Open Access
REVIEW
Immunomodulatory and chemopreventive effects of resveratrol on the digestive system cancers
Laboratory for Immunology and Hematology Research, Rabin Medical Center, Hasharon Hospital, Petah-Tiqva, the Sackler School of Medicine, Tel-Aviv University, Ramat Aviv, 69978, Israel
* Corresponding Author: MEIR DJALDETTI. Email:
Oncology Research 2024, 32(9), 1389-1399. https://doi.org/10.32604/or.2024.049745
Received 17 January 2024; Accepted 23 May 2024; Issue published 23 August 2024
Abstract
Resveratrol (RSV), the primary polyphenol found in grapes, has been revealed to have anti-inflammatory properties by reducing the capacity of the peripheral blood mononuclear cells to produce pro-inflammatory cytokines, including IL-1β, IL-6, IL-1ra and TNFα. Considering the close association between chronic inflammation and cancer development, RSV’s immunomodulatory properties are one way by which the polyphenol may inhibit cancer initiation, proliferation, neovascularization, and migration. Resveratrol influences the generation of microtumor environment which is one of the key factors in cancer progress. In addition to immunomodulation, RSV inhibits cancer development by expressing anti-oxidant effects, causing cell cycle arrest, stimulating the function of certain enzymes, and activating cell signaling pathways. The end outcome is one of the various forms of cell death, including apoptosis, pyroptosis, necroptosis, and more, as it has been observed in vitro. RSV has been shown to act against cancer in practically every organ, while its effects on colon cancer have been documented more frequently. It is remarkable that longer-term clinical studies that may have established the potential for this natural substance to serve as a therapeutic adjuvant to traditional anti-cancer medications were not prompted by the encouraging outcomes seen with cancer cells treated with non-toxic doses of resveratrol. The current review aims to assess the recent findings about the immunological and anti-cancer characteristics of RSV, with a particular emphasis on cancers of the digestive tract, as a challenge for future clinical research that may contribute to the better prognosis of cancer.Graphic Abstract
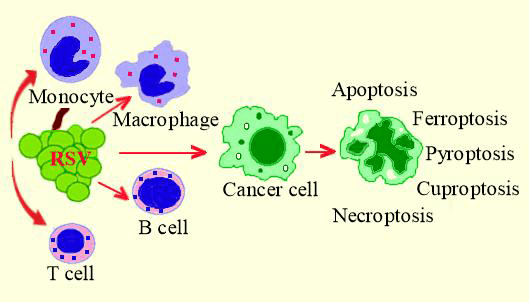
Keywords
The history of grape cultivation is estimated to be 6,000 to 8,000 old, based on archeological and seed findings [1]. The dual utilization of grapes as a delicious fruit and as a source for extensive vine production explains their widespread farming and development. Leaving apart the high commercial value of the grapes in the nutritional and vine industry, grapes consumption is associated with beneficial health virtues. Studies have shown that grapes feeding diminishes the risk of cardiovascular and skin diseases, diabetes, neurodegeneration, Alzheimer disease, obesity, and certain types of cancers [2–4]. These effects result from grapes’ high polyphenol content, of which RSV is the most significant. Plants that produced RSV were significant items in the Asian pharmacopeia, according to Venkat et al. [5].
RSV has demonstrated anti-inflammatory properties by mitigating the harmful effects of reactive oxygen species (ROS), primarily hydrogen peroxide and superoxide radicals [6]. For middle-aged and older people with increased oxidative and anti-inflammatory activity, RSV plays an important role. It was shown that treatment of their peripheral blood mononuclear cells (PBMC) with RSV regulated the production of the pro-inflammatory cytokines IL-6 and TNFα [7]. RSV inhibits the activity of immune recognition receptors (TLR), such as TLR4, which stimulates the inflammatory mediators nuclear factor kappa B (NF-kB) and interferon (IFN) [8]. Given the proven impact of chronic inflammation on the onset and progression of cancer [9,10] and the immune system’s active participation in this process, it is plausible that the immunomodulatory properties of polyphenols play a significant role in cancer prevention. Inflammatory reactions are heightened by malignant processes in general and digestive tract malignancies in particular. This, in turn, activates immune system components such as PBMC, initiating a cross-talk between immune and cancer cells [11].
A comprehensive review of RSV pharmacokinetics has been conducted by Neves et al. [12]. Given orally it is readily absorbed, however, each person’s absorption is different. In the digestive system, it is converted by the intestinal and liver cells into glucuronic acid and sulfate coupled with the phenol clusters and rapidly excreted through the feces and urine suggesting a low bioavailability. To avoid this impediment, efforts have been made to provide its consistent delivery using RSV-loaded nanoparticles which showed encouraging outcomes by inhibition of colon cancer cell migration and triggering apoptosis [13].
RSV may induce cancer cell death through a variety of mechanisms such as apoptosis, pyroptosis, ferroptosis, cuproptosis, necrosis, and more [14]. With an emphasis on digestive tract cancers, the current work aimed to review the immunomodulatory and carcinopreventive effects of RSV in connection to the beneficial effect of polyphenols on the prevention of tumor development.
Resveratrol as an Immunomodulator
There is sound evidence that RSV exerts immuno-regulatory functions. RSV reduces the immune cell expression of pro-inflammatory cytokines by inhibiting of toll-like receptor (TLR) [15]. It regulates the immune properties of lymphocyte subtypes having a crucial role in the process of inflammation [16]. RSV induces polarization and immune response of CD8+T lymphocytes and the production of cancer preventing IFNγ and TNFα cytokines [17]. Acting on CD8+T cells by upregulating the levels of TNFα, IL-2, and IL-12, RSV enhanced their cytotoxic effect, an important step in halting the growth of cancer [17–20]. By upregulating protein kinase B (Akt B) and the mammalian rapamycin target (mTORC2) pathway, RSV enhanced the function of the natural killer (NK) cells that actively participate in the process of carcinoprevention [21].
After being treated with RSV, neutrophils, and macrophages exhibited a concentration-dependent reduction of ROS generation [22]. When unstimulated human PBMC were incubated with RSV, the production of IL-6, IL-1ra, and IL-10 was lowered, while TNFα release was slightly elevated [23]. Conversely, exposing unstimulated RAW-264.7 macrophages to RSV increased IL-10 release and decreased TNFα production [24], indicating that the polyphenol has distinct effects on various macrophage subtypes. When human THP-1 monocytes and macrophages were treated with RSV, a difference in the immune response was seen; although the polyphenol stimulated the pro-inflammatory cascade and inhibited monocyte proliferation by causing cell cycle arrest in the S phase, it also produced an anti-inflammatory burst in THP-1 macrophages [25].
The pro-inflammatory cytokines IL-6 and TNFα were significantly inhibited when lipopolysaccharide (LPS) stimulated macrophages were treated with RSV [26], whereas the generation of the anti-inflammatory cytokines IL-10 and TGF-β was enhanced [27]. Further explanations for the anti-inflammatory activity of RSV treated macrophages include inhibition of NF-kB, Janus kinase, and signal transducers and transcription activators (JAK/STAT), as well as inhibition of IL-6 and TNFα secretion by increased production of cytokine signaling suppressor 1 (SOCS1), being a STAT inhibitor [28,29]. These findings are consistent with the decreased generation of inflammatory mediators achieved with RSV, such as TNFα, IL-8, and monocyte chemoattractant protein-1 (MCP-1) through activation of NF-kB, JAK/STAT, and Janus kinase in LPS stimulated monocytes [30].
Inhibition of vascular endothelial growth factor suppressor (miR-146a), activation of nuclear relation factor 2 (Nrf2), and suppression of hypoxia-inducible factor 1α (HIF-1α) are additional mechanisms to account for the anti-inflammatory activity of LPS stimulated RAW 264.7 macrophages exposed to RSV [31,32]. RSV affects M1/M2 macrophage polarization resulting in the inhibition of pro-inflammatory cytokine production and amplification of the anti-inflammatory ones [17,33,34] in part via adenosine monophosphate-activated protein kinase (AMPK) activation [35]. RSV suppresses the function of the pro-inflammatory M1 macrophages by activation of poly (ADP-ribose) polymerase (PARP), an effect amplified by the addition of nicotinamide [36]. These and other investigations provide strong evidence for RSV’s immunomodulatory function as a carcinopreventer. Fig. 1 illustrates the way RSV induces PBMC to produce anti-inflammatory cytokines that affect cancer cells and the tumor microenvironment.
