Open Access
REVIEW
Ferroptosis’s Role in Genitourinary System Cancer
1 Zhumadian Academy of Industry Innovation and Development, HuangHuai University, Zhumadian, 463000, China
2 School of Biological and Food Processing Engineering, HuangHuai University, Zhumadian, 463000, China
* Corresponding Author: Enzhong Li. Email:
# These authors contributed equally to this work and share first authorship
(This article belongs to the Special Issue: Ferroptosis in the Occurrence and Treatment of Cancer)
Oncologie 2022, 24(4), 679-691. https://doi.org/10.32604/oncologie.2022.025705
Received 26 July 2022; Accepted 17 October 2022; Issue published 31 December 2022
Abstract
A cell is the basic unit of life, and death is inevitable for any cell. However, cancer cells that deviate from the normal track can resist death and survive. Ferroptosis is recently discovered as a modulated cell death different from other known forms of cell death in morphology, biochemistry, and genetics. It is characterized by iron-dependent lipid peroxidation regulated by various metabolic pathways. The incidence and mortality of genitourinary system cancer have been increasing recently. Although clinical practice therapy techniques have improved, no plan with a positive prognosis has been identified. For the therapy of cancer, ferroptosis opens up new avenues. Many studies have shown a complex link between ferroptosis and cancer, while some studies have also found the role of ferroptosis in genitourinary system-related cancers and therapeutic prospects. This article reviews the ferroptosis research progress in genitourinary system cancers, including bladder cancer, prostate cancer, ovarian cancer, and cervical cancer. It will also provide new ideas for the treatment of these cancers.Keywords
Nomenclature
GPX4 | Glutathione Peroxidase 4 |
ROS | Reactive Oxygen Species |
GSH | Glutathione |
FINs | Ferroptosis-Inducing Agents |
HSPB1 | Heat Shock Protein Beta-1 |
LIP | Labile Iron Pool |
TXNRD1 | Thioredoxin Reductase 1 |
ACSL4 | Acyl-CoA Synthetase Long-Chain Family Member 4 |
PUFAs | Polyunsaturated Fatty Acids |
TFR/TFRC | Transferrin Receptor |
FPN | Ferroportin |
PCa | Prostate Cancer |
AR | Androgen Receptor |
CRPC | Castration-Resistant Prostate Cancer |
MDA | Malonaldehyde |
Nrf2 | NF-E2-Related Factor 2 |
ITC | Isothiocyanate |
BSO | Buthionine Sulfoximine |
ATF6 | Activating Transcription Factor 6 |
Ceapin-A7 | The ATF6α Inhibitor |
DATS | Diallyl Trisulfide |
DFS | Disease-Free Survival |
BCa | Bladder Cancer |
MIBC | Muscle-Invasive Bladder Cancer |
NMIBC | Non-Muscle-Invasive Bladder Cancer |
RC | Radical Cystectomy |
BCG | Bacillus Calmette-Guérin |
YAP | Yes-Associated Protein |
TNM | Tumor Node Metastasis |
MAP30 | A Bioactive Protein Isolated From Bitter Melon Seeds |
COX-2 | Cyclooxygenase-2 |
SCD1 | Stearoyl-CoA-Desaturase-1 |
PARP | Poly ADP-Ribose Polymerases |
SNAI2 | A Zinc Finger Protein |
DMOG | Dimethyloxallyl Glycine |
OA | Oleanolic Acid |
SNG | Sanguinarine |
SCC | Squamous Cell Carcinoma |
Ferroptosis was originally discovered by Dixon et al. [1] in 2012 as a unique iron-dependent death, which is different from apoptosis, necrosis, and autophagy on a morphological, genetic and biochemical basis. It is due to lipid peroxide accumulation because of intracellular redox homeostasis imbalance caused by excessive iron. Iron chelating agents can reduce the iron level in cells and prevent the formation of active free radicals, preventing cell death [1]. Iron is a redox-active metal involved in the free radical formation and lipid peroxidation that plays an important role in controlling cell death. The sensitivity of cells to ferroptosis is regulated by various genes or proteins involved in iron homeostasis [2]. Cancer cells usually rely on more iron to fuel their growth than normal non-cancer cells, making them more susceptible to ferroptosis. Glutathione peroxidase4 (GPX4) is a selenoprotein expressed in most tissues and organs. It can detoxicate complex phospholipid hydroperoxide and inhibit the production of reactive oxygen species (ROS). GPX4 has been identified as a central regulator of ferroptosis, and inhibition of GPX4 activity or knockdown of the GPX4 gene can induce ferroptosis [3]. GPX4 typically reduces toxic phospholipid hydroperoxides into lipid alcohols using two electrons provided by glutathione (GSH). GSH is a tripeptide antioxidant synthesized from cysteine and is also the cofactor of many enzymes. Almost all cells can synthesize this thiol tripeptide and protect against the harmful effects of various endogenous stresses. Erastin can indirectly inactivate GPX4 by lowering GSH levels, leading to ROS accumulation and lipid peroxidation [1,4]. System
Figure 1: Mechanisms of ferroptosis. Erastin, sorafenib, sulfasalazine, etc., promote ferroptosis by inhibiting system
Ferroptosis-inducing agents (FINs) can be roughly divided into four main categories based on their modes of action: increasing intracellular labile iron pool (LIP); depleting GSH; depleting GPX4 and CoQ10 by the SQS-mevalonic acid pathway and targeted inactivation of GPX4 [6]. Recently, some new signaling pathways and regulatory factors have also been found to play a role in ferroptosis. A common example is the acyl CoA synthetase long-chain family member 4 (ACSL4) gene, which can regulate ferroptosis [7,8], Cell contact through the NF2-Hipo-Yap axis can resist ferroptosis caused by the cysteine starvation and GPX4 inactivation [9], and heat shock protein beta-1 (HSPB1) is a negative regulator of ferroptosis [10]. Interestingly, ferroptosis has been found to play an important role in cancer, acute renal failure, neurodegenerative diseases, diabetes, and other diseases. The emergence of ferroptosis has profoundly impacted various areas of life science, and a comprehensive understanding of the mechanism of ferroptosis will also help us solve existing diseases, especially cancer.
