Open Access
ARTICLE
The Neurosurgical Challenge of Primary Central Nervous System Lymphoma Diagnosis: A Multimodal Intraoperative Imaging Approach to Overcome Frameless Neuronavigated Biopsy Sampling Errors
1
Division of Neurosurgery, Department of Neurosciences, Policlinico “G. Rodolico-S. Marco”, University Hospital, Catania, 95123, Italy
2
PhD Program at Department of Neuroscience, University of Turin, Turin, 10100, Italy
3
Department of Radiodiagnostic and Oncological Radiotherapy, University Hospital Policlinico-Vittorio Emanuele, Catania, 95123, Italy
4
Department of Medical, Surgical Sciences and Advanced Technologies “G.F. Ingrassia”, Anatomic Pathology, University of Catania,
Catania, 95123, Italy
* Corresponding Author: Roberto Altieri. Email:
Oncologie 2022, 24(4), 693-706. https://doi.org/10.32604/oncologie.2022.025393
Received 08 August 2022; Accepted 30 September 2022; Issue published 31 December 2022
Abstract
Background: Intracranial lymphoma remains a challenging differential diagnosis in daily neurosurgical practice. We analyzed our early experience with a surgical series of frameless neuronavigated biopsies in Primary CNS Lymphomas (PCNSLs), highlighting the importance of using an intraoperative combined imaging protocol (5-ALA fluorescence, i-CT and 11C-MET-PET) to overcome potential targeting errors secondary to tumor volume reduction after corticosteroid therapy. Materials and Methods: All patients treated for PCNLSs at our center in a 24-month period (1/1/2019 to 31/12/2020) were analyzed. Our cohort included 6 patients (4 males), with a median age of 67 years (59–82). A total of 45 samples were evaluated for correlation between intraoperative fluorescence and pathological findings. 39 samples biopsy were evaluated. Results: 54% samples showed a clear diagnosis of PCNSL. 64% of samples had a lava like fluorescence. i-CT scan showed tumor volume changes, in comparison to preoperative MRI, in all cases and helped in planning correct trajectories on updated imaging; after biopsy i-CT confirmed sampling accuracy and excluded procedural complications in all cases. Conclusions: We believe that the use of a multimodal intraoperative imaging approach overcomes the demonstrated PCNSL morphological changes caused by corticosteroid therapy and gives a reliable tissue diagnosis by frameless biopsy.Keywords
Intracranial lymphoma remains a challenging differential diagnosis in daily neurosurgical practice. Primary Central Nervous System Lymphomas (PCNSLs) are extra-nodal, non-Hodgkin lymphomas (NHLs), involving brain, eyes, leptomeninges and, rarely, the spinal cord without systemic involvement. PCNSLs account for 1%–3% of all NHLs and about 3% of all primary brain tumors [1]. Approximately 95% of all PCNSLs are diffuse large B-cell lymphomas, with the remaining 5% being T-cell, Burkitt, lymphoblastic, and marginal zone lymphomas [2].
PCNSL clinical onset is commonly subacute, with progressive cognitive decline or personality changes, confusion, focal neurological deficits, headache and/or nausea and vomiting [3]. Because of the presence of neurological symptoms and the difficult differential diagnosis, these patients are often treated with corticosteroids that may induce a rapid clinical improvement as well as a radiologically documented tumor reduction, thus increasing the risk (and the rate) of non-diagnostic biopsies.
In previous studies we proved the safety and efficacy of combining several intraoperative tools to overcome surgical pitfalls related to navigation based on preoperative imaging and to improve the extent of brain tumor resection [4–8]. The aim of this paper was to analyze our early experience with a surgical series of frameless neuronavigated biopsies in PCNSLs, highlighting the importance of having an intraoperative, multimodal imaging protocol to compensate for tumor volume reduction or tumor shape changes after corticosteroid therapy, which may affect the accuracy of tissue targeting and sampling.
The study was performed in accordance with the 1964 Declaration of Helsinki and approved by the Catania 1 Ethics Committee, Santa Sofia 78 street, Catania, Italy (protocol code: 166/2015/PO; 17/12/2015); the non-interventional retrospective nature of our study did not require any informed consent by the local research ethics committee. We analyzed patients treated for PCNLSs at our center in a 24-month period (1/1/2019 to 31/12/2020). 45 samples of 6 patients (4 males) with histologically proven PCNSLs operated using the intraoperative multimodal imaging protocol were analyzed; the median age was 67 years (59–82). For each patient, anonymized data were extracted: age, gender, preoperative MRI, preoperative 11C-methionine-positron emission tomography (11C-MET-PET) and intraoperative (i-CT) tumor volumes, 5-ALA fluorescence (strong, vague, none), days of preoperative corticosteroid therapies, clinical and neuroradiological features, post-surgical complications and pathological results. At each patient hospitalization, standard preoperative MRI scans were performed. In 2 cases we also performed a 11C-methionine-positron emission tomography (11C-MET-PET) and images were merged with preoperative MRI and intraoperative CT in order to guide the selection of the optimal biopsy target, as pre-operative standard MRI documented large necrotic and non-enhancing areas.
i-CT scan with contrast enhancement was routinely obtained after patient positioning as previously described [6]. All pre- and intra-operative images were then uploaded, and merged, into the navigation system (StealthStation S8, Medtronic, Minneapolis, Minnesota, USA) and used to choose the best target and plan the safest trajectory.