Figure 1: Peripheral blood mononuclear cells are stimulated by resveratrol to release anti-inflammatory cytokines, which subsequently prevent chronic inflammation and the growth of cancer cells. M-monocytes and macrophages, L-large lymphocytes, SL-small lymphocytes, and asterisks denote anti-inflammatory cytokines.
Resveratrol as Cancer Suppressor
A considerable number of studies suggest that RSV functions as a suppressor of cancer cell proliferation and has the potential to prevent their spreading to practically all organs [37,38]. Regrettably, while most of the studies have been carried out in vitro or with animal models using various types of cancer cells, clinical research has been conducted mostly on patients with colorectal cancer [39]. In addition to immunomodulatory activity, RSV prevents cancer development by affecting tumor microenvironment and inactivating essential pathways such as NF-kB, the mitogen-activated protein kinase (MAPK), and JAK/STAT that are essential for cancer cells’ development [40–42]. Inhibition of ROS species and pSTAT-3 expression in the gut microbiota by RSV play an important role in carcinoprevention [43].
Cancer development is closely associated with the function of tumor-associated macrophages (TAM). It has been reported that the M2 TAM polarization was affected by RSV due to a significant decrease in STAT3 activity, an effect observed both in lung cancer cells and in a mouse lung cancer model [44]. Promoting M1 to M2 macrophage polarization, lesser production of IL-6, and suppression of STAT3 pathway by RSV was observed in breast cancer cells [45]. Enhanced chemosensitivity, as well as inhibited differentiation, migration, and vascular endothelial growth factor (VEGF) production, were also the result of activated M2 polarization when tumor cells were incubated with RSV, or with the conditioned medium obtained after incubation of M2 macrophages treated with the polyphenol [46]. Additional factors such as angiogenesis, epithelial-mesenchymal transition, and repressing the functions of cancer stem cells targeted by RSV contribute to preventing cancer development and metastasis [47].
Overcoming cancer cells’ radioresistance is another benefit granting RSV the role as an adjuvant to radiotherapy [48]. Moreover, it has been demonstrated that RSV increases the susceptibility of breast cancer cells to talazoparib by simultaneously inhibiting Akt signaling and increasing autophagy [49]. RSV may induce programed cancer cells’ death in 11 different ways [50], the most explored being autophagy, apoptosis, ferroptosis, pyroptosis, cuproptosis, and necroptosis [51] schematically presented in Fig. 2.
Figure 2: A schematic presentation of some of the mechanisms by which resveratrol may induce cancer cells’ death. The knowledge about the impact of RSV on cuproptosis is limited, as shown by the question mark. Ca cell-cancer cell.
Autophagy is a process by which RSV may inhibit cancer cell proliferation and metastasis [52], acting through a number of autophagic signaling pathways [53]. Sirtuin 3 (SIRT3), the protein that maintains mitochondrial homeostasis plays an important role in the regulation of autophagy, a process promoted by RSV in 4T1 breast cancer cells by upregulation of the SIRT3/AMPK autophagy pathway [54]. However, in certain types of cancer, SIRT3 may function as a tumor promoter [55]. Activation of the tumor suppressor nerve growth factor receptor (NGFR-AMPK-mTOR) pathway by RSV promoted both autophagy and apoptosis in A549 non-small lung cancer cells [56]. Restraint of p62 autophagy suppressor and mTOR activation is an additional mechanism by which RSV inhibits cancer progression [57,58]. Autophagy in human leukemia HL-60 cells induced by RSV was found to be dependent on a serine-threonine kinase (LKB1)-AMK and phosphatidylinositol-3-kinase (PI3K)/AKT-regulated mTOR pathways [59].
Apoptosis plays a key role in cancer cells’ death. Depending on the cancer cell type RSV may induce apoptosis triggering a chain reaction by activating AMPK. Consequently, it promotes enhancement of the tumor suppressor TSC2 that inhibits the anti-apoptotic activity of mTOR. Stimulation of apoptotic receptors such as ROS and activation of P53 are additional mechanisms by which RSV sets off the procession of apoptosis [60]. Treating HCT-116WT colorectal cancer cells with RSV at doses >10 µM induced apoptosis by inhibition of the tumor promoter SIRT-1, increasing the expression of P21, P53, and the Bcl-2-associated x protein (Bax-2) [61]. Increased caspase activity and DNA breakdown induced by RSV lead to apoptosis expressed by condensation of nuclear chromatin and nuclear damage [62]. Tumor long non-coding RNAs linked with cell proliferation, spreading, and metastasis are also targeted by RSV [63]. Applied in combination with anti-cancer drugs, RSV increases cancer cells’ chemosensitivity by boosting apoptosis [64].
Ferroptosis, another mode of programmed cell death may be triggered by natural polyphenols including RSV [65]. The primary mechanism leading to ferroptosis is intracellular iron accumulation causing cell damage and death. The main ferroptosis regulators are amino acid transporters that exchange cysteine uptake for glutamate (SLC7A11), glutathione peroxidase 4 (GPX4), p53, NRF2, the heat shock proteins HSPB1, CISD1, the protein-coding FANCD2 and the converter of long-chain fatty acids and coenzyme A into fatty-acid coenzymes (ACSL4) [66,67]. RSV has the potential to cause ferroptosis in colorectal cancer cells through upregulating the formation of glutathione reductase 4 (GPX4), decreasing the expression of the cystine transporter solute carrier family 7 member 11 (SLC7A11), and increasing ROS production and lipid peroxidation [68]. Notably, RSV can enhance ferroptosis by acting as a SIRT inducer [69]. Therefore, activation of ferroptosis by RSV may serve as an additional adjuvant for the therapy of many diseases including cancer prevention.
Pyroptosis is an alternative mechanism of cell death that is seen in various illnesses, including cancer. The role of pyroptosis in cancer cell death initiated extensive research. The process impacts the proliferation, invasion, and metastasis of cancer cells and is triggered by inflammasomes, gasdermin D cleavage, and elevated IL-18 and IL-1β cytokine production [62]. In a recent study, Huang et al. [70] brought attention to the capacity of dietary polyphenols, including RSV, to induce pyroptosis in cancer cells and in those in the tumor microenvironment via different pathways contingent on cancer type. RSV was able to induce pyroptosis by suppressing the activation of the NLRP3 inflammasomes bearing a sensor protein of the NLR family which is one of the key regulators of the inflammatory and innate immune response [71]. Pyroptosis could be enhanced in HT-29 and HCT116 colon cancer cells by certain drugs such as lobaplatin [72].
Cuproptosis is a recently described mechanism that may overcome cancer cells’ drug resistance and leads to cell death by transfer of cupper ionophores into the cells causing cupper accumulation [14] and dysregulation of mitochondrial respiration. Copper overload induces membrane damage, mitochondrial vacuolization, chromatin condensation, and finally cell death [73]. The effect of cuproptosis is closely associated with the activity of the ferrodoxin 1 (FDX1) gene which is downregulated in several types of cancers. Low FDX1 expression correlates with poor prognosis [74]. Copper ions activate the PI3K pathway which in turn suppresses Akt activation [75]. According to Wang et al. [76], cuproptosis dependent on a number of copper-related genes is one of the causes of lung carcinoma cell viability, their detection being of prognostic value. Interestingly, copper-induced cell death caused by curcumin has been reported in HCC cells [77], but elucidating the effect of RSV and other polyphenols on cuproptosis in cancer cells, in general, presents a challenge.
Necroptosis. Galluzi et al. [78] defined necroptosis as a type of programmed cell death dependent on serine-threonine kinase 3 (RIPK3) and mixed lineage kinase domain-like (MLKL). The authors highlighted the molecular mechanisms governing the process in a variety of diseases including malignancies. Because research suggests that necroptosis can function as both a tumor promoter and an inhibitor, its exact role in tumorigenesis is not fully clarified [79]. According to Lee et al. [80] RSV reduced the viability of LNCap prostatic cancer cells by activation of both necroptosis and apoptosis by raising the receptor interacting protein 3 (RIPK3) and mixed-lineage kinase domain like (p-MLKL) levels. This effect was more pronounced when the cells were treated with both RSV and docetaxel.