2 Ferroptosis and Genitourinary System Cancer
As the second leading cause of death worldwide, cancer threatens human health. Current cancer treatment methods are not very successful in eradicating cancer. However, many anticancer drugs have been used for clinical treatment and have also shown good therapeutic effects. However, serious problems exist, such as apoptosis avoidance, drug side effects, and drug resistance. The characteristics of iron addiction and ROS tolerance of cancer cells make them sensitive to ferroptosis. Recent evidence has shown that ferroptosis is related to cancer’s occurrence, progression, and drug resistance [11]. For example, metallothionein-1G knockout enhances the sensitivity of hepatocellular carcinoma to Sorafenib by promoting ferroptosis [12]. Furthermore, cancer of the genitourinary system has always been a serious threat to human life for both genders. Here, in this study, we will review the latest research advancement and progress of ferroptosis in the prostate, bladder, ovarian and cervical cancer, providing new approaches for treating related diseases.
2.2 Ferroptosis and Prostate Cancer
Prostate cancer (PCa) is the most common malignant tumor worldwide and is one of the three most common cancers in the United States [13]. About 10% of men who receive PCa treatment have metastasis [14]. Current metastatic disease treatment depends on androgen deprivation and androgen receptor (AR) regulation. However, androgen-targeted therapy usually fails in most patients with PCa and develops into castration-resistant prostate cancer (CRPC). Identifying more accurate markers is essential to improve the diagnosis and treatment of PCa [15] (Fig. 2). Based on the discovery of ferroptosis, Liao et al. [16] found that Pannexin 2 gene can be used as a new marker of ferroptosis and the malignant phenotype of prostate cancer cells. Pannexin 2 is necessary for the metastasis and invasion of the PCa cells. The expression of Pannexin 2 was significantly upregulated in the PCa tissues and cell lines as well, and it is considered to be an important factor affecting the severity of PCa. Therefore, Pannexin 2 can be used as a specific marker in patients with PCa. Knockdown of the Pannexin 2 results in increased intracellular iron divalent level and accumulation of malonaldehyde (MDA) which is a representative end-product of lipid peroxidation. The Nrf2 pathway plays a key role in ferroptosis. It was found that Pannexin 2 knockout can significantly reduce the mRNA and protein expression of Nrf2, as well as the expression of downstream genes (HO-1 and FTH1). Blocking the expression of Pannexin 2 can inhibit the proliferation, migration, and invasion of the PCa cells and increase the levels of ferrous iron and MDA. The Nrf2 activator oltipraz can restore the damage caused by Pannexin 2 knockout [16].
Figure 2: Ferroptosis and prostate cancer. Knockout of pannexin 2 and DECR1 induces ferroptosis; pannexin 2 knockout results in increased iron divalent ion and MDA levels, as well as decreased Nrf2 expression, thereby inhibiting HO-1 and FTH1 expression; Oltipraz can rescue the effect caused by pannexin 2 knockout; ITC-Ari-BSO not only acts on AR but also down-regulates GSH and promotes ferroptosis
Studies show that Pannexin 2 regulates the malignant phenotypes and ferroptosis of PCa cells via the Nrf2 pathway, and this target could be used as a novel treatment for PCa. If this approach is validated in vivo, Pannexin 2 could be used as the biomarker for early detection of PCa and a new method for treating PCa, which will ultimately benefit treating the disease. Nassar et al. [17] found that the DECR1 gene is upregulated in clinical prostate tumors, and DECR1 expression is closely related to the progression of PCa and the patient’s prognosis. By knocking out DECR1, lipid peroxidation and ferroptosis can be induced, and PCa proliferation and metastasis can be inhibited. For the treatment of CRPC, Qin et al. [18] designed and synthesized isothiocyanate (ITC) mixed AR antagonists (ITC-ARi). A reasonable combination of ITC-ARi and Buthionine sulfoximine (BSO), an inhibitor of GSH synthesis, could effectively down-regulate AR/AR splicing variants and induce ferroptosis in CRPC cells. This combination approach provides new ideas for the treatment of CRPC. Some studies have shown that the key factors regulating ferroptosis (GPX4, TFRC, FPN, Iron) may also play an important role in prostate cancer. Studies about androgen targeting therapy in prostate cancer have shown that GPX4 dependence and ferroptosis hypersensitivity in persister cells are associated with extensive lipid remodeling [19]. Overexpression of the transferrin receptor (TFRC) promotes the proliferation, migration, and invasion of the PCa cell lines [20]. Recombinant Ferroportin (FPN) inhibits the proliferation of prostate cancer cells by influencing both the iron efflux and cell cycle regulators [21]. At different stages of PCa (androgen-sensitive and insensitive), increasing iron content in the treatment strategies maximizes efficacy and may overcome resistance to the existing therapies [22]. It is important to note that Zhao et al. examined the cell survival of androgen-sensitive PCa cell line LNCaP and CRPC-like PCa cell line LNCAP-AL after treatment with the GPX4 inhibitor RSL3. They discovered that the former was more resistant to ferroptosis. And the activation of PLA2G4A by ATF6 controls this resistance. Based on this, they found that enzalutamide and the ATF6 inhibitor Ceapin-A7 can work together to halt the course of CRPC and trigger ferroptosis (2022) [23]. According to reports, apoptosis resistance is the fundamental mechanism of therapeutic resistance in PCa. Samy et al. discovered that diallyl trisulfide (DATS) could trigger ferroptosis targeting PCa and override apoptosis resistance [24]. Ferroptosis may be used to overcome the cancer cells’ resistance to apoptosis. Although studies on the regulation of ferroptosis in prostate cancer are frequently reported and showed a good therapeutic prospect, the real application of ferroptosis in clinical treatment needs both in vivo experiment validation and multi-demonstration.