Pre-operative enhancing tumor volume (MRI) and intra-operative enhancing tumor volume (i-CT) were computed using the StealthViz software package (Medtronic) with semiautomatic segmentation.
All patients received 5-aminolevulinic acid (5-ALA-Gliolan® Medac, Wedel, Germany) at 20 mg/kg body weight, 4 h before surgery. We used a neurosurgical microscope (Kinevo 900, Carl Zeiss) equipped with a filter to switch from conventional white light to ultraviolet light (Blue 400 modality) to visualize 5-ALA induced fluorescence. Each biopsy sample was evaluated to determine its fluorescence status (strong, vague, absent) by two surgeons experienced with fluorescence technology in neuro-oncology [4,9].
All frameless neuronavigated biopsies were performed using the Navigus frame and Stealth Station S8 Neuronavigation System (Medtronic, Minneapolis, Minnesota, USA). We have taken multiple samples from the superficial part of the target, from the central core and from the deep part of the target.
An i-CT scan was routinely obtained after sampling to check that the planned target had been reached and to exclude procedural complications.
We compared the pre- and intra-operative enhancing tumor volume to assess if pre-operative corticosteroid therapy could have increased the sampling error. Moreover, we estimated the correlation between intraoperative fluorescence and pathological findings using a chi square analysis with 0.1 of significance level.
Surgical specimens were formalin-fixed, paraffin-embedded, cut to 4–5 micron and stained with hematoxylin and eosin. Further sections from each paraffin blocks were cut for immunohistochemical analyses that were performed using the streptavidin–biotin labeling technique; then, sections were deparaffinized in xylene for 15 min, rehydrated and treated with 3% H2O2 to deactivate the endogenous peroxidases. The following antibodies were tested: CD20 (rabbit monoclonal; EP459Y; working dilution 1:250), PAX5 (rabbit monoclonal; EPR3730(2); working dilution 1:500), BCL2 (rabbit monoclonal; E17; working dilution 1:500), CD30 (mouse monoclonal; Ber-H2; working dilution 1:100), ALK (rabbit polyclonal; working dilution 1:100), CD10 (mouse monoclonal; 56C6; working dilution 1:50), CD3 (rat monoclonal; 17A2; working dilution 1:100), CD5 (mouse monoclonal; 4C7; working dilution 1:50), Cyclin D1 (rabbit monoclonal; SP4; working dilution 1:100), CD43 (mouse monoclonal; eBio84-3C1 (84-3C1); working dilution 1:200), BCL-6 (mouse monoclonal; BL6.02 (PG-B6p); working dilution 1:50) and Ki67 (rabbit monoclonal; SP6; working dilution 1:200).
We analyzed 45 samples biopsies from 6 immunocompetent patients affected by PCNSLs. We considered clinical, radiological, pathological, and surgical data; patients’ age ranged from 59 to 82 years (median age: 67). At the time of diagnosis, all patients had neurologic deterioration, presenting either constitutional impairment or cognitive or motor symptoms; one presented with coma, four out of six patients had lateralizing symptoms, such as hemiparesis or isolated facial nerve palsy; three presented cognitive impairment, one had amnesia and dysphasia, one aphasia and one ataxia (Table 1). All patients underwent corticosteroid administration from the day of admission following a standard protocol (dexamethasone 16 mg/day). Median time of pre-operative corticosteroid therapy was 10 days; range 7 to 14 days.
Pre-operatively, all patients underwent an MRI scan, including gadolinium-enhancing sequences, demonstrating homogeneous enhancing lesions in all but one case, without central necrosis and with a clear mass effect. In one case there was a central necrosis with diffuse hemorrhagic areas. Three of the six patients had a single, solitary lesion. Diffusion-weighted imaging (DWI) demonstrated restricted diffusion in all lesions, with corresponding high signal intensity on DWI and hypo-intensity on apparent diffusion coefficient (ADC). In one patient the PCNSL was located in the basal ganglia, in another patient the tumor was deep in the left frontal lobe; in two cases there was a multinodular involvement of the corpus callosum; in one patient there was a multinodular involvement of the left Rolandic area and in the last one there was an involvement of the left thalamous (Table 1) (Fig. 1).
Figure 1: (A–F) Different neuroradiological features and locations (arrows) of PCNSL
Because of highly eloquent areas (basal ganglia and the left Rolandic region) and the presence of necrotic/hemorrhagic lesions in one of these patients, we performed a PET-MET scan in two patients. It was then merged with pre-operative MRI and i-CT to detect the safe and effective target as well as the best trajectory (Fig. 2); while the MRI was used to better visualize the brain and vessels, the iCT overimpressed showed the tumors with their real time volume and shape. In this way it was possible to plan the best trajectory considering the correct target and the safest way to avoid sulci, vessels and brain eloquent areas.