Future studies must clarify the mechanisms by which RSV causes cancer cell death and the dosage required to accomplish this objective in humans.
Resveratrol and Cancers of the Digestive System
Prior research on 594 patients with different types of esophageal cancer who were given a diet high in polyphenols demonstrated that quercetin and RSV had a dose-dependent protective effect against the growth of the malignancy [81]. RSV, at dosages of 10 mM/L for 24–96 h significantly slowed the development of esophageal cancer EC-9706 cells and caused apoptosis, these effects being dose- and time-dependent. Additionally, in rats receiving intraperitoneal injections of RSV for surgically produced esophagitis, there was a decrease in the progression of Barrett’s metaplasia to esophageal cancer following RSV administration compared to controls [82]. RSV caused apoptosis and enhanced ROS generation in three esophageal cancer cell lines, i.e., OE33, OE19, and FLO-1, whereas the B-cell lymphoma-2 (Bcl-2) apoptosis regulating protein levels were lowered [83]. Further investigation into the potential protective effects of RSV against esophageal cancer appears crucial, given the higher morbidity and death rates associated with the disease and the limited number of therapeutic options now available.
Although primarily in vitro, the RSV immunomodulatory, anti-inflammatory, and anti-cancer characteristics have been explored in gastric carcinoma. Wang et al. [84] have provided a thorough account of the mechanisms by which RSV may limit the genesis, progression, and metastasis of gastric cancer. According to Yang et al. [85], RSV may block IL-6-induced oncogenic uncontrolled cell proliferation and apoptosis by restricting Raf-MAPK pathway activation, which in turn suppresses the growth of the SGC7901 gastric cancer cell line. Suppressing the Wnt signaling pathway had a similar result in MGC-803 gastric cells [86]. When applied to human gastric cancer SGC-7901 cells, RSV elevated the expression of Bax and Bcl-2 apoptosis regulators, downregulated PARP, activated caspase-3, and caused apoptosis. Furthermore, the in vivo proliferation of cancer cells was suppressed in the SGC-7901 xerograph [87]. In the same kind of cells, RSV exerted a dose-dependent reduction in cell viability and an increase in apoptosis because of elevated cleavage of caspases 3 and 8, increased apoptotic Bax, and decreased NF-kB [88]. The ability of RSV to induce apoptosis in gastric cancer cells is explained by down-regulation of the NF-kB signaling pathway, which is known to support cancer progression, suppression of the anti-apoptotic protein, and activation of the apoptotic caspases 3 and 8. Moreover, the polyphenol’s capacity to damage mitochondrial membranes intensifies this action [89]. As a result of decreased NF-kB and heparanase activity by resveratrol, AGS and MKN45 gastric cancer cells expressed lesser invasive function [90]. RSV inhibits gastric cancer cells’ proliferation, invasion and metastasis by targeting miR-155-5p that acts as a pro-inflammatory oncogene [91] and by inhibition of their TGF-β1 induced epithelial-mesenchymal transition potential [92]. When coupled with cisplatin RSV had a synergistic effect in inducing gastric cancer cells’ apoptosis and inhibition of cells metastatic capacity through elevated ROS levels and inhibited mitotic cycle at GO/G1 phase [93].
Colorectal cancer (CRC) is well-known for its high prevalence and mortality rate and its resistance to chemotherapy [88] Research has shown that adding polyphenols, such as RSV, to traditional therapeutic medications may be rather beneficial [94]. The effect of the conventional drugs on tumor size, cancer cells’ cytotoxicity, and apoptosis was discovered to be larger when RSV was admitted concurrently with chemotherapy, according to a recent study based on 21 appropriate publications dealing with animal models with colorectal cancer [95]. Both in CRC human histologic sections and human CRC HCT116 cell line, RSV reduced cell growth by AKT1 and IL-6 downregulation [96]. RSV suppresses the Wnt signaling pathway, as well as cyclins D1 and D2 which are closely related to the development of CRC cells [97], and activates the metastasis-suppressor Raf-1 (RKP) protein [98]. Similarly, repressing and Wnt/β-catenin activation RSV inhibits CRC cells’ proliferation and differentiation while inducing apoptosis [99]. By increasing ROS and Bax expression levels, and inducing Bcl-2 downregulation, RSV promotes the apoptotic pathway and reduces CRC cell viability [100]. Activation of AMPK by RSV reduces the epithelial sodium channels in HCT116 and HT29 human colon cancer cells with a subsequent decrease in their proliferation, migration, and invasion [101]. Additional molecular ways by which RSV may attenuate CRC development include SIRT1, P53, P21, BMP7, COX-2, NF-kB, epithelial-mesenchymal transition (EMT), and certain caspases [102]. Moreover, treatment of CRC cells with RSV suppressed their cross-talk with tumor microenvironment (TME) cells through activation of the Sirt1 pathway, thus abating cell survival and migration [103].
Pancreatic cancer is a fatal illness due to challenges with diagnosis and drug resistance [104]. Efforts to prevent its development and migration by conventional chemotherapy are rather disappointing. Consequently, the chemopreventive properties of RSV in this instance would be advantageous. RSV seems to have the potential to slow the spread of pancreatic cancer in a number of ways. In vitro and animal studies showed that blocking NF-kB activation therapy with RSV decreased the development of pancreatic intraepithelial metaplasia and acinar-to-ductal metaplasia [105]. Research has shown that RSV may affect the cell division and metastatic ability of pancreatic stem cells which are extremely resistant to the drugs now in use. Inhibition of the hypoxia-inducible factor-1α (HIF-1α) is another mechanism by which RSV slows the development and progression of pancreatic cancer cells [106]. Notably, the anti-proliferative effect of RSV depends on the cancer cell type. While all three pancreatic cancer cell lines, i.e., EPP85-181P, EPP85-181RNOV, and AsPC-1 exhibited inhibited proliferation after treatment with RSV, in the mitoxantrone-resistant EPP85-181RNOV cells this effect was observed to a much greater extent. However, the inhibitory effect of RSV on cell proliferation in all three lines proceeded through altered levels of the Bcl-2 pro- and anti-apoptotic proteins [107]. Qin et al. [108] reported a close relationship between autophagy and oxidative stress in relation to the nutrient-deprivation autophagy factor-1 (NAF-1) found in pancreatic cancer tissue and cancer stem cells. The authors revealed that inhibition of NAF-1 activity by RSV leads to inhibited cell migration and invasion. RSV applied concurrently with other phytochemicals to human pancreatic cancer MA-Pa-Ca-2 cells enhanced the activation of the cells’ resistance to oxidants regulator Nrf2 at a higher level than acting alone [109]. Remarkably, it was recently demonstrated that artificially produced RSV derivatives could inhibit the proliferation of pancreatic cells by causing DNA damage, apoptosis, and cell cycle arrest while not affecting normal pancreatic cells [110].
