue S, Imanishi M, Kanzaki A, Fujimoto A, Maeyama M, Okamoto A, et al. Role of cancer stem-like cells in the process of invasion and mesenchymal transformation by a reconstituted triple-negative breast cancer cell population resistant to p53-induced apoptosis. Acta Histochem Cytochem. 2022;55(5):169–84. [Google Scholar] [PubMed]
56. Monchusi B, Kaur M. miRNAs as modulators of cholesterol in breast cancer stem cells: an approach to overcome drug resistance in cancer. Curr Drug Targets. 2022;23(6):656–77. [Google Scholar] [PubMed]
57. Lu H, Lyu Y, Tran L, Lan J, Xie Y, Yang Y, et al. HIF-1 recruits NANOG as a coactivator for TERT gene transcription in hypoxic breast cancer stem cells. Cell Rep. 2021;36(13):109757. [Google Scholar] [PubMed]
58. Azzoni V, Wicinski J, Macario M, Castagne M, Finetti P, Ambrosova K, et al. BMI1 nuclear location is critical for RAD51-dependent response to replication stress and drives chemoresistance in breast cancer stem cells. Cell Death Dis. 2022;13(2):96. [Google Scholar] [PubMed]
59. Knopik-Skrocka A, Sempowicz A, Piwocka O. Plasticity and resistance of cancer stem cells as a challenge for innovative anticancer therapies-do we know enough to overcome this? Excli J. 2024;23:335–55. [Google Scholar] [PubMed]
60. Pai CP, Wang H, Seachrist DD, Agarwal N, Adams JA, Liu Z, et al. The PML1-WDR5 axis regulates H3K4me3 marks and promotes stemness of estrogen receptor-positive breast cancer. Cell Death Differ. 2024;31(6):768–78. [Google Scholar] [PubMed]
61. Tang W, Wu Y, Qi X, Yu R, Lu Z, Chen A, et al. PGK1-coupled HSP90 stabilizes GSK3β expression to regulate the stemness of breast cancer stem cells. Cancer Biol Med. 2021;19(4):486–503. [Google Scholar] [PubMed]
62. Taurin S, Alkhalifa H. Breast cancers, mammary stem cells, and cancer stem cells, characteristics, and hypotheses. Neoplasia. 2020;22(12):663–78. [Google Scholar] [PubMed]
63. Banerjee S, Norman DD, Lee SC, Parrill AL, Pham TC, Baker DL, et al. Highly potent non-carboxylic acid autotaxin inhibitors reduce melanoma metastasis and chemotherapeutic resistance of breast cancer stem cells. J Med Chem. 2017;60(4):1309–24. [Google Scholar] [PubMed]
64. Brugnoli F, Grassilli S, Al-Qassab Y, Capitani S, Bertagnolo V. CD133 in breast cancer cells: more than a stem cell marker. J Oncol. 2019;2019:7512632. [Google Scholar] [PubMed]
65. Tay ASS, Amano T, Edwards LA, Yu JS. CD133 mRNA-transfected dendritic cells induce coordinated cytotoxic and helper T cell responses against breast cancer stem cells. Mol Ther Oncolytics. 2021;22:64–71. [Google Scholar] [PubMed]
66. Grant CV, Cai S, Risinger AL, Liang H, O’Keefe BR, Doench JG, et al. CRISPR-Cas9 genome-wide knockout screen identifies mechanism of selective activity of dehydrofalcarinol in mesenchymal stem-like triple-negative breast cancer cells. J Nat Prod. 2020;83(10):3080–92. [Google Scholar] [PubMed]
67. Zhao R, Kaakati R, Liu X, Xu L, Lee AK, Bachelder R, et al. CRISPR/Cas9-mediated BRCA1 knockdown adipose stem cells promote breast cancer progression. Plast Reconstr Surg. 2019;143(3):747–56. [Google Scholar] [PubMed]