Surprisingly, ferroptosis can predict disease-free survival (DFS) and immunotherapy response for PCa patients following radical prostatectomy in addition to killing cancer cells [25,26]. In addition, by comparing the data of whole genome patients, Li et al. [27], they were found that the characteristics of genomic changes in Chinese patients were significantly different from those in western populations, and the mutations or deletions of these genes may have other effects on the occurrence of ferroptosis. Therefore, accelerated research on the role of ferroptosis in prostate cancer will provide new strategies for treating prostate cancer patients and, hopefully, the radical treatment of prostate cancer.
2.3 Ferroptosis and Bladder Cancer
Bladder cancer (BCa) is one of the most common epithelial malignancies and the second most common urogenital malignancy globally [28]. In China, bladder cancer ranks first among male genitourinary malignancies. Depending on the extent of the tumor invasion and depth, BCa can be classified as either muscle-invasive bladder cancer (MIBC) or non-muscle-invasive bladder cancer (NMIBC). Radiation therapy, adjuvant immunotherapy, radical cystectomy (RC), and other options are available to patients with the former [29]. Intravesical chemotherapy and intravesical bacillus Calmette-Guérin (BCG) immunotherapy are two treatment options for NMIBC patients [30]. One of the primary treatments for BCa is cisplatin-based combination chemotherapy, but the effectiveness of this treatment has been severely limited due to the development of cisplatin resistance. In 2014, Drayton et al. [31] discovered a new mechanism of cisplatin resistance: Increased microRNA-27a expression mediates the intracellular glutathione regulation. Cisplatin resistance is mediated by the increased SLC7A11 expression and glutathione production. Overexpression of miRNA-27a reduces SLC7A11 and intracellular glutathione levels and re-sensitizes drug-resistant cells to cisplatin. Inhibition of SLC7A11 by sulfasalazine also re-sensitized the cisplatin-resistant bladder cancer cells. Both glutathione and SLC7A11 are also considered regulatory factors in ferroptosis. Although Drayton RM did not directly associate this result with the ferroptosis, FINs could inhibit SLC7A11 function and reduce the GSH production. Thus, we speculated that FINs might also play a similar role in cisplatin resistance in bladder cancer. However, this needs further experimental proof, and this method may be a new way to treat bladder cancer. Currently, there is no direct report on how ferroptosis regulates bladder cancer, but current studies show that ferroptosis-related factors play an important role in bladder cancer. For example, reduced serum iron ion levels may be an important cause of bladder cancer [32]. The expression of MIR497HG inhibits the growth, migration, and invasion of BCa cells in vitro by affecting Hippo/YAP and TGF-β/Smad signaling pathways [33]. Verteporfin inhibits YAP-induced bladder cancer cell growth and invasion through the Hippo signaling pathway [34]. Genetic variation in the GSH pathway may affect cancer recurrence in non-muscle-invasive bladder cancer patients [35]. Inhibiting the expression of YAP and Nrf2 can affect the migrative ability of drug-resistant bladder cancer cells and significantly improves the sensitivity to cisplatin [36]. At the same time, an accurate cancer prognosis can improve the survival rate of patients. Zhou et al. developed a bladder cancer risk model based on ferroptosis, which can help clinicians evaluate the prognosis, immunotherapy outcome, and chemotherapy response of patients with bladder cancer [37]. Xia et al. developed a scoring system based on ferroptosis to predict the prognosis of BCa patients and is closely connected to TNM classification, tumor grade, and other clinical characteristics [38]. There are numerous reports about the prediction of BCa at this time [39–42]. Treatment of bladder cancer benefits from knowledge of the connection between ferroptosis and bladder cancer. In addition to paying close attention to the prognosis of ferroptosis on BCa, we should also investigate the molecular mechanism of ferroptosis in the therapy of BCa.
2.4 Ferroptosis and Ovarian Cancer
Ovarian cancer is one of the most common cancers in women and is a highly fatal gynaecological malignant tumor worldwide [43]. Clinically, ovarian cancer exhibits a deceptive beginning. Most ovarian cancer patients pass away from advanced disease because there are few reliable symptoms and biomarkers at an early stage. One of the current conventional therapies for advanced ovarian cancer is platinum-based chemotherapy. Since ferroptosis was reported, many studies have shown a strong link between iron death and ovarian cancer (Fig. 3). In 2017, Basuli et al. [44] found iron “addiction” is a new target in ovarian cancer treatment. The increase of iron efflux can inhibit the proliferation of ovarian cancer cells and reduce the tumor load and metastasis of ovarian cancer in vivo. They noted that ovarian cancer stem cells are sensitive to both the ferroptosis-inducing drugs and iron-chelating agents, providing a new target for ovarian cancer treatment. Cisplatin is the most effective anticancer drug, but it has significant limitations due to drug resistance of tumor cells and toxic side effects caused by high drug use. Chan et al. [45] found that MAP30 isolated from the bitter gourd seeds can also act as a FIN for ovarian cancer cells. MAP30 can enhance lipid peroxidation by inhibiting the GPX4, reducing GSH/GSSG ratio, and increasing ROS quantity. In addition, MAP30 can inhibit the migration, invasion, and proliferation of cancer cells in various ovarian cancer cells without producing adverse reactions. It can also enhance the sensitivity of cancer cells to cisplatin when combined with cisplatin. Meanwhile, Cheng et al. [46] showed that Erastin, in conjunction with cisplatin, inhibits ovarian cancer growth via ferroptosis in vivo and in vitro. In particular, HE staining of tissue sections showed a low degree of organ injury upon cisplatin and Erastin combination therapy. The combination therapy induces the increased ACSL4 and COX-2 expression in HEY cells and significantly inhibits the GPX4 and FTH1 expression. Although this is an effective therapeutic approach, Erastin cannot be used directly in the clinic as it is metabolically unstable in vivo. Therefore, searching for Erastin