RSV is a useful adjuvant to the currently available medications for the treatment of hepatocellular carcinoma (HCC) because of its anti-inflammatory and anti-oxidative health qualities [111,112]. Considering the connection between inflammation and HCC development, one method of preventing the development of cancer is through RSV’s capacity to inhibit NF-kB activity, which is the catalyst for the start of the inflammatory cascade and the production of pro-inflammatory cytokines TNFα, IL-1α, IL-1β, and IL-6. Moreover, RSV increases the apoptosis of HCC cells by inhibiting the PI3/Akt/mTOR pathway [113]. In a mouse model with HCC induced with Hepa1-6 cells, RSV inhibited tumor growth by enhancing the production of anti-inflammatory cytokines and reducing the number of the immunosuppressive CD8+CD122+ Treg cells. Additionally, there was a decrease in the M2 macrophages [114]. HCC cells are resistant to the apoptotic and growth inhibition capacity of IFN-α. Yang et al. [115] have found that RSV increases the SMMC7721 liver cancer cells’ sensitivity to IFN-α through the SIRT/STAT1 pathway, which in turn promotes apoptosis and inhibits cells’ proliferation. Administration of RSV to hepatic cancer cells inhibited their growth and migration by promoting the activity of the autophagosomal proteins LC3II/I ratio, beclin 1, and the tumor suppressor protein P53 [116]. Tong et al. [117] discovered that RSV may impede Huh7 HCC cells’ development and migration by preventing the Rab27a protein-exosome creation, which plays a significant role in tumor development. A recent research showed that by activating the SIRT1/Nrf2 pathway in a HCC cell line, RSV may upregulate the activity of human telomerase inhibitor-telomerase reverse transcriptase (HepG2), thus reducing cancer cell survival [118]. Concurrent treatment of the HePG2 hepatocellular carcinoma cell line with RSV and berberine had a stronger inhibitory effect on cell viability, development, and apoptosis [119]. The fact that Hep-3B-p53 mutants were more susceptible to this combination suggests that p53 plays a crucial role in the growth of HCC [120]. HCC is resistant to most of the anticancer drugs and actually, the one that cancer cells may respond to is sorafenib. Research conducted in vitro using HepG2 and Hu7 hepatic cancer cell lines, as well as animals with HepG2-induced tumors showed that the combined treatment of sorafenib and RSV had a stronger anticancer effect than that of each component alone. A greater number of cells in the S phase mitotic arrest and apoptosis were indicative of this effect. The AMPK-activated protein kinase (PKA/AMPK/eEF2K) pathway was proposed as the underlying mechanism of this combination [121]. Table 1 illustrates the vast range of RSV dosages used in vitro and animal models and their primary impact on cells derived from digestive tract cancers. Further research presents a significant scientific challenge, as does figuring out an effective, non-toxic dose for humans.
Inhibition of cancer cells’ proliferation and migration and achieving their death should be essential goals in cancer treatment strategy. Unfortunately, cancer remains a challenging disease to treat even with major advancements in immune- and chemotherapy. The review presented herein provides significant insights into the mechanisms by which RSV demonstrates its anti-tumor properties. Notably, elucidating certain constraints of RSV as an anticancer agent, like inadequate pharmacokinetics, and lack of appropriate dose in humans, ought to showcase upcoming viewpoints [122]. Clarifying RSV’s benefits for cancer treatment will require addressing the low bioavailability issue and finding new methods to deliver RSV to the cells. A combination of anticancer drugs with plant polyphenols such as RSV, expressing both immune and anti-proliferative activities could be a feasible adjuvant in the anti-cancer pharmacopeia. It is necessary to move from in vitro experiments to further animal and clinical studies to achieve this goal.
Acknowledgement: None.
Funding Statement: None.
Author Contributions: The author collected and analyzed the data.
Availability of Data and Materials: Not applicable.
Ethics Approval: Not applicable.
Conflicts of Interest: The author declares that they have no conflicts of interest to report regarding the present study.
References
1. Grassi, F., Arroyo-Garcia, R. (2020). Editorial: Origins and domestication of the grape. Frontiers in Plant Science, 11, 1176. [Google Scholar] [PubMed]
2. Pezzuto, J. M. (2008). Grapes and human health: A perspective. Journal of Agriculture and Food Chemistry, 56, 6777–6784. [Google Scholar]
3. Singh, C. K., Liu, X., Ahmad, N. (2015). Resveratrol, in its natural combination in whole grape, for health promotion and disease management. Annals of New York Academy of Science, 1348, 150–160. [Google Scholar]
4. Molani-Gol, R., Rafraf, M. (2023). The anti-obesity effects of resveratrol on the 3T3-L1 adipocytes. International Journal for Vitamin and Nutrition Research. https://doi.org/10.1024/0300-9831/a000784. [Google Scholar] [PubMed] [CrossRef]
5. Venkat, R., Verma, E., Daimary, U. D., Kumar, A., Girisa, S. et al. (2023). The journey of resveratrol from vineyards to clinics. Cancer Investigation, 41, 183–220. [Google Scholar] [PubMed]
6. Shahcheraghi, S. H., Salemi, F., Small, S., Syed, S., Salari, F. et al. (2023). Resveratrol regulates inflammation and improves oxidative stress via Nrf2 signaling pathway: Therapeutic and biotechnological prospects. Phytotherapy Research, 37, 1590–1605. [Google Scholar] [PubMed]
7. Santos, M. A., Franco, F. N., Caldeira, C. A., de Araújo, G. R., Vieira, A. et al. (2023). Resveratrol has its antioxidant and anti-inflammatory protective mechanisms decreased in aging. Archives of Gerontology and Geriatrics, 107, 104895. [Google Scholar] [PubMed]
8. Coutinho-Wolino, K. S., Almeida, P. P., Mafra, D., Stockler-Pinto, M. B. (2022). Bioactive compounds modulating Toll-like 4 receptor (TLR4)-mediated inflammation: Pathways involved and future perspectives. Nutrition Research, 107, 96–116. [Google Scholar] [PubMed]
9. Nigam, M., Mishra, A. P., Deb, V. K., Dimri, D. B., Tiwari, V. et al. (2023). Evaluation of the association of chronic inflammation and cancer: Insights and implications. Biomedicine and Pharmacotherapy, 164, 115015. [Google Scholar] [PubMed]
10. Waldum, H., Fossmark, R. (2023). Inflammation and digestive cancer. International Journal of Molecular Sciences, 24, 13503. [Google Scholar] [PubMed]
11. Maiorino, L., Daßler-Plenker, J., Sun, L., Egeblad, M. (2022). Innate immunity and cancer pathophysiology. Annals Review of Pathology, 17, 425–427. [Google Scholar]
12. Neves, A. R., Lucio, M., Lima, J. L., Reis, S. (2012). Resveratrol in medicinal chemistry: A critical review of its pharmacokinetics, drug-delivery, and membrane interactions. Current Medical Chemistry, 19(11), 1663–1681. [Google Scholar] [PubMed]
13. Sun, X., Li, F., Yuan, L., Bing, Z., Li, X. et al. (2024). pH-responsive resveratrol-loaded ZIF-8 nanoparticles modified with tannic acid for promoting colon cancer cell apoptosis. Journal of Biomedical Materials Research Part B: Applied Biomaterials, 112(1), e35320. [Google Scholar] [PubMed]