Figure 2: (A, B) Trajectory plan based on 11C-MET-PET merged with MRI. (C, D) Isolate neoplastic large B cells infiltrating brain tissue, which show a prominent astrocytic and microglial activation and reactive inflammatory infiltrates consisting of mature T and B cells; nuclear staining with B-cell immunohistochemical marker PAX-5 is seen in large atypical B cells (D) (scale bar 100 μm)
Median preoperative enhancing volume (MRI) was 11.3 cc (ranging from 2.8 to 42.1 cc), while median intraoperative enhancing tumor volume (i-CT) was 8.5 cc (ranging from 0.6 to 28.8 cc). In one patient there was an increase in tumor volume despite corticosteroid use, while 83.3% of cases showed a median 64.4% tumor volume reduction (range 42.1%–87.4%) (Fig. 3).
Figure 3: (A–C) show a progressive reduction of tumor volume (yellow arrows), after corticosteroid administration, among first MRI, second preoperative MRI and i-CT. (D) The post-operative i-CT scan shows an air bubble in the target (green arrow)
In all but one of the six patients, the histological examination performed on surgical samples showed malignant proliferative disease consistent with “diffuse large B-cell lymphoma”; in only one case was the diagnosis “suggestive, but not conclusive, for diffuse large B-cell lymphoma” (Table 1). Overall, 45 samples biopsy were evaluated. 11 (24.4%) were resulted healthy tissue at pathological examination, 13 (28.9%) showed normal brain with tumoral infiltration while 21 (46.6%) samples showed a clear diagnosis of PCNSL. Immunohistochemically, all pathological samples were positive for CD20, PAX5, BCL2, while no staining was obtained with CD30, ALK, CD10, CD3, CD5, Cyclin D1, CD43. Only one case was also positive for BCL-6. Ki67/proliferative index was high, ranging from 40 up to 90%.
Correlation between fluorescence data and pathological findings showed that the surgical samples of 5 patients (83.3%) had a strong homogenous fluorescence induced by 5-ALA (Fig. 4).
Figure 4: Correlation between: (A) post-operative sagittal MRI showing a biopsy track on the posterior tumor margin (arrow); (B) biopsy specimen: half of it shows lava-like 5-ALA fluorescence and the remaining part is not fluorescent. (C, D) histological examination showing half of the sample infiltrated by neoplastic cells (arrow), interface and the other half consisting of unaffected brain. Higher magnification (C) showing large atypical cells with large round, oval, irregular or pleomorphic nuclei and distinct nucleoli, corresponding to centroblasts or immunoblasts. Some cases exhibit a relatively monomorphic cell population, with intermingled macrophages mimicking the morphology of Burkitt lymphoma (scale bars 100 μm-C- and 500 μm-D-)
In detail, on 45 samples, 31 (68.9%) had a lava like fluorescence. Between these fluorescent specimens 21 (67.7%) correlated with pathological finding of PCNSL. On the remaining 14 (31.1%) samples biopsies resulting negative for intraoperative fluorescence, 13 (92.9%) were also positive for PCNSL at pathological examination. The only patient with a suggestive, but not conclusive, diagnosis of diffuse large B-cell lymphoma had a similar strong intraoperative fluorescence, while the only patient with non-fluorescent surgical samples had a histologically proven diffuse large B-cell lymphoma. A chi-square analysis shows a correlation between intraoperative fluorescence and pathological findings (p-v of 0.07).
i-CT scan after biopsies confirmed sampling accuracy and excluded procedural complications in all cases (Fig. 5).
Figure 5: (A–D) Preoperative planning of biopsies (upper left image) and preoperative MRI merged with i-CT after biopsies confirming the correct target and the absence of early post-operative complications
Our patient data ruled out ocular involvement. As information on cerebrospinal fluid analysis was not available, potential concurrent leptomeningeal involvement could not be completely excluded. No post-surgical complications were documented.
PCNSL is a rare primary brain tumor, representing a subtype of extra-nodal non-Hodgkin lymphomas that can involve the brain, eyes, meninges or the spinal cord with the absence of systemic disease. The annual incidence of PCNSLs is around 7 cases per 1 000 000 people in the USA, with a male predominance occurring mostly in the elderly population [1]. Epidemiological studies have demonstrated the increase of incidence of PCNSLs in the last few decades, with higher overall rates in immunocompetent patients aged 65 years or older [10,11]. When PCNSL is suspected, contrast MRI is the primary diagnostic modality. Typical locations on MRI include periventricular white matter, basal ganglia, and corpus callosum [12]. Usually, PCNSLs enhance homogeneously but sometimes the enhancement is mild or with a ring pattern or is even absent [13]. Most cases of PCNSLs present as single lesions (about 70% of cases), while in the remaining 30% of cases multiple lesions can be observed. Differential diagnosis includes high grade gliomas (HGGs) and, less often, tumefactive demyelinating lesions (locally aggressive form of demyelination that may mimic a tumor-Balo like, Marburg variant, etc.), metastasis and brain abscess. CNS lymphomas may have a characteristic appearance on traditional CT and MR imaging; however, radiological features are not specific and thus they cannot unequivocally differentiate PCNSLs from other neoplasms or non-neoplastic diseases. DWI, perfusion MR imaging and MR spectroscopy are increasingly used in clinical practice and may help to differentiate PCNSLs from other brain lesions. DWI measures the diffusion of water molecules in biologic tissues; because PCNSLs are highly cellular tumors, water diffusion is often restricted, resulting in making them appear hyperintense on DWI and hypointense on ADC maps. PCNSLs often have a more restricted diffusion and lower ADC values than high-grade gliomas and metastases [14,15]. Previous studies have shown that an ADC value lower than 600 10–6 mm2/s is considered suggestive of CNS lymphomas [16]. 1H-MR spectroscopy (1H-MRS) obtains biochemical information noninvasively from biologic tissue. In PCNSLs, 1H-MRS has demonstrated reduced N-acetylaspartate (NAA) peaks (neuronal damage) and elevated lipid peaks combined with high Cho/Cr ratios.