analogues or alternative compounds will open new avenues for the clinical treatment of ovarian cancer worldwide. In 2019, Tesfay et al. [47] found that stearoyl-CoA-desaturase-1 (SCD1) is highly expressed in ovarian cancer; blocking SCD1 in vitro and in vivo can regulate lipid metabolism and make ovarian cancer cells sensitive to the FINs. In addition, researchers found that SLC7A11 is a key ferroptosis factor that significantly upregulated ovarian cancer cells. Sun et al. [48] reported that cocaine extract lidocaine promotes ferroptosis in ovarian cancer cells via targeting the miR-382-5p/SLC7A11 axis. Lidocaine upregulates miR-382-5p and subsequently inhibits the SLC7A11 to induce ferroptosis. However, due to the lack of direct evidence and model validation of the effect of lidocaine on ferroptosis, its clinical value in the treatment of ovarian cancer needs to be demonstrated in detail. Drug inhibition of Poly ADP-Ribose polymerases (PARP) is the main treatment strategy for breast cancer (BRCA) mutant ovarian cancer. Hong et al. [49] found that PARP inhibition promotes ferroptosis by inhibiting the SLC7A11. They demonstrated that the PARP, in combination with FINs, can sensitize BRCA-proficient ovarian cancer cells to PARP inhibitor olaparib, which provides a promising treatment strategy for using PARP inhibitors in BRCA-proficient ovarian cancer. Jin et al. discovered that SNAI2 knockdown would reduce the production of SLC7A11 protein and enhance the occurrence of ferroptosis, which can decrease the advancement of ovarian cancer. SNAI2 is a zinc finger protein that encodes the SNAI transcription factor family. The authors discovered that SNAI2 could bind to the GPX4 promoter as well as the SLC7A11 promoter at the same time. However, they did not specify specifically how these two promoters were related [50]. Inhibiting the growth of ovarian cancer and promoting ferroptosis may be the dual targets of SNAI2. Cai colleagues. Discovered that the lncRNA ADAMTS9-AS1 attenuates ferroptosis in epithelial ovarian cancer by inhibiting the miR-587/SLC7A11 axis [51]. These data suggest that SLC7A11 may significantly influence the relationship between ferroptosis and ovarian cancer. Cancer cells avoid ferroptosis through genetic regulation of the expression of related proteins. Recently, You et al. [52] constructed an ovarian cancer scoring system based on ferroptosis-related gene expression and discovered a potential drug, DMOG, which can help doctors predict tumor progression. To maintain their ability to increase, ovarian cancer cells appear to have bidirectional resistance to ferroptosis and apoptosis. An important treatment approach is to influence the death mechanism of cancer cells in multiple directions. Further research into the connection between ferroptosis and ovarian cancer is extremely important.
Figure 3: Ferroptosis and ovarian cancer. MAP30 inhibits GPX4, increases ROS, enhances lipid peroxidation, and leads to ferroptosis. MAP30 combined with cisplatin can improve the sensitivity of cancer cells to cisplatin; PARP inhibition promotes ferroptosis by inhibiting the SLC7A11; ADAMTS9-AS1 inhibited ferroptosis by the miR-587/SLC7A11 axis; Lidocaine upregulates the miR-382-5p, inhibits SLC7A11, and promotes ferroptosis; Cisplatin combined with the erastin increases ACSL4 and COX-2 expression and decreases GPX4 and FTH1 expression
2.5 Ferroptosis and Cervical Cancer
Currently, cervical cancer remains the leading cause of cancer-related death in women worldwide. Persistent infection with high-risk human papillomavirus (HR-HPV) is the primary cause of cervical cancer [53]. There are more than 200 types of HPV, but only 52 types can cause cancer development. According to clinical data and related studies, HPV types 16 and 18 are closely associated with the occurrence of cervical cancer. Although chemotherapy, radiotherapy, and surgery are available, the survival rate is quite low due to tumor recurrence, metastasis, and drug resistance. The discovery of ferroptosis provides a new target for the treatment of cervical cancer (Fig. 4). Wu et al. [54] found that the expression level of circEPSTI1 was high in cervical cancer cells, and the knockout of this gene could inhibit the proliferation of cervical cancer cells. By silencing circEPSTI1, it was found that the ratio of GSH/GSSG and the expression of GPX4 were decreased, and the accumulation of lipid peroxides on the cell membrane is increased in ferroptosis, which was caused by the inhibition of the SLC7A11 expression. This is the first time circRNAs are associated with ferroptosis, providing a new target for treating cervical cancer. Xiaofei et al. [55] also found that oleanolic acid (OA), a substance naturally existing in leaves, fruits, and roots of plants, inhibits the proliferation of cervical cancer cells by regulating the ACSL4 ferroptosis signaling pathway. ACSL4-mediated activation of ferroptosis reduces the size of cervical cancer tumors and the viability of Hela cells. At the same time, OA, as a natural activator of ACSL4, may enhance its anticancer effect in cervical cancer. This study suggests that OA may be a potential anticancer drug for cervical cancer. According to research on Sanguinarine (SNG) by Alakkal et al. [56], SNG caused ferroptosis through SL7A11 and apoptosis through caspase-3 in human cervical carcinoma. A second death mechanism was likewise blocked by the administration of an RCD inhibitor [56]. This raises the question of whether cancer cells’ regulation of H2O2 is what leads to medication resistance. To address the issue of treatment resistance, it is crucial to study how cancer cells control H2O2. Ferroptosis, however, does not always have an anti-tumor impact. According to Wang et al. [57], an anti-ferroptosis impact would be triggered by prolonged ferroptosis. This effect raises the Kirsten rat sarcoma viral oncogene homolog (KRAS), which accelerates the development of squamous cell carcinoma (SCC) [57]. Additionally, Liu et al. discovered that miR-193a-5p/GPX4 and circular RNA circACAP2 prevented ferroptosis in cervical cancer’s malignant progression [58]. An important development in the fight against tumor treatment resistance is discovering the relationship between ferroptosis and apoptosis. The impact of genes associated with ferroptosis in determining the prognosis of cervical cancer has also been found in related investigations [59,60]. The use of ferroptosis in diagnosing and treating.