14. Tong, X., Tang, R., Xiao, M., Xu, J., Wang, W. et al. (2022). Targeting cell death pathways for cancer therapy: Recent developments in necroptosis, pyroptosis, ferroptosis, and cuproptosis research. Journal of Hematology and Oncology, 15(18), 174. [Google Scholar] [PubMed]
15. Malaguarmera, L. (2019). Influence of resveratrol on the immune response. Nutrition, 11(5), 946. [Google Scholar]
16. Delmas, D., Limagne, E., Ghiringhelli, F., Aires, V. (2020). Immune Th17 lymphocytes play a critical role in the multiple beneficial properties of resveratrol. Food and Chemical Toxicology, 137, 111091. [Google Scholar] [PubMed]
17. Chen, L., Musa, A. E. (2021). Boosting immune system against cancer by resveratrol. Phytotherapy Research, 35(10), 5514–5526. https://doi.org/10.1002/ptr.7189. [Google Scholar] [PubMed] [CrossRef]
18. Shan, G., Minchao, K., Jizhao, W., Rui, Z., Guangjian, Z. et al. (2023). Resveratrol improves the cytotoxic effect of CD8+T cells in the tumor microenvironment by regulating HMMR/Ferroptosis in lung squamous cell carcinoma. Journal of Pharmaceutics and Biomedical Analysis, 229(9), 115346. https://doi.org/10.1016/j.jpba.2023.115346. [Google Scholar] [PubMed] [CrossRef]
19. Lafta, H. A., AbdulHussein, A. H., Al-Shalah, S. A. J., Alnassar, Y. S., Mohammed, N. M. et al. (2023). Tumor-associated macrophages (TAMs) in cancer resistance; modulation by natural products. Current Topics in Medical Chemistry, 23(12), 1104–1122. https://doi.org/10.2174/1568026623666230201145909. [Google Scholar] [PubMed] [CrossRef]
20. Lalani, A. R., Fakhari, F., Radgoudarzi, S., Rastegar-Pouyani, N., Moloudi, K. et al. (2023). Immunoregulation by resveratrol; implications for normal tissue protection and tumour suppression. Clinical and Experimental Pharmacology and Physiology, 50(5), 353–368. https://doi.org/10.1111/1440-1681.13760. [Google Scholar] [PubMed] [CrossRef]
21. Lee, Y. J., Kim, J. (2020). Resveratrol activates natural killer cells through Akt- and mTORC2-mediated c-Myb upregulation. International Journal of Molecular Sciences, 21(24), 9575. https://doi.org/10.3390/ijms21249575. [Google Scholar] [PubMed] [CrossRef]
22. Nosáľ, R., Drábiková, K., Jančinová, V., Perečko, T., Ambrožová, G. et al. (2014). On the molecular pharmacology of resveratrol on oxidative burst inhibition in professional phagocytes. Oxidative Medicine and Cellular Longevity, 2014(1), 706269. https://doi.org/10.1155/2014/706269. [Google Scholar] [PubMed] [CrossRef]
23. Bergman, M., Levin, G. S., Bessler, H., Djaldetti, M., Salman, H. (2013). Resveratrol affects the cross talk between immune and colon cancer cells. Biomedicine and Pharmacotherapy, 67(1), 43–47. https://doi.org/10.1016/j.biopha.2012.10.008. [Google Scholar] [PubMed] [CrossRef]
24. Palacz-Wrobel, M., Borkowska, P., Paul-Samojedny, M., Kowalczyk, M., Fila-Danilow, A. et al. (2017). Effect of apigenin, kaempferol and resveratrol on the gene expression and protein secretion of tumor necrosis factor alpha (TNF-α) and interleukin-10 (IL-10) in RAW-264.7 macrophages. Biomedicine and Pharmacotherapy, 93, 1205–1212. https://doi.org/10.1016/j.biopha.2017.07.054. [Google Scholar] [PubMed] [CrossRef]
25. Feng, L., Yasmeen, R., Schoene, N. W., Lei, K. Y., Wang, T. T. Y. (2019). Resveratrol differentially modulates immune responses in human THP-1 monocytes and macrophages. Nutrition Research, 72(8), 57–69. https://doi.org/10.1016/j.nutres.2019.10.003. [Google Scholar] [PubMed] [CrossRef]
26. Walker, J., Schuelle, K., Schaefer, L. M., Pignitter, M., Esefelder, L. et al. (2014). Resveratrol and its metabolites inhibit pro-inflammatory effects of lipopolysaccharides in U-937 macrophages in plasma-representative concentrations. Food and Function, 5(1), 74–84. https://doi.org/10.1039/C3FO60236B. [Google Scholar] [PubMed] [CrossRef]
27. Alesci, A., Nicosia, N., Fumi, A., Giorgianni, F., Santini, A. et al. (2022). Resveratrol and immune cells, a link to improve human health. Molecules, 27(2), 424. https://doi.org/10.3390/molecules27020424. [Google Scholar] [PubMed] [CrossRef]
28. Ma, C., Wang, Y., Dong, L., Li, M., Cai, W. (2015). Anti-inflammatory effect of resveratrol through the suppression of NF-κB and JAK/STAT signaling pathways. Acta Biochimica et Biophysica Sinica, 47(3), 207–213. https://doi.org/10.1093/abbs/gmu135. [Google Scholar] [PubMed] [CrossRef]
29. Ma, C., Wang, Y., Shen, A., Cai, W. (2017). Resveratrol upregulates SOCS1 production by lipopolysaccharide-stimulated RAW264.7 macrophages by inhibiting miR-155. International Journal of Molecular Medicine, 39(1), 231–237. https://doi.org/10.3892/ijmm.2016.2802. [Google Scholar] [PubMed] [CrossRef]
30. Pinheiro, D. M. L., de Oliveira, A. H. S., Coutinho, L. G., Fontes, F. L., de Medeiros Oliveira, R. K. et al. (2019). Resveratrol decreases the expression of genes involved in inflammation through transcriptional regulation. Free Radical Biology and Medicine, 130(26), 8–22. https://doi.org/10.1016/j.freeradbiomed.2018.10.432. [Google Scholar] [PubMed] [CrossRef]
31. Bigagli, E., Cinci, L., Paccosi, S., Parenti, A., D'Ambrosio, M. et al. (2017). Nutritionally relevant concentrations of resveratrol and hydroxytyrosol mitigate oxidative burst of human granulocytes and monocytes and the production of pro-inflammatory mediators in LPS-stimulated RAW 264.7 macrophages. International Immunopharmacology, 43(5), 147–155. https://doi.org/10.1016/j.intimp.2016.12.012. [Google Scholar] [PubMed] [CrossRef]
32. Zheng, H., Qiu, C., Jin, H., Sun, Y., Li, Z. (2020). Resveratrol reduces apoptosis of mouse RAW264.7 macrophage via down-regulating HIF-1α. Chinese Journal of Cellular and Molecular Immunology, 36(1), 42–48 (In Chinese). [Google Scholar] [PubMed]
33. Ding, Y., Yang, P., Li, S., Zhang, H., Ding, X. et al. (2022). Resveratrol accelerates wound healing by inducing M2 macrophage polarization in diabetic mice. Pharmaceutical Biology, 60(1), 2328–2337. https://doi.org/10.1080/13880209.2022.2149821. [Google Scholar] [PubMed] [CrossRef]
34. Schwager, J., Bompard, A., Raederstorff, D., Hug, H., Bendik, I. (2022). Resveratrol and ω-3 PUFAs promote human macrophage differentiation and function. Biomedicines, 10(7), 1524. https://doi.org/10.3390/biomedicines10071524. [Google Scholar] [PubMed] [CrossRef]
35. Quan, H., Yin, M., Kim, J., Jang, E. A., Yang, S. H. et al. (2021). Resveratrol suppresses the reprogramming of macrophages into an endotoxin-tolerant state through the activation of AMP-activated protein kinase. European Journal of Pharmacology, 899, 173993. https://doi.org/10.1016/j.ejphar.2021.173993. [Google Scholar] [PubMed] [CrossRef]
36. Yanez, M., Jhanji, M., Murphy, K., Gower, R. M., Sajish, M. et al. (2019). Nicotinamide augments the anti-Inflammatory properties of resveratrol through PARP1 activation. Scientific Reports, 9(1), 10219. https://doi.org/10.1038/s41598-019-46678-8. [Google Scholar] [PubMed] [CrossRef]
37. Wu, S. X., Xiong, R. G., Huang, S. Y., Zhou, D. D., Saimaiti, A. et al. (2023). Effects and mechanisms of resveratrol for prevention and management of cancers: An updated review. Critical Review of Food Science and Nutrition, 63(33), 12422–12440. [Google Scholar]