However, these features can also be seen in glioblastoma multiforme (GBM), but in this tumor lipid peaks are due to necrosis; therefore, an increase in lipid resonance in the absence of necrosis seems to be the most specific finding in PCNSLs [15,17]. Perfusion MR imaging visualizes nutritive delivery of arterial blood to the capillary bed in biologic tissues (e.g., tumors); post-processing of the acquired data enables calculation of physiologic parameters, such as CBV, cerebral blood flow, mean transit time, and time to peak. PCNSLs demonstrate low CBV and a characteristic intensity time curve, which is related to a massive leakage of contrast media into the interstitial space. Furthermore, maximum relative CBV measured in the tumor tissue, calculated as the ratio to contralateral normal-appearing white matter, is typically lower in lymphomas than in other brain tumors.
This characteristic finding can help to differentiate GBMs and metastases from PCNSLs [18]. PCNSLs have higher cellularity and nuclear-cytoplasm ratio than GBMs. Thus, in PCNSLs, DWI results more restricted than in GBMs, with a lower apparent diffusion coefficient (ADC) [14]. 1H-magnetic resonance spectroscopy (1H-MRS) can help physicians in distinguishing PCNSLs from GBMs. In both cases we can usually find a reduced N-acetylaspartate (NAA) peak (neuronal damage), an elevated choline to creatine ratio for the elevated membrane turnover, a lipid peak due to the release of fats by transformed lymphocytes in PCNSL and to necrosis in GBM, and lactate peaks caused by the anaerobic metabolism. An increase in lipid resonance in the absence of necrosis seems to be the most specific finding of PCNSLs [17]. As concerns amino acid PET, 11C-methionine (MET-PET) uptake reflects an increase in cellular proliferation. In the evaluation of CNS tumors, 11C-MET-PET presents some advantages over Fluorodeoxyglucose (FDG), due to its lower uptake in the normal brain. 11C-MET-PET is useful for delineating PCNSL tumor boundaries, with larger areas of increased uptake than the enhancing tumor visualized on MRI [19,20].
However, neuroradiological findings are suggestive but not diagnostic of PCNSLs, and definitive diagnosis needs histological confirmation by biopsy. A positive cerebrospinal fluid (CSF) or vitreous biopsy (in the presence of ocular involvement) can obviate the need for a neurosurgical procedure but the rate of cytological/histological diagnosis on CSF/vitreous sampling remains lower than on brain biopsy [21]. Indeed, the accuracy rate of CSF flow cytometry (FCM) and cytomorphology (CM) ranges from 0.3% to 42.9% in different studies, while the diagnostic rate of an ocular examination is about 50% [3].
Currently, most papers evaluate the success rate of stereotactic biopsies by “the percentage of cases in which a conclusive histopathological diagnosis could be reached” [22,23]. The so-called “diagnostic yield” reported in the literature is 76%−99% [24,25].
Considering the superior diagnostic yield and low morbidity, an early brain biopsy has been recommended to prevent diagnostic delay [11]. However, it is well known that corticosteroids can cause rapid tumor regression and decrease in tumor-associated edema in about 45%–50% of PCNSLs. It is also well demonstrated that an initial response to corticosteroid portends a more favorable outcome in PCNSLs (117 months in responders vs. 5.5 months in non-responders) [26]. This PCNSL feature often allows a “ex juvantibus” diagnosis but could be a crucial pitfall to obtain a diagnostic brain biopsy. In order to increase the diagnostic accuracy, some authors have suggested the use of 5-ALA, describing that about 75% of PCNSLs showed strong intraoperative 5-ALA fluorescence [27,28]. Other authors reported that the combination of intraoperative MRI and 5-ALA increased the safety and accuracy of brain biopsies [29].
5-ALA actively accumulates in malignant glial cells, where it is converted to a fluorescent substance, protoporphyrin IX [27,28,30,31]. This phenomenon has a high tumor specificity; hence, 5-ALA has been adopted as a specific intraoperative marker for HGGs and low-grade gliomas (LGGs) with anaplastic foci [27,28,30,31]. In addition to glioma surgery, the application of 5-ALA has been described in brain metastases, lymphomas and meningiomas, as well as in other non glial tumors. These tumors can display different degrees of fluorescence, and 5-ALA has been used as a guide for tissue sampling or to extend resection to infiltrated white matter [27,28,30,31].
However, the exact mechanism of 5-ALA metabolism and accumulation of fluorescent protoporphyrin IX in these different subsets has been not yet clarified.