Figure 4: Ferroptosis and cervical cancer. circEPSTI1 silencing reduces the expression of SLC7A11, GSH/GSSG, and GPX4 and promotes ferroptosis; circACAP2 prevented ferroptosis by the miR-193a-5p/GPX4 axis; Oleanolic acid can increase the ACSL4 and TFR1 expression and induces ferroptosis; SNG caused ferroptosis and apoptosis
Cell death plays an important role in normal development, homeostasis, and prevention of hyperproliferative diseases. With the discovery of ferroptosis’s role in the treatment of human diseases, the ferroptosis mechanism has attracted more and more attention from researchers, and new FINs have gradually entered people’s vision, such as iron-based nanomaterials based on nanotechnology (iron oxide nanoparticles, FePt nanoparticles, etc.) and indirect iron-based nanomaterials. Iron oxide nanoparticles have been found to kill cancer cells by increasing iron and ROS levels. Cancer is scary because it has many biological characteristics, such as autonomy, aggressiveness, metastasis, abnormal differentiation, loss of contact inhibition, etc. Since cancer cells are sensitive to iron, inducing ferroptosis in the cancer cells by the ferroptosis mechanism will be a new way of cancer treatment. In addition, transfection key factors such as P53 and ACSL4 into tumor cells can also be used for cancer treatment. Ferroptosis opens a new avenue for treating malignant tumors of genitourinary system cancer, including prostate, bladder, ovarian and cervical cancers, which seriously threaten mental and physical health. Combining ferroptosis with traditional cancer treatments to trigger multimodal cancer cell death or finding new cancer therapies based on ferroptosis is expected to make a breakthrough in the current clinical cancer treatment.
Authorship: The authors confirm their contribution to the paper as follows: study conception and design: Chaoying Liu, Xinfeng Yang, Ye Wang; data collection: Ye Wang, Keyu Wu, Siqiang Li, Gailing Wang, Yun Li; analysis and interpretation of results: Chaoying Liu, Xinfeng Yang, Ye Wang, Chuanfeng Li, Mingcheng Wang, Enzhong Li; draft manuscript preparation: Chaoying Liu, Xinfeng Yang. All authors reviewed the results and approved the final version of the manuscript.
Ethics Approval and Informed Consent Statement: Not applicable.
Acknowledgement: Thanks to the two co-authors and other authors for their hard work on this review; Thanks for the financial support provided by the National Natural Science Foundation of China and Key Scientific Research Projects of Higher Education Institutions in Henan Province.
Funding Statement: This work was supported by the National Natural Science Foundation of China [Grant No. 31960692, Chaoying Liu]; 2022 Key Scientific Research Projects of Higher Education Institutions in Henan Province [Grant No. 22B230008, Chaoying Liu].
Conflicts of Interest: The authors declare that they have no conflicts of interest to report regarding the present study.
References
1. Dixon, S. J., Lemberg, K. M., Lamprecht, M. R., Skouta, R., Zaitsev, E. M. et al. (2012). Ferroptosis: An iron-dependent form of nonapoptotic cell death. Cell, 149(5), 1060–1072. DOI 10.1016/j.cell.2012.03.042. [Google Scholar] [CrossRef]
2. Alvarez, S. W., Sviderskiy, V. O., Terzi, E. M., Papagiannakopoulos, T., Moreira, A. L. et al. (2017). NFS1 undergoes positive selection in lung tumours and protects cells from ferroptosis. Nature, 551(7682), 639–643. DOI 10.1038/nature24637. [Google Scholar] [CrossRef]
3. Yang, W. S., SriRamaratnam, R., Welsch, M. E., Shimada, K., Skouta, R. et al. (2014). Regulation of ferroptosis cancer cell death by GPX4. Cell, 156(1–2), 317–331. DOI 10.1016/j.cell.2013.12.010. [Google Scholar] [CrossRef]
4. Dixon, S. J., Stockwell, B. R. (2014). The role of iron and reactive oxygen species in cell death. Nature Chemical Biology, 10(1), 9–17. DOI 10.1038/nchembio.1416. [Google Scholar] [CrossRef]
5. Bannai, S. (1986). Exchange of cystine and glutamate across the plasma membrane of human fibroblasts. The Journal of Biological Chemistry, 261(5), 2256–2263. DOI 10.1016/S0021-9258(17)35926-4. [Google Scholar] [CrossRef]
6. Feng, H., Stockwell, B. R. (2018). Mysteries: How does lipid peroxidation cause ferroptosis? PLoS Biology, 16(5), e2006203. DOI 10.1371/journal.pbio.2006203. [Google Scholar] [CrossRef]
7. Yuan, H., Li, X., Zhang, X., Kang, R., Tang, D. (2016). Identification of ACSL4 as a biomarker and contributor of ferroptosis. Biochemical and Biophysical Research Communications, 478(3), 1338–1343. DOI 10.1016/j.bbrc.2016.08.124. [Google Scholar] [CrossRef]
8. Doll, S., Proneth, B., Tyurina, Y. Y., Panzilius, E., Kobayashi S. et al. (2017). ACSL4 dictates ferroptosis sensitivity by shaping cellular lipid composition. Nature Chemical Biology, 13(1), 91–98. DOI 10.1038/nchembio.2239. [Google Scholar] [CrossRef]