38. Rauf, A., Imran, M., Butt, M. S., Nadeem, M., Peters, D. G. et al. (2018). Resveratrol as an anti-cancer agent: A review. Critical Review of Food Science and Nutrition, 58(9), 1428–1447. [Google Scholar]
39. Han, Y., Jo, H., Cho, J. H., Dhanasekaran, D. N., Song, Y. S. (2019). Resveratrol as a tumor-suppressive nutraceutical modulating tumor microenvironment and malignant behaviors of cancer. International Journal of Molecular Sciences, 20(4), 925. [Google Scholar] [PubMed]
40. Deng, L. J., Qi, M., Li, N., Lei, Y. H., Zhang, D. M. et al. (2020). Natural products and their derivatives: Promising modulators of tumor immunotherapy. Journal of Leukocyte Biology, 108(2), 493–508. [Google Scholar] [PubMed]
41. Talib, W. H., Alsayed, A. R., Farhan, F., Al Kury, L. T. (2020). Resveratrol and tumor microenvironment: Mechanistic basis and therapeutic targets. Molecules, 25(18), 4282. [Google Scholar] [PubMed]
42. Kursvietiene, L., Kopustinskiene, D. M., Staneviciene, I., Mongirdiene, A., Kubová, K. et al. (2023). Anti-cancer properties of resveratrol: A focus on its impact on mitochondrial functions. Antioxidants, 12(12), 2056–2080. [Google Scholar] [PubMed]
43. Caserta, S., Genovese, C., Cicero, N., Toscano, V., Gangemi, S. et al. (2023). The interplay between medical plants and gut microbiota in cancer. Nutrients, 15(15), 3327. [Google Scholar] [PubMed]
44. Sun, L., Chen, B., Jiang, R., Li, J., Wang, B. (2017). Resveratrol inhibits lung cancer growth by suppressing M2-like polarization of tumor associated macrophages. Cell Immunology, 311, 86–93. [Google Scholar]
45. Cheuk, I. W., Chen, J., Siu, M., Ho, J. C., Lam, S. S. et al. (2022). Resveratrol enhanced chemosensitivity by reversing macrophage polarization in breast cancer. Clinical and Translation Oncology, 24(5), 854–863. [Google Scholar] [PubMed]
46. Kimura, Y., Sumiyoshi, M. (2016). Resveratrol prevents tumor growth and metastasis by inhibiting lymphangiogenesis and M2 macrophage activation and differentiation in tumor-associated macrophages. Nutrition and Cancer, 68(4), 667–678. [Google Scholar] [PubMed]
47. Song, B., Wang, W., Tang, X., Goh, R. M. W., Thuya, W. L. et al. (2023). Inhibitory potential of resveratrol in cancer metastasis: From biology to therapy. Cancers, 15(10), 2758. [Google Scholar] [PubMed]
48. Komorowska, D., Radzik, T., Kalenik, S., Rodacka, A. (2022). Natural radiosensitizers in radiotherapy: Cancer treatment by combining ionizing radiation with resveratrol. International Journal of Molecular Sciences, 23(18), 10627. [Google Scholar] [PubMed]
49. Pai Bellare, G., Sankar Patro, B. (2022). Resveratrol sensitizes breast cancer to PARP inhibitor, talazoparib through dual inhibition of AKT and autophagy flux. Biochemistry and Pharmacology, 199, 115024. [Google Scholar]
50. Galluzzi, L., Bravo-San Pedro, J. M., Vitale, I., Aaronson, S. A., Abrams, J. M. et al. (2015). Essential versus accessory aspects of cell death: Recommendations of the NCCD 2015. Cell Death Differentiation, 22, 58–73. [Google Scholar] [PubMed]
51. Jiang, H., Wang, G., Gu, J., Xiao, Y., Wang, P. et al. (2022). Resveratrol inhibits the expression of RYR2 and is a potential treatment for pancreatic cancer. Naunyn Schmiedebergs Archives of Pharmacology, 395, 315–324. [Google Scholar] [PubMed]
52. Musial, C., Siedlecka-Kroplewska, K., Kmiec, Z., Gorska-Ponikowska, M. (2021). Modulation of autophagy in cancer cells by dietary polyphenols. Antioxidants, 10(1), 123. [Google Scholar] [PubMed]
53. Xie, J., Yang, Y., Gao, Y., He, J. (2023). Cuproptosis: Mechanisms and links with cancers. Molecular Cancer, 22(1), 46. [Google Scholar] [PubMed]
54. Wang, J., Huang, P., Pan, X., Xia, C., Zhang, H. et al. (2023). Resveratrol reverses TGF-β1-mediated invasion and metastasis of breast cancer cells via the SIRT3/AMPK/autophagy signal axis. Phytotherapy Research, 37(1), 211–230. [Google Scholar] [PubMed]
55. Torrens-Mas, M., Oliver, J., Roca, P., Sastre-Serra, J. (2017). SIRT3: Oncogene and tumor suppressor in cancer. Cancers, 9(7), 90. [Google Scholar] [PubMed]
56. Li, J., Fan, Y., Zhang, Y., Liu, Y., Yu, Y. et al. (2022). Resveratrol induces autophagy and apoptosis in non-small-cell lung cancer cells by activating the NGFR-AMPK-mTOR pathway. Nutrients, 14(12), 2413. [Google Scholar] [PubMed]
57. Tian, Y., Song, W., Li, D., Cai, L., Zhao, Y. (2019). Resveratrol as a natural regulator of autophagy for prevention and treatment of cancer. OncoTargets and Therapy, 12, 8601–8609. [Google Scholar] [PubMed]
58. Park, D., Jeong, H., Lee, M. N., Koh, A., Kwon, O. et al. (2016). Resveratrol induces autophagy by directly inhibiting mTOR through ATP competition. Scientific Reports, 6, 21772. [Google Scholar] [PubMed]
59. Fan, Y., Chiu, J. F., Liu, J., Deng, Y., Xu, C. et al. (2018). Resveratrol induces autophagy-dependent apoptosis in HL-60 cells. BMC Cancer, 18(1), 581. [Google Scholar] [PubMed]
60. Ashrafizadeh, M., Taeb, S., Haghi-Aminjan, H., Afrashi, S., Moloudi, K. et al. (2021). Resveratrol as an enhancer of apoptosis in cancer: A mechanistic review. Anticancer Agents in Medical Chemistry, 21(17), 2327–2336. [Google Scholar]
61. Brockmueller, A., Buhrmann, C., Shayan, P., Shakibaei, M. (2023). Resveratrol induces apoptosis by modulating the reciprocal crosstalk between p53 and Sirt-1 in the CRC tumor microenvironment. Frontiers in Immunology, 14, 1225530. [Google Scholar] [PubMed]
62. Fang, Y., Tian, S., Pan, Y., Li, W., Wang, Q. et al. (2020). Pyroptosis: A new frontier in cancer. Biomedicine and Pharmacotherapy, 121, 109595. [Google Scholar] [PubMed]
63. Asemi, R., Nikoo, N. R., Asemi, Z., Shafabakhsh, R., Hajijafari, M. et al. (2023). Modulation of long non-coding RNAs by resveratrol as a potential therapeutic approach in cancer: A comprehensive review. Pathology Research and Practice, 246, 154507. [Google Scholar] [PubMed]
64. Cal, C., Garban, H., Jazirehi, A., Yeh, C., Mizutani, Y. et al. (2003). Resveratrol and cancer: Chemoprevention, apoptosis, and chemo-immunosensitizing activities. Current Medicinal Chemistry Anticancer Agents, 3(2), 77–93. [Google Scholar] [PubMed]
65. Stepanić, V., Kučerová-Chlupáčová, M. (2023). Review and chemoinformatic analysis of ferroptosis modulators with a focus on natural plant products. Molecules, 28(2), 475. [Google Scholar] [PubMed]
66. Shi, Z. Z., Fan, Z. W., Chen, Y. X., Xie, X. F., Jiang, W. et al. (2019). Ferroptosis in carcinoma: Regulatory mechanisms and new method for cancer therapy. OncoTargets and Therapy, 12, 11291–11304. [Google Scholar] [PubMed]
67. Kang, R., Tang, D. (2017). Autophagy and ferroptosis—What’s the connection? Current Pathobiology Reports, 5(2), 153–159. https://doi.org/10.1007/s40139-017-0139-5. [Google Scholar] [PubMed] [CrossRef]
68. Zhang, Z., Ji, Y., Hu, N., Yu, Q., Zhang, X. et al. (2022). Ferroptosis-induced anticancer effect of resveratrol with a biomimetic nano-delivery system in colorectal cancer treatment. Asian Journal of Pharmacological Sciences, 17(5), 751–766. https://doi.org/10.1016/j.ajps.2022.07.006. [Google Scholar] [PubMed] [CrossRef]