Marbacher et al. first described the use of 5-ALA fluorescence in stereotactic biopsies of suspected intracranial lymphomas involved 6 patients, with visible 5-ALA–induced fluorescence in half of the biopsied tumors [30]. Kiesel et al. in a recent study, demonstrated that approximately 20% of intracranial lymphomas lack visible fluorescence during stereotactic biopsies [27]. The precise mechanism of 5-ALA uptake and metabolism determining intraoperative fluorescence or intracranial lymphomas, is not yet fully understood. In vitro studies of glioblastoma cells demonstrated that corticosteroids result in a significantly decreased 5-ALA–induced PpIX production by tumor cells but there are no specific study for PCNSL. Desmoulin et al. described a proton-coupled folate transporter that might have a crucial role in the metabolism of 5-ALA in PCNSL [32].
To the best of our knowledge this is the first description of the use of a multimodal intraoperative imaging approach involving i-CT, 11C-MET-PET and 5-ALA fluorescence. Our protocol has been extensively described and validated for tumor resection; it is now applied, for the first time, to frameless neuronavigated PCNSL biopsies [5,6,33]. In this early experience, i-CT helped to demonstrate unpredictable variations in tumor volume and morphology after corticosteroid administration. In detail, we encountered only one patient with increased tumor volume; conversely, in 83.3% of our cases a median value of 64.4% in tumor volume reduction (42.1%–87.4%) was found after i-CT. It is deducible that, in light of the documented 64.4% of volumetric changes, we could have an increase of possibility of a sampling error if the target is not centered on the updated neuroimaging. We think that contrast-enhanced i-CT, also merged with 11C-MET-PET, becomes crucial to update the surgeon’s knowledge of tumor morphology and to plan the safest trajectory on the best target. However, as part of our multimodal imaging protocol, MET-PET imaging also proved to be very useful in analyzing the regions with high metabolic activity and helped to choose targets with the highest chance of a positive sample. In this series we found 68.9% of surgical samples with strong intraoperative 5-ALA fluorescence. The only patient without positive fluorescence had a tumor sample, which was deemed diagnostic by histological examination. In one case (basal ganglia tumor with a large necrotic/hemorrhagic component) we observed strong intraoperative 5-ALA fluorescence, with a suggestive, but not conclusive, histological diagnosis of PCNSL. Although the corticosteroids generally should cause a rapid volume reduction of PCNSL, one patient from our series had an increased tumor volume despite the corticosteroid therapy; we first analyzed the volumetric evolution of these tumors and their therapy response. In this way further studies are needed to improve our knowledge about this topic.
The literature shows a percentage of PCNSL positive to 5-ALA that varies between 62% and 100% [34]. Kiesel et al. reported a large series with 100% of cases in which there was strong fluorescence, a predictive positive value of 100%, sensitivity of 63% and a specificity of 100% [27]. Although it is well known that these tumors can display different degrees of fluorescence, the exact mechanism of 5-ALA metabolism and accumulation of fluorescent protoporphyrin IX in the different subsets has not been clarified yet [4,28,35–37]. Some clinicians studied the correlation between ADC, relative CBV and Ki-67 proliferation rate in CNS lymphomas and found that ADC may be used as noninvasive predictor of cell proliferation in these lesions [38].
Lin et al. described a retrospective series of 12 patients affected by PCNSL and treated with the support of sodium fluorescein. They depict that the specificity of the specimens sent for frozen section was 86.4% (19/22) [39]. In our series we have a 100% of confirmation of biopsy correctness with i-CT, so despite the cost increase, accordingly with the evidence of a sensitivity and specificity lower than 90% of every intraoperative fluorescence technique, we support the importance of integrate this important tool with the i-CT in order to improve the diagnostic yield.
Although we are aware that performing a new MRI after corticosteroid therapy can also overcome the potential morphological changes induced by the therapy in order to correctly plan the biopsy, we emphasize that our multi-modal approach seems reasonably faster and no less effective in avoiding sampling errors, as MRI is more time consuming compared to the intraoperative CT scan and 5-ALA could further improve the accuracy of the procedure.
We acknowledge several limitations: the number of studied patients should be increased; the evaluation of tumor volumes based on two different imaging modalities (MRI and i-CT) limits the accuracy of tumor reduction quantification. In addition, we emphasize that we translated the protocol used for tumor resection on the biopsies; accordingly, the planning was performed intraoperatively and we have not compared our results with those obtained using different protocol. Consequently, we cannot be sure neither that the planned biopsy trajectory has changed significantly after the use of our protocol, nor that the percentage of non-representative biopsies would have been higher without i-CT and 5-ALA.
However, this is a preliminary experience on a rare brain tumor histotype showing for the first time the possibility to use the proposed intraoperative multimodal approach also with frameless neuronavigated biopsies for PCNSLs. Despite the difference in imaging modalities used to compute preoperative and intraoperative tumor volume, we believe that this is the first study computing such a volume, demonstrating the role of i-CT and its importance in updating tumor morphological data to reduce sampling errors.
A further interesting perspective in the diagnosis of CNS lymphomas is the possibility of obtaining a diagnosis without biopsy, by searching for miRNAs on CSF by FCM. This promising technique seems to be very useful when stereotactic brain biopsy cannot be performed due to the location of the lesion, or in case of vanishing tumors after the corticosteroid therapy [37,38]. In this regards, different miRNA expression profiles between PCNSLs and other brain tumors have been demonstrated by some clinicians: in more detail, miR-21, miR-19b, and miR-92a levels were found to be significantly higher in CSF of patients with PCNSL than of patients with other brain neoplasms [40,41].