9. Wu, J., Minikes, A. M., Gao, M., Bian, H., Li, Y. et al. (2019). Intercellular interaction dictates cancer cell ferroptosis via NF2-YAP signalling. Nature, 572(7769), 402–406. DOI 10.1038/s41586-019-1426-6. [Google Scholar] [CrossRef]
10. Sun, X., Ou, Z., Xie, M., Kang, R., Fan, Y. et al. (2015). HSPB1 as a novel regulator of ferroptosis cancer cell death. Oncogene, 34(45), 5617–5625. DOI 10.1038/onc.2015.32. [Google Scholar] [CrossRef]
11. Su, Y., Zhao, B., Zhou, L., Zhang, Z., Shen, Y. et al. (2020). Ferroptosis, a novel pharmacological mechanism of anticancer drugs. Cancer Letters, 483, 127–136. DOI 10.1016/j.canlet.2020.02.015. [Google Scholar] [CrossRef]
12. Sun, X., Niu, X., Chen, R., He, W., Chen, D. et al. (2016). Metallothionein-1G facilitates sorafenib resistance through the inhibition of ferroptosis. Hepatology, 64(2), 488–500. DOI 10.1002/hep.28574. [Google Scholar] [CrossRef]
13. Siegel, R. L., Miller, K. D., Fuchs, H. E., Jemal, A. (2021). Cancer statistics, 2021. CA: A Cancer Journal for Clinicians, 71(1), 7–33. DOI 10.3322/caac.21654. [Google Scholar] [CrossRef]
14. Cornford, P., Bellmunt, J., Bolla, M., Briers, E., de Santis, M. et al. (2017). EAU-ESTRO-SIOG guidelines on prostate cancer. Part II: Treatment of relapsing, metastatic, and castration-resistant prostate cancer. European Urology, 71(4), 630–642. DOI 10.1016/j.eururo.2016.08.002. [Google Scholar] [CrossRef]
15. Zhou, J. G., Zhao, H. T., Jin, S. H., Tian, X., Ma, H. (2019). Identification of an RNA-seq-based signature to improve prognostics for uterine sarcoma. Gynecologic Oncology, 155(3), 499–507. DOI 10.1016/j.ygyno.2019.08.033. [Google Scholar] [CrossRef]
16. Liao, D., Yang, G., Yang, Y., Tang, X., Huang, H. et al. (2020). Identification of pannexin 2 as a novel marker correlating with ferroptosis and malignant phenotypes of prostate cancer cells. OncoTargets and Therapy, 13, 4411–4421. DOI 10.2147/OTT.S249752. [Google Scholar] [CrossRef]
17. Nassar, Z. D., Mah, C. Y., Dehairs, J., Burvenich, I. J., Irani, S. et al. (2020). Human DECR1 is an androgen-repressed survival factor that regulates PUFA oxidation to protect prostate tumor cells from ferroptosis. eLife, 9, e54166. DOI 10.7554/eLife.54166. [Google Scholar] [CrossRef]
18. Qin, Z., Ou, S., Xu, L., Sorensen, K., Zhang, Y. et al. (2021). Design and synthesize isothiocyanate-containing hybrid androgen receptor (AR) antagonist to downregulate AR and induce ferroptosis in GSH-deficient prostate cancer cells. Chemical Biology & Drug Design, 97(5), 1059–1078. DOI 10.1111/cbdd.13826. [Google Scholar] [CrossRef]
19. Tousignant, K. D., Rockstroh, A., Poad, B., Talebi, A., Young, R. et al. (2020). Therapy-induced lipid uptake and remodeling underpin ferroptosis hypersensitivity in prostate cancer. Cancer & Metabolism, 8, 11. DOI 10.1186/s40170-020-00217-6. [Google Scholar] [CrossRef]
20. Liu, H., Gao, L., Xie, T., Li, J., Zhai, T. S. et al. (2021). Identifying and validating a prognostic signature for prostate cancer based on ferroptosis-related genes. Frontiers in Oncology, 11, 623313. DOI 10.3389/fonc.2021.623313. [Google Scholar] [CrossRef]
21. Deng, Z., Manz, D. H., Torti, S. V., Torti, F. M. (2019). Effects of ferroportin-mediated iron depletion in cells representative of different histological subtypes of prostate cancer. Antioxidants & Redox Signaling, 30(8), 1043–1061. DOI 10.1089/ars.2017.7023. [Google Scholar] [CrossRef]
22. Bordini, J., Morisi, F., Elia, A. R., Santambrogio, P., Pagani, A. et al. (2020). Iron induces cell death and strengthens the efficacy of antiandrogen therapy in prostate cancer models. Clinical Cancer Research, 26(23), 6387–6398. DOI 10.1158/1078-0432.CCR-20-3182. [Google Scholar] [CrossRef]
23. Zhao, R., Lv, Y., Feng, T., Zhang, R., Ge, L. et al. (2022). ATF6α promotes prostate cancer progression by enhancing PLA2G4A-mediated arachidonic acid metabolism and protecting tumor cells against ferroptosis. The Prostate, 82(5), 617–629. DOI 10.1002/pros.24308. [Google Scholar] [CrossRef]
24. Samy, A., Shah, D., Shahagadkar, P., Shah, H., Munirathinam, G. (2022). Can diallyl trisulfide, a dietary garlic-derived compound, activate ferroptosis to overcome therapy resistance in prostate cancer? Nutrition and Health, 28(2), 207–212. DOI 10.1177/02601060211018360. [Google Scholar] [CrossRef]
25. Wang, Y., Fan, J., Chen, T., Xu, L., Liu, P. et al. (2022). A novel ferroptosis-related gene prognostic index for prognosis and response to immunotherapy in patients with prostate cancer. Frontiers in Endocrinology, 13, 975623. DOI 10.3389/fendo.2022.975623. [Google Scholar] [CrossRef]
26. Ji, J., Li, H., Wang, W., Yuan, B., Shen, T. (2022). STAT3 regulates ARPC1A to inhibit ferroptosis and promote prostate cancer progression. Human Cell, 35(5), 1591–1601. DOI 10.1007/s13577-022-00754-w. [Google Scholar] [CrossRef]
27. Li, J., Xu, C., Lee, H. J., Ren, S., Zi, X. et al. (2020). A genomic and epigenomic atlas of prostate cancer in asian populations. Nature, 580(7801), 93–99. DOI 10.1038/s41586-020-2135-x. [Google Scholar] [CrossRef]