69. Lee, J., You, J. H., Kim, M. S., Roh, J. L. (2020). Epigenetic reprogramming of epithelial-mesenchymal transition promotes ferroptosis of head and neck cancer. Redox Biology, 37, 101697. https://doi.org/10.1016/j.redox.2020.101697. [Google Scholar] [PubMed] [CrossRef]
70. Huang, X., Wang, Y., Yang, W., Dong, J., Li, L. (2022). Regulation of dietary polyphenols on cancer cell pyroptosis and the tumor immune microenvironment. Frontiers in Nutrition, 9, 974896. https://doi.org/10.3389/fnut.2022.974896. [Google Scholar] [PubMed] [CrossRef]
71. Chang, Y. P., Ka, S. M., Hsu, W. H., Chen, A., Chao, L. K. et al. (2015). Resveratrol inhibits NLRP3 inflammasome activation by preserving mitochondrial integrity and augmenting autophagy. Journal of Cell Physiology, 230(7), 1567–1579. https://doi.org/10.1002/jcp.24903. [Google Scholar] [PubMed] [CrossRef]
72. Yu, J., Li, S., Qi, J., Chen, Z., Wu, Y. et al. (2019). Cleavage of GSDME by caspase-3 determines lobaplatin-induced pyroptosis in colon cancer cells. Cell Death and Disease, 10(3), 193. https://doi.org/10.1038/s41419-019-1441-4. [Google Scholar] [PubMed] [CrossRef]
73. Wang, D., Tian, Z., Zhang, P., Zhen, L., Meng, Q. et al. (2023). The molecular mechanisms of cuproptosis and its relevance to cardiovascular disease. Biomedicine and Pharmacotherapy, 163(1), 114830. https://doi.org/10.1016/j.biopha.2023.114830. [Google Scholar] [PubMed] [CrossRef]
74. Cao, S., Wang, Q., Sun, Z., Zhang, Y., Liu, Q. et al. (2023). Role of cuproptosis in understanding diseases. Human Cell, 36(4), 1244–1252. https://doi.org/10.1007/s13577-023-00914-6. [Google Scholar] [PubMed] [CrossRef]
75. Xie, Q., Chen, Y., Tan, H., Liu, B., Zheng, L. L. et al. (2021). Targeting autophagy with natural compounds in cancer: A renewed perspective from molecular mechanisms to targeted therapy. Frontiers in Pharmacology, 12, 748149. https://doi.org/10.3389/fphar.2021.748149. [Google Scholar] [PubMed] [CrossRef]
76. Wang, S., Xing, N., Meng, X., Xiang, L., Zhang, Y. (2022). Comprehensive bioinformatics analysis to identify a novel cuproptosis-related prognostic signature and its ceRNA regulatory axis and candidate traditional Chinese medicine active ingredients in lung adenocarcinoma. Frontiers in Pharmacology, 13, 971867. https://doi.org/10.3389/fphar.2022.971867. [Google Scholar] [PubMed] [CrossRef]
77. Liu, Z., Ma, H., Lai, Z. (2023). The role of ferroptosis and cuproptosis in curcumin against hepatocellular carcinoma. Molecules, 28(4), 1623. [Google Scholar] [PubMed]
78. Galluzzi, L., Kepp, O., Chan, F. K., Kroemer, G. (2017). Necroptosis: Mechanisms and relevance to disease. Annual Review of Pathology, 12, 103–130. [Google Scholar] [PubMed]
79. Liu, Z. G., Jiao, D. (2019). Necroptosis, tumor necrosis and tumorigenesis. Cell Stress, 4(1), 1–8. [Google Scholar] [PubMed]
80. Lee, S. H., Lee, Y. J. (2021). Synergistic anticancer activity of resveratrol in combination with docetaxel in prostate carcinoma cells. Nutrition Research and Practice, 15(1), 12–25. [Google Scholar] [PubMed]
81. Lin, Y., Yngve, A., Lagergren, J., Lu, Y. (2014). A dietary pattern rich in lignans, quercetin and resveratrol decreases the risk of oesophageal cancer. British Journal of Nutrition, 112(12), 2002–2009. [Google Scholar] [PubMed]
82. Zhou, H. B., Yan, Y., Sun, Y. N., Zhu, J. R. (2003). Resveratrol induces apoptosis in human esophageal carcinoma cells. World Journal of Gastroenterology, 9(3), 408–411. https://doi.org/10.3748/wjg.v9.i3.408. [Google Scholar] [PubMed] [CrossRef]
83. Dhir, H., Choudhury, M., Patil, K., Cheung, C., Bodlak, A. et al. (2021). Interception of signaling circuits of esophageal adenocarcinoma cells by resveratrol reveals molecular and immunomodulatory signatures. Cancers, 13(2), 5811. https://doi.org/10.3390/cancers13225811. [Google Scholar] [PubMed] [CrossRef]
84. Wang, L. Y., Zhao, S., Lv, G. J., Ma, X. J., Zhang, J. B. (2020). Mechanisms of resveratrol in the prevention and treatment of gastrointestinal cancer. World Journal of Clinical Cases, 8(12), 2425–2437. https://doi.org/10.12998/wjcc.v8.i12.2425. [Google Scholar] [PubMed] [CrossRef]
85. Yang, T., Zhang, J., Zhou, J., Zhu, M., Wang, L. et al. (2018). Resveratrol inhibits interleukin-6 induced invasion of human gastric cancer cells. Biomedicine and Pharmacotherapy, 99, 766–773. https://doi.org/10.1016/j.biopha.2018.01.153. [Google Scholar] [PubMed] [CrossRef]
86. Dai, H., Deng, H. B., Wang, Y. H., Guo, J. J. (2018). Resveratrol inhibits the growth of gastric cancer via the Wnt/β-catenin pathway. Oncology Letters, 16(2), 1579–1583. https://doi.org/10.3892/ol.2018.8772. [Google Scholar] [PubMed] [CrossRef]
87. Yang, Y., Huang, X., Chen, S., Ma, G., Zhu, M. et al. (2018). Resveratrol induced apoptosis in human gastric carcinoma SGC-7901 cells via activation of mitochondrial pathway. Asia-Pacific Journal of Clinical Oncology, 4, e317–e324. [Google Scholar]
88. Wu, X., Xu, Y., Zhu, B., Liu, Q., Yao, Q. et al. (2018). Resveratrol induces apoptosis in SGC-7901 gastric cancer cells. Oncology Letters, 16, 2949–2956. [Google Scholar] [PubMed]
89. Ashrafizadeh, M., Javanmardi, S., Moradi-Ozarlou, M., Mohammadinejad, R., Farkhondeh, T. et al. (2020). Natural products and phytochemical nanoformulations targeting mitochondria in oncotherapy: An updated review on resveratrol. Bioscience Reports, 40(4), BSR20200257. [Google Scholar] [PubMed]
90. Rojo, D., Madrid, A., Martín, S. S., Párraga, M., Silva Pinhal, M. A. et al. (2022). Resveratrol decreases the invasion potential of gastric cancer cells. Molecules, 27(10), 3047. [Google Scholar] [PubMed]
91. Su, N., Li, L., Zhou, E., Li, H., Wu, S. et al. (2022). Resveratrol downregulates miR-155-5p to block the malignant behavior of gastric cancer cells. Biomedical Research International, 2022, 6968641. [Google Scholar]
92. Deng, L., Zou, J., Su, Y., Wang, M., Zhao, L. (2022). Resveratrol inhibits TGF-β1-induced EMT in gastric cancer cells through Hippo-YAP signaling pathway. Clinical and Translation Oncology, 24(11), 2210–2221. https://doi.org/10.1007/s12094-022-02882-z. [Google Scholar] [PubMed] [CrossRef]
93. Rahimifard, M., Baeeri, M., Mousavi, T., Azarnezhad, A., Haghi-Aminjan, H. et al. (2023). Combination therapy of cisplatin and resveratrol to induce cellular aging in gastric cancer cells: Focusing on oxidative stress, and cell cycle arrest. Frontiers in Pharmacology, 13, 1068863. https://doi.org/10.3389/fphar.2022.1068863. [Google Scholar] [PubMed] [CrossRef]
94. Ding, S., Xu, S., Fang, J., Jiang, H. (2020). The protective effect of polyphenols for colorectal cancer. Frontiers in Immunology, 11, 1407. https://doi.org/10.3389/fimmu.2020.01407. [Google Scholar] [PubMed] [CrossRef]
95. Wu, X. Y., Zhai, J., Huan, X. K., Xu, W. W., Tian, J. et al. (2023). A Systematic review of the therapeutic potential of resveratrol during colorectal cancer chemotherapy. Mini Review of Medical Chemistry, 23(10), 1137–1152. https://doi.org/10.2174/1389557522666220907145153. [Google Scholar] [PubMed] [CrossRef]
96. Li, R., Ma, X., Song, Y., Zhang, Y., Xiong, W. et al. (2019). Anti-colorectal cancer targets of resveratrol and biological molecular mechanism: Analyses of network pharmacology, human and experimental data. Journal of Cell Biochemistry, 120(7), 11265–11273. https://doi.org/10.1002/jcb.28404. [Google Scholar] [PubMed] [CrossRef]