PCNSLs are relatively rare brain tumors and early, accurate diagnosis is difficult to achieve. Their subacute progressive clinical presentation often induces clinicians to treat patients with corticosteroids; however, such therapy can determine significant tumor volume reduction and changes in morphology, which can only be seen by repeated MRI. 5-ALA fluorescence proved to be a valid tool in PCNSL diagnosis and i-CT is increasingly recognized as reliable, cheaper and easier-to-use than i-MRI imaging modality, helping to obtain updated neuronavigation data and real-time tumor information [42]. In conclusion, we emphasize that the use of a multimodal intraoperative imaging approach can overcome the demonstrated PCNSL morphological changes caused by corticosteroid therapy and obtain a reliable tissue diagnosis by frameless biopsy. We also highlight the necessity to update in “real time” the neuroimaging in order to obtain a better planning of the target in light of the demostrated volumetrical modification.
Authorship: The authors confirm contribution to the paper as follows: study conception and design: Roberto Altieri, Francesco Certo and Giuseppe Barbagallo; data collection: Roberto Altieri, Giacomo Cammarata, Massimiliano Maione, Marco Garozzo, Giuseppe Broggi, Rosario Caltabiano and Giada Maria Vecchio; analysis and interpretation of results: Giuseppa Fiumanò; draft manuscript preparation: Roberto Altieri, Gaetano Magro and Giuseppe Barbagallo. All authors reviewed the results and approved the final version of the manuscript.
Ethics Approval and Informed Consent Statement: The study was performed in accordance with the 1964 Declaration of Helsinki and approved by the Catania 1 Ethics Committee, Santa Sofia 78 street, Catania, Italy (protocol code: 166/2015/PO; 17/12/2015); the non-interventional retrospective nature of our study did not require any informed consent by the local research ethics committee.
Availability of Data and Materials: All data presented in this study are available from the corresponding author upon reasonable request.
Funding Statement: The authors received no specific funding for this study.
Conflicts of Interest: The authors declare that they have no conflicts of interest to report regarding the present study.
References
1. Villano, J. L., Koshy, M., Shaikh, H., Dolecek, T. A., McCarthy, B. J. (2011). Age, gender, and racial differences in incidence and survival in primary CNS lymphoma. British Journal of Cancer, 105(9), 1414–1418. DOI 10.1038/bjc.2011.357. [Google Scholar] [CrossRef]
2. Carnevale, J., Rubenstein, J. L. (2016). The challenge of primary central nervous system lymphoma. Hematology/Oncology Clinics of North America, 30(6), 1293–1316. DOI 10.1016/j.hoc.2016.07.013. [Google Scholar] [CrossRef]
3. Chiavazza, C., Pellerino, A., Ferrio, F., Cistaro, A., Soffietti, R. et al. (2018). Primary CNS lymphomas: Challenges in diagnosis and monitoring. BioMed Research International, 2018, 3606970. DOI 10.1155/2018/3606970. [Google Scholar] [CrossRef]
4. Certo, F., Stummer, W., Farah, J. O., Freyschlag, C., Visocchi, M. et al. (2019). Supramarginal resection of glioblastoma: 5-ALA fluorescence, combined intraoperative strategies and correlation with survival. Journal of Neurosurgical Sciences, 63(6), 625–632. DOI 10.23736/S0390-5616.19.04787-8. [Google Scholar] [CrossRef]
5. Barbagallo, G., Morrone, A., Certo, F. (2018). Intraoperative computed tomography and awake craniotomy: A useful and safe combination in brain surgery. World Neurosurgery, 119, e159–e166. DOI 10.1016/j.wneu.2018.07.078. [Google Scholar] [CrossRef]
6. Barbagallo, G., Palmucci, S., Visocchi, M., Paratore, S., Attinà, G. et al. (2016). Portable intraoperative computed tomography scan in image-guided surgery for brain high-grade gliomas: Analysis of technical feasibility and impact on extent of tumor resection. Operative Neurosurgery, 12(1), 19–30. DOI 10.1227/NEU.0000000000001112. [Google Scholar] [CrossRef]
7. Altieri, R., Zenga, F., Fontanella, M. M., Cofano, F., Agnoletti, A. et al. (2015). Glioma surgery: Technological advances to achieve a maximal safe resection. Surgical Technology International, 27, 297–302. [Google Scholar]
8. Altieri, R., Melcarne, A., di Perna, G., Specchia, F., Fronda, C. et al. (2018). Intra-operative ultrasound: Tips and tricks for making the most in neurosurgery. Surgical Technology International, 33, 353–360. [Google Scholar]