28. Sanli, O., Dobruch, J., Knowles, M. A., Burger, M., Alemozaffar, M. et al. (2017). Bladder cancer. Nature Reviews. Disease Primers, 3, 17022. DOI 10.1038/nrdp.2017.22. [Google Scholar] [CrossRef]
29. Witjes, J. A., Bruins, H. M., Cathomas, R., Compérat, E. M., Cowan, N. C. et al. (2021). European association of urology guidelines on muscle-invasive and metastatic bladder cancer: Summary of the 2020 guidelines. European Urology, 79(1), 82–104. DOI 10.1016/j.eururo.2020.03.055. [Google Scholar] [CrossRef]
30. Sylvester, R. J., Rodríguez, O., Hernández, V., Turturica, D., Bauerová, L. et al. (2021). European association of urology (EAU) prognostic factor risk groups for non-muscle-invasive bladder cancer (NMIBC) incorporating the WHO 2004/2016 and WHO 1973 classification systems for grade: An update from the EAU NMIBC guidelines panel. European Urology, 79(4), 480–488. DOI 10.1016/j.eururo.2020.12.033. [Google Scholar] [CrossRef]
31. Drayton, R. M., Dudziec, E., Peter, S., Bertz, S., Hartmann, A. et al. (2014). Reduced expression of miRNA-27a modulates cisplatin resistance in bladder cancer by targeting the cystine/glutamate exchanger SLC7A11. Clinical Cancer Research, 20(7), 1990–2000. DOI 10.1158/1078-0432.CCR-13-2805. [Google Scholar] [CrossRef]
32. Mazdak, H., Yazdekhasti, F., Movahedian, A., Mirkheshti, N., Shafieian, M. (2010). The comparative study of serum iron, copper, and zinc levels between bladder cancer patients and a control group. International Urology and Nephrology, 42(1), 89–93. DOI 10.1007/s11255-009-9583-4. [Google Scholar] [CrossRef]
33. Zhuang, C., Liu, Y., Fu, S., Yuan, C., Luo, J. et al. (2021). Corrigendum: Silencing of lncRNA MIR497HG via CRISPR/cas13d induces bladder cancer progression through promoting the crosstalk between hippo/Yap and TGF-β/Smad signaling. Frontiers in Molecular Biosciences, 8, 664616. DOI 10.3389/fmolb.2021.664616. [Google Scholar] [CrossRef]
34. Dong, L., Lin, F., Wu, W., Liu, Y., Huang, W. (2018). Verteporfin inhibits YAP-induced bladder cancer cell growth and invasion via hippo signaling pathway. International Journal of Medical Sciences, 15(6), 645–652. DOI 10.7150/ijms.23460. [Google Scholar] [CrossRef]
35. Ke, H. L., Lin, J., Ye, Y., Wu, W. J., Lin, H. H. et al. (2015). Genetic variations in glutathione pathway genes predict cancer recurrence in patients treated with transurethral resection and bacillus calmette-guerin instillation for non-muscle invasive bladder cancer. Annals of Surgical Oncology, 22(12), 4104–4110. DOI 10.1245/s10434-015-4431-5. [Google Scholar] [CrossRef]
36. Ciamporcero, E., Daga, M., Pizzimenti, S., Roetto, A., Dianzani, C. et al. (2018). Crosstalk between Nrf2 and YAP contributes to maintaining the antioxidant potential and chemoresistance in bladder cancer. Free Radical Biology & Medicine, 115, 447–457. DOI 10.1016/j.freeradbiomed.2017.12.005. [Google Scholar] [CrossRef]
37. Zhou, R., Liang, J., Tian, H., Chen, Q., Yang, C. et al. (2021). Developing a ferroptosis-related lncRNA signature to predict the prognosis and immune landscape of bladder cancer. Disease Markers, 2021, 1031906. DOI 10.1155/2021/1031906. [Google Scholar] [CrossRef]
38. Xia, Q. D., Sun, J. X., Liu, C. Q., Xu, J. Z., An, Y. et al. (2022). Ferroptosis patterns and tumor microenvironment infiltration characterization in bladder cancer. Frontiers in Cell and Developmental Biology, 10, 832892. DOI 10.3389/fcell.2022.832892. [Google Scholar] [CrossRef]
39. Wang, Y., Shao, W., Feng, Y., Tang, J., Wang, Q. et al. (2022). Prognostic value and potential biological functions of a ferroptosis-related gene signature in bladder cancer. Oncology Letters, 24(3), 301. DOI 10.3892/ol.2022.13421. [Google Scholar] [CrossRef]
40. Wang, Y., Zhang, S., Bai, Y., Li, G., Wang, S. et al. (2022). Development and validation of ferroptosis-related LncRNA biomarker in bladder carcinoma. Frontiers in Cell and Developmental Biology, 10, 809747. DOI 10.3389/fcell.2022.809747. [Google Scholar] [CrossRef]
41. Shen, L., Zhang, J., Zheng, Z., Yang, F., Liu, S. et al. (2022). PHGDH inhibits ferroptosis and promotes malignant progression by upregulating SLC7A11 in bladder cancer. International Journal of Biological Sciences, 18(14), 5459–5474. DOI 10.7150/ijbs.74546. [Google Scholar] [CrossRef]
42. Yang, Y., Hu, H., Chen, L., Zhang, H., Yang, J. (2022). A new survival model based on ferroptosis-related genes (FRGS) for prognostic prediction in bladder cancer. Actas Urológicas Españolas, 46(8), 494–503. DOI 10.1016/j.acuroe.2022.06.001. [Google Scholar] [CrossRef]
43. Książek, K. (2022). Where does cellular senescence belong in the pathophysiology of ovarian cancer? Seminars in Cancer Biology, 81, 14–23. DOI 10.1016/j.semcancer.2020.11.021. [Google Scholar] [CrossRef]