68. Jang JY, Kim MK, Jeon YK, Joung YK, Park KD, Kim CW. Adenovirus adenine nucleotide translocator-2 shRNA effectively induces apoptosis and enhances chemosensitivity by the down-regulation of ABCG2 in breast cancer stem-like cells. Exp Mol Med. 2012;44(4):251–9. [Google Scholar] [PubMed]
69. Ryspayeva DE, Smolanka II, Dudnichenko capital AC, Lyashenko AA, Grinevich YA, Gurianov VG, et al. Are CD44+/CD24- cells the assumed cancer stem cells in breast cancer? Exp Oncol. 2017;39(3):224–8. [Google Scholar] [PubMed]
70. Yeh WL, Tsai CF, Chen DR. Peri-foci adipose-derived stem cells promote chemoresistance in breast cancer. Stem Cell Res Ther. 2017;8(1):177. [Google Scholar] [PubMed]
71. Yin S, Cheryan VT, Xu L, Rishi AK, Reddy KB. Myc mediates cancer stem-like cells and EMT changes in triple negative breast cancers cells. PLoS One. 2017;12(8):e0183578. [Google Scholar] [PubMed]
72. Li G, Wang D, Ma W, An K, Liu Z, Wang X, et al. Transcriptomic and epigenetic analysis of breast cancer stem cells. Epigenomics. 2018;10(6):765–83. [Google Scholar] [PubMed]
73. Li SY, Sun R, Wang HX, Shen S, Liu Y, Du XJ, et al. Combination therapy with epigenetic-targeted and chemotherapeutic drugs delivered by nanoparticles to enhance the chemotherapy response and overcome resistance by breast cancer stem cells. J Control Release. 2015;205:7–14. [Google Scholar] [PubMed]
74. Li J, Liu R, Yang Y, Huang Y, Li X, Liu R, et al. Triptolide-induced in vitro and in vivo cytotoxicity in human breast cancer stem cells and primary breast cancer cells. Oncol Rep. 2014;31(5):2181–6. [Google Scholar] [PubMed]
75. Li X, Pan YZ, Seigel GM, Hu ZH, Huang M, Yu AM. Breast cancer resistance protein BCRP/ABCG2 regulatory microRNAs (hsa-miR-328, -519c and -520h) and their differential expression in stem-like ABCG2+ cancer cells. Biochem Pharmacol. 2011;81(6):783–92. [Google Scholar] [PubMed]
76. De Angelis ML, Francescangeli F, Zeuner A. Breast cancer stem cells as drivers of tumor chemoresistance, dormancy and relapse: new challenges and therapeutic opportunities. Cancers. 2019;11(10):1569. [Google Scholar] [PubMed]
77. Di Lisa D, Cortese K, Chiappalone M, Arnaldi P, Martinoia S, Castagnola P, et al. Electrophysiological and morphological modulation of neuronal-glial network by breast cancer and nontumorigenic mammary cell conditioned medium. Front Bioeng Biotechnol. 2024;12:1368851. [Google Scholar] [PubMed]
78. Lee KL, Chen G, Chen TY, Kuo YC, Su YK. Effects of cancer stem cells in triple-negative breast cancer and brain metastasis: challenges and solutions. Cancers. 2020;12(8):2122. [Google Scholar] [PubMed]
79. Tang H, Liang T, Zhou Y, Ju H, Song D, Fang H. Telocytes reduce oxidative stress by downregulating DUOX2 expression in inflamed lungs of mice. Acta Biochim Biophys Sin. 2022;54(4):574–82. [Google Scholar] [PubMed]
80. Saeg F, Anbalagan M. Breast cancer stem cells and the challenges of eradication: a review of novel therapies. Stem Cell Investig. 2018;5:39. [Google Scholar] [PubMed]
Cite This Article
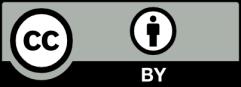
This work is licensed under a Creative Commons Attribution 4.0 International License , which permits unrestricted use, distribution, and reproduction in any medium, provided the original work is properly cited.