9. Altieri, R., Meneghini, S., Agnoletti, A., Tardivo, V., Vincitorio, F. et al. (2019). Intraoperative ultrasound and 5-ALA: The two faces of the same medal? Journal of Neurosurgical Sciences, 63(3), 258–264. DOI 10.23736/S0390-5616.16.03641-9. [Google Scholar] [CrossRef]
10. Shiels, M. S., Pfeiffer, R. M., Besson, C., Clarke, C. A., Morton, L. M. et al. (2016). Trends in primary central nervous system lymphoma incidence and survival in the U.S. British Journal of Haematology, 174(3), 417–424. DOI 10.1111/bjh.14073. [Google Scholar] [CrossRef]
11. Morell, A. A., Shah, A. H., Cavallo, C., Eichberg, D. G., Sarkiss, C. A. et al. (2019). Diagnosis of primary central nervous system lymphoma: A systematic review of the utility of CSF screening and the role of early brain biopsy. Neuro-Oncology Practice, 6(6), 415–423. DOI 10.1093/nop/npz015. [Google Scholar] [CrossRef]
12. Jiménez de la Peña, M. D., Vicente, L. G., Alonso, R. C., Cabero, S. F., Suárez, A. M. et al. (2017). The multiple faces of nervous system lymphoma. Atypical magnetic resonance imaging features and contribution of the advanced imaging. Current Problems in Diagnostic Radiology, 46(2), 136–145. DOI 10.1067/j.cpradiol.2016.04.004. [Google Scholar] [CrossRef]
13. Sutherland, T., Yap, K., Liew, E., Tartaglia, C., Pang, M. et al. (2012). Primary central nervous system lymphoma in immunocompetent patients: A retrospective review of MRI features. Journal of Medical Imaging and Radiation Oncology, 56(3), 295–301. DOI 10.1111/j.1754-9485.2012.02366.x. [Google Scholar] [CrossRef]
14. Malikova, H., Koubska, E., Weichet, J., Klener, J., Rulseh, A. et al. (2016). Can morphological MRI differentiate between primary central nervous system lymphoma and glioblastoma? Cancer Imaging, 16(1), 40. DOI 10.1186/s40644-016-0098-9. [Google Scholar] [CrossRef]
15. Haldorsen, I. S., Espeland, A., Larsson, E. M. (2011). Central nervous system lymphoma: Characteristic findings on traditional and advanced imaging. American Journal of Neuroradiology, 32(6), 984–992. DOI 10.3174/ajnr.A2171. [Google Scholar] [CrossRef]
16. Huang, W. Y., Wen, J. B., Wu, G., Yin, B., Li, J. J. et al. (2016). Diffusion-weighted imaging for predicting and monitoring primary central nervous system lymphoma treatment response. American Journal of Neuroradiology, 37(11), 2010–2018. DOI 10.3174/ajnr.A4867. [Google Scholar] [CrossRef]
17. Lu, S. S., Kim, S. J., Kim, N., Kim, H. S., Choi, C. G. et al. (2015). Histogram analysis of apparent diffusion coefficient maps for differentiating primary CNS lymphomas from tumefactive demyelinating lesions. American Journal of Roentgenology, 204(4), 827–834. DOI 10.2214/AJR.14.12677. [Google Scholar] [CrossRef]
18. Hartmann, M., Heiland, S., Harting, I., Tronnier, V. M., Sommer, C. et al. (2003). Distinguishing of primary cerebral lymphoma from high-grade glioma with perfusion-weighted magnetic resonance imaging. Neuroscience Letters, 338(2), 119–122. DOI 10.1016/s0304-3940(02)01367-8. [Google Scholar] [CrossRef]
19. Gupta, M., Gupta, T., Purandare, N., Rangarajan, V., Puranik, A. et al. (2019). Utility of flouro-deoxy-glucose positron emission tomography/computed tomography in the diagnostic and staging evaluation of patients with primary CNS lymphoma. CNS Oncology, 8(4). DOI 10.2217/cns-2019-0016. [Google Scholar] [CrossRef]
20. Mohile, N. A., Deangelis, L. M., Abrey, L. E. (2008). The utility of body FDG PET in staging primary central nervous system lymphoma. Neuro-Oncology, 10(2), 223–228. DOI 10.1215/15228517-2007-061. [Google Scholar] [CrossRef]
21. Hoang-Xuan, K., Bessell, E., Bromberg, J., Hottinger, A. F., Preusser, M. et al. (2015). Diagnosis and treatment of primary CNS lymphoma in immunocompetent patients: Guidelines from the European Association for Neuro-Oncology. The Lancet Oncology, 16(7), e322–e332. DOI 10.1016/S1470-2045(15)00076-5. [Google Scholar] [CrossRef]
22. Calişaneller, T., Ozdemir, O., Ozger, O., Ozen, O., Kiyici, H. et al. (2008). The accuracy and diagnostic yield of computerized tomography guided stereotactic biopsy in brain lesions. Turkish Neurosurgery, 18(1), 17–22. [Google Scholar]
23. Aker, F. V., Hakan, T., Karadereler, S., Erkan, M. (2005). Accuracy and diagnostic yield of stereotactic biopsy in the diagnosis of brain masses: Comparison of results of biopsy and resected surgical specimens. Neuropathology, 25(3), 207–213. DOI 10.1111/j.1440-1789.2005.00634.x. [Google Scholar] [CrossRef]
24. Tilgner, J., Herr, M., Ostertag, C., Volk, B. (2005). Validation of intraoperative diagnoses using smear preparations from stereotactic brain biopsies: Intraoperative versus final diagnosis--influence of clinical factors. Neurosurgery, 56(2), 257–265. DOI 10.1227/01.neu.0000148899.39020.87. [Google Scholar] [CrossRef]