44. Basuli, D., Tesfay, L., Deng, Z., Paul, B., Yamamoto, Y. et al. (2017). Iron addiction: A novel therapeutic target in ovarian cancer. Oncogene, 36(29), 4089–4099. DOI 10.1038/onc.2017.11. [Google Scholar] [CrossRef]
45. Chan, D. W., Yung, M. M., Chan, Y. S., Xuan, Y., Yang, H. et al. (2020). MAP30 protein from momordica charantia is therapeutic and has synergic activity with cisplatin against ovarian cancer in vivo by altering metabolism and inducing ferroptosis. Pharmacological Research, 161, 105157. DOI 10.1016/j.phrs.2020.105157. [Google Scholar] [CrossRef]
46. Cheng, Q., Bao, L., Li, M., Chang, K., Yi, X. (2021). Erastin synergizes with cisplatin via ferroptosis to inhibit ovarian cancer growth in vitro and in vivo. The Journal of Obstetrics and Gynaecology Research, 47(7), 2481–2491. DOI 10.1111/jog.14779. [Google Scholar] [CrossRef]
47. Tesfay, L., Paul, B. T., Konstorum, A., Deng, Z., Cox, A. O. et al. (2019). Stearoyl-CoA desaturase 1 protects ovarian cancer cells from ferroptotic cell death. Cancer Research, 79(20), 5355–5366. DOI 10.1158/0008-5472.CAN-19-0369. [Google Scholar] [CrossRef]
48. Sun, D., Li, Y. C., Zhang, X. Y. (2021). Lidocaine promoted ferroptosis by targeting miR-382-5p/SLC7A11 axis in ovarian and breast cancer. Frontiers in Pharmacology, 12, 681223. DOI 10.3389/fphar.2021.681223. [Google Scholar] [CrossRef]
49. Hong, T., Lei, G., Chen, X., Li, H., Zhang, X. et al. (2021). PARP inhibition promotes ferroptosis via repressing SLC7A11 and synergizes with ferroptosis inducers in BRCA-proficient ovarian cancer. Redox Biology, 42, 101928. DOI 10.1016/j.redox.2021.101928. [Google Scholar] [CrossRef]
50. Jin, Y., Chen, L., Li, L., Huang, G., Huang, H. et al. (2022). SNAI2 promotes the development of ovarian cancer by regulating ferroptosis. Bioengineered, 13(3), 6451–6463. DOI 10.1080/21655979.2021.2024319. [Google Scholar] [CrossRef]
51. Cai, L., Hu, X., Ye, L., Bai, P., Jie, Y. et al. (2022). Long non-coding RNA ADAMTS9-AS1 attenuates ferroptosis by targeting microRNA-587/solute carrier family 7 members 11 axis in epithelial ovarian cancer. Bioengineered, 13(4), 8226–8239. DOI 10.1080/21655979.2022.2049470. [Google Scholar] [CrossRef]
52. You, Y., Fan, Q., Huang, J., Wu, Y., Lin, H. et al. (2021). Ferroptosis-related gene signature promotes ovarian cancer by influencing immune infiltration and invasion. Journal of Oncology, 2021, 9915312. DOI 10.1155/2021/9915312. [Google Scholar] [CrossRef]
53. Lei, J., Ploner, A., Elfström, K. M., Wang, J., Roth, A. et al. (2020). HPV vaccination and the risk of invasive cervical cancer. The New England Journal of Medicine, 383(14), 1340–1348. DOI 10.1056/NEJMoa1917338. [Google Scholar] [CrossRef]
54. Wu, P., Li, C., Ye, D. M., Yu, K., Li, Y. et al. (2021). Circular RNA circEPSTI1 accelerates cervical cancer progression via miR-375/409-3P/515-5p-SLC7A11 axis. Ageing, 13(3), 4663–4673. DOI 10.18632/aging.202518. [Google Scholar] [CrossRef]
55. Jiang, X. F., Shi, M., Q., Sui, M., Yuan, Y. Z., Zhang, S. et al. (2021). Oleanolic acid inhibits cervical cancer hela cell proliferation through modulation of the ACSL4 ferroptosis signaling pathway. Biochemical and Biophysical Research Communications, 545, 81–88. DOI 10.1016/j.bbrc.2021.01.028. [Google Scholar] [CrossRef]
56. Alakkal, A., Thayyullathil, F., Pallichankandy, S., Subburayan, K., Cheratta, A. R. et al. (2022). Sanguinarine induces H2O2-dependent apoptosis and ferroptosis in human cervical cancer. Biomedicines, 10(8), 1795. DOI 10.3390/biomedicines10081795. [Google Scholar] [CrossRef]
57. Wang, T., Gong, M., Cao, Y., Zhao, C., Lu, Y. et al. (2022). Persistent ferroptosis promotes cervical squamous intraepithelial lesion development and oncogenesis by regulating KRAS expression in high-risk-HPV infection patients. Cell Death Discovery, 8(1), 201. DOI 10.1038/s41420-022-01013-5. [Google Scholar] [CrossRef]
58. Liu, Y., Li, L., Yang, Z., Wen, D., Hu, Z. (2022). Circular RNA circACAP2 suppresses ferroptosis of cervical cancer during malignant progression by miR-193a-5p/GPX4. Journal of Oncology, 2022, 5228874. DOI 10.1155/2022/5228874. [Google Scholar] [CrossRef]
59. Yang, X., Yin, F., Liu, Q., Ma, Y., Zhang, H. et al. (2022). Ferroptosis-related genes identify tumor immune microenvironment characterization for predicting prognosis in cervical cancer. Annals of Translational Medicine, 10(2), 123. DOI 10.21037/atm-21-6265. [Google Scholar] [CrossRef]
60. Jiang, Z., Li, J., Feng, W., Sun, Y., Bu, J. (2022). A ferroptosis-related lncRNA model to enhance the predicted value of cervical cancer. Journal of Oncology, 2022, 6080049. DOI 10.1155/2022/6080049. [Google Scholar] [CrossRef]
Cite This Article
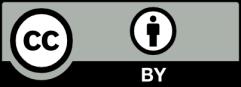
This work is licensed under a Creative Commons Attribution 4.0 International License , which permits unrestricted use, distribution, and reproduction in any medium, provided the original work is properly cited.