97. Honari, M., Shafabakhsh, R., Reiter, R. J., Mirzaei, H., Asemi, Z. (2019). Resveratrol is a promising agent for colorectal cancer prevention and treatment: Focus on molecular mechanisms. Cancer Cell International, 19, 180. [Google Scholar] [PubMed]
98. Dariya, B., Behera, S. K., Srivani, G., Aliya, S., Alam, A. et al. (2020). Resveratrol binds and activates RKIP protein in colorectal cancer. Amino Acids, 52(9), 1299–1306. [Google Scholar] [PubMed]
99. Pashirzad, M., Johnston, T. P., Sahebkar, A. (2021). Therapeutic effects of polyphenols on the treatment of colorectal cancer by regulating Wnt β-Catenin signaling pathway. Journal of Oncology, 2021, 3619510. [Google Scholar] [PubMed]
100. Fu, X., Li, M., Tang, C., Huang, Z., Najafi, M. (2021). Targeting of cancer cell death mechanisms by resveratrol: A review. Apoptosis, 26(11–12), 561–573. [Google Scholar] [PubMed]
101. Gündoğdu, A.Ç., Özyurt, R. (2023). Resveratrol downregulates ENaCs through the activation of AMPK in human colon cancer cells. Tissue and Cell, 82, 102071. [Google Scholar] [PubMed]
102. Vernousfaderani, E. K., Akhtari, N., Rezaei, S., Rezaee, Y., Shiranirad, S. et al. (2021). Resveratrol and colorectal cancer: A molecular approach to clinical researches. Current Topics in Medical Chemistry, 21(29), 2634–2646. [Google Scholar]
103. Buhrmann, C., Shayan, P., Brockmueller, A., Shakibaei, M. (2020). Resveratrol suppresses cross-talk between colorectal cancer cells and stromal cells in multicellular tumor microenvironment: A bridge between in vitro and in vivo tumor microenvironment study. Molecules, 25(18), 4292. [Google Scholar]
104. Subramaniam, D., Kaushik, G., Dandawate, P., Anant, S. (2018). Targeting cancer stem cells for chemoprevention of pancreatic cancer. Current Medicinal Chemistry, 25(22), 2585–2594. [Google Scholar] [PubMed]
105. Qian, W., Xiao, Q., Wang, L., Qin, T., Xiao, Y. et al. (2020). Resveratrol slows the tumourigenesis of pancreatic cancer by inhibiting NFκB activation. Biomedicine and Pharmacotherapy, 127, 110116. [Google Scholar] [PubMed]
106. Srivani, G., Behera, S. K., Dariya, B., Aliya, S., Alam, A. et al. (2020). Resveratrol binds and inhibits transcription factor HIF-1α in pancreatic cancer. Experimental Cell Research, 394(1), 112126. [Google Scholar] [PubMed]
107. Ratajczak, K., Glatzel-Plucińska, N., Ratajczak-Wielgomas, K., Nowińska, K., Borska, S. (2021). Effect of resveratrol treatment on human pancreatic cancer cells through alterations of Bcl-2 family members. Molecules, 26(21), 6560. https://doi.org/10.3390/molecules26216560. [Google Scholar] [PubMed] [CrossRef]
108. Qin, T., Cheng, L., Xiao, Y., Qian, W., Li, J. et al. (2020). NAF-1 inhibition by resveratrol suppresses cancer stem cell-like properties and the invasion of pancreatic cancer. Frontiers in Oncology, 10, 1038. https://doi.org/10.3389/fonc.2020.01038. [Google Scholar] [PubMed] [CrossRef]
109. Cykowiak, M., Krajka-Kuźniak, V., Baer-Dubowska, W. (2022). Combinations of phytochemicals more efficiently than single components activate Nrf2 and induce the expression of antioxidant enzymes in pancreatic cancer cells. Nutrition Cancer, 74(3), 996–1011. https://doi.org/10.1080/01635581.2021.1933097. [Google Scholar] [PubMed] [CrossRef]
110. Florio, R., De Filippis, B., Veschi, S., di Giacomo, V., Lanuti, P. et al. (2023). Resveratrol derivative exhibits marked antiproliferative actions, affecting stemness in pancreatic cancer cells. International Journal of Molecular Sciences, 24(3), 1977. https://doi.org/10.3390/ijms24031977. [Google Scholar] [PubMed] [CrossRef]
111. Bishayee, A., Politis, T., Darvesh, A. S. (2010). Resveratrol in the chemoprevention and treatment of hepatocellular carcinoma. Cancer Treatment Reviews, 36(1), 43–53. https://doi.org/10.1016/j.ctrv.2009.10.002. [Google Scholar] [PubMed] [CrossRef]
112. Singh, A. K., Singh, S. V., Kumar, R., Kumar, S., Senapati, S. et al. (2023). Current therapeutic modalities and chemopreventive role of natural products in liver cancer: Progress and promise. World Journal of Hepatology, 15(1), 1–18. [Google Scholar] [PubMed]
113. Chupradit, S., Bokov, D., Zamanian, M. Y., Heidari, M., Hakimizadeh, E. (2022). Hepatoprotective and therapeutic effects of resveratrol: A focus on anti-inflammatory and antioxidative activities. Fundam Clinical Pharmacology, 36(8), 468–485. [Google Scholar]
114. Zhang, Q., Huang, H., Zheng, F., Liu, H., Qiu, F. et al. (2020). Resveratrol exerts antitumor effects by downregulating CD8+CD122+ Tregs in murine hepatocellular carcinoma. Oncoimmunology, 9(1), 1829346. [Google Scholar] [PubMed]
115. Yang, Z., Meng, Q., Zhao, Y., Han, R., Huang, S. et al. (2018). Resveratrol promoted interferon-α-Induced growth inhibition and apoptosis of SMMC7721 cells by activating the SIRT/STAT1. Journal of Interferon and Cytokine Research, 38(6), 261–271. [Google Scholar] [PubMed]
116. Zhang, B., Yin, X., Sui, S. (2018). Resveratrol inhibited the progression of human hepatocellular carcinoma by inducing autophagy via regulating p53 and the phosphoinositide 3-kinase/protein kinase B pathway. Oncology Reports, 40(5), 2758–2765. [Google Scholar] [PubMed]
117. Tong, K., Wang, P., Li, Y., Tong, Y., Li, X. et al. (2024). Resveratrol inhibits hepatocellular carcinoma progression through regulating exosome secretion. Current Medicinal Chemistry, 31(15), 2107–2118. [Google Scholar] [PubMed]
118. Moghadam, D., Zarei, R., Vakili, S., Ghojoghi, R., Zarezade, V. et al. (2023). The effect of natural polyphenols resveratrol, gallic acid, and kuromanin chloride on human telomerase reverse transcriptase (hTERT) expression in HepG2 hepatocellular carcinoma: Role of SIRT1/Nrf2 signaling pathway and oxidative stress. Molecular Biology Reports, 50(1), 77–84. [Google Scholar] [PubMed]
119. D’Arcy, M. S., Pike, C. V. S., Coussons, P. J. (2021). A novel combined resveratrol/berberine phytochemotheraputic using the HePG2 cell line as a model for the treatment of hepatocarcinoma. Cell Biology International, 45(12), 2499–2509. [Google Scholar] [PubMed]
120. Skonieczna, M., Adamiec-Organisciok, M., Hudy, D., Dziedzic, A., Los, L. et al. (2022). Hepatocellular cancer cell lines, Hep-3B and Hep-G2 display the pleiotropic response to resveratrol and berberine. Advances in Medical Sciences, 67(2), 379–385. [Google Scholar] [PubMed]
121. Gao, M., Deng, C., Dang, F. (2021). Synergistic antitumor effect of resveratrol and sorafenib on hepatocellular carcinoma through PKA/AMPK/eEF2K pathway. Food and Nutrition Research, 13, 65. [Google Scholar]
122. Ren, B., Kwah, M. X., Liu, C., Ma, Z., Shanmugam, M. K. et al. (2021). Resveratrol for cancer therapy: Challenges and future perspectives. Cancer Letters, 515, 63–72. [Google Scholar] [PubMed]
Cite This Article
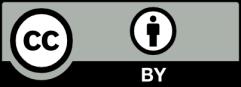
This work is licensed under a Creative Commons Attribution 4.0 International License , which permits unrestricted use, distribution, and reproduction in any medium, provided the original work is properly cited.