25. Shooman, D., Belli, A., Grundy, P. L. (2010). Image-guided frameless stereotactic biopsy without intraoperative neuropathological examination. Journal of Neurosurgery, 113(2), 170–178. DOI 10.3171/2009.12.JNS09573. [Google Scholar] [CrossRef]
26. Mathew, B. S., Carson, K. A., Grossman, S. A. (2006). Initial response to glucocorticoids. Cancer, 106(2), 383–387. DOI 10.1002/cncr.21583. [Google Scholar] [CrossRef]
27. Kiesel, B., Millesi, M., Woehrer, A., Furtner, J., Bavand, A. et al. (2018). 5-ALA-induced fluorescence as a marker for diagnostic tissue in stereotactic biopsies of intracranial lymphomas: Experience in 41 patients. Neurosurgical Focus, 44(6). DOI 10.3171/2018.3.FOCUS1859. [Google Scholar] [CrossRef]
28. La Rocca, G., Sabatino, G., Menna, G., Altieri, R., Ius, T. et al. (2020). 5-Aminolevulinic acid false positives in cerebral neuro-oncology: Not all that is fluorescent is tumor. A case-based update and literature review. World Neurosurgery, 137, 187–193. DOI 10.1016/j.wneu.2020.01.238. [Google Scholar] [CrossRef]
29. Giordano, M., Gallieni, M., Zaed, I., Samii, A. (2019). Use of frameless stereotactic navigation system combined with intraoperative magnetic resonance imaging and 5-aminolevulinic acid. World Neurosurgery, 131, 32–37. DOI 10.1016/j.wneu.2019.07.171. [Google Scholar] [CrossRef]
30. Marbacher, S., Klinger, E., Schwyzer, L., Fischer, I., Nevzati, E. et al. (2014). Use of fluorescence to guide resection or biopsy of primary brain tumors and brain metastases. Neurosurgical Focus, 36(2), E10. DOI 10.3171/2013.12.FOCUS13464. [Google Scholar] [CrossRef]
31. Lawrence, J. E., Steele, C. J., Rovin, R. A., Belton Jr., R. J., Winn, R. J. (2016). Dexamethasone alone and in combination with desipramine, phenytoin, valproic acid or levetiracetam interferes with 5-ALA-mediated PpIX production and cellular retention in glioblastoma cells. Journal of Neuro-Oncology, 127(1), 15–21. DOI 10.1007/s11060-015-2012-x. [Google Scholar] [CrossRef]
32. Desmoulin, S. K., Hou, Z., Gangjee, A., Matherly, L. H. (2012). The human proton-coupled folate transporter: Biology and therapeutic applications to cancer. Cancer Biology & Therapy, 13(14), 1355–1373. DOI 10.4161/cbt.22020. [Google Scholar] [CrossRef]
33. Barbagallo, G., Maione, M., Peschillo, S., Signorelli, F., Visocchi, M. et al. (2019). Intraoperative computed tomography, navigated ultrasound, 5-amino-levulinic acid fluorescence and neuromonitoring in brain tumor surgery: Overtreatment or useful tool combination? Journal of Neurosurgical Sciences. DOI 10.23736/S0390-5616.19.04735-0. [Google Scholar] [CrossRef]
34. Boschi, A., Della Puppa, A. (2019). 5-ALA fluorescence on tumors different from malignant gliomas. review of the literature and our experience. Journal of Neurosurgical Sciences, 63(6), 661–669. DOI 10.23736/S0390-5616.19.04766-0. [Google Scholar] [CrossRef]
35. La Rocca, G., Della Pepa, G. M., Menna, G., Altieri, R., Ius, T. et al. (2019). State of the art of fluorescence guided techniques in neurosurgery. Journal of Neurosurgical Sciences, 63(6), 619–624. DOI 10.23736/S0390-5616.19.04854-9. [Google Scholar] [CrossRef]
36. Lau, D., Hervey-Jumper, S. L., Chang, S., Molinaro, A. M., McDermott, M. W. et al. (2016). A prospective phase II clinical trial of 5-aminolevulinic acid to assess the correlation of intraoperative fluorescence intensity and degree of histologic cellularity during resection of high-grade gliomas. Journal of Neurosurgery, 124(5), 1300–1309. DOI 10.3171/2015.5.JNS1577. [Google Scholar] [CrossRef]
37. Certo, F., Altieri, R., Maione, M., Schonauer, C., Sortino, G. et al. (2021). FLAIRectomy in supramarginal resection of glioblastoma correlates with clinical outcome and survival analysis: A prospective, single institution, case series. Operative Neurosurgery, 20(2), 151–163. DOI 10.1093/ons/opaa293. [Google Scholar] [CrossRef]
38. Duy Hung, N., Minh Duc, N., Hong Nhung, T., Thanh Dung, L., van Giang, B. et al. (2020). The correlation between apparent diffusion coefficient (ADC) and relative cerebral blood volume (rCBV) with Ki-67 expression in central nervous system lymphoma. International Journal of Cancer Management, 13(12), e107834. DOI 10.5812/ijcm.107834. [Google Scholar] [CrossRef]
39. Lin, F. H., Zhang, X. H., Zhang, J., He, Z. Q., Duan, H. et al. (2018). Fluorescein sodium-guided biopsy or resection in primary central nervous system lymphomas with contrast-enhancing lesion in MRI. Journal of Neuro-Oncology, 139(3), 757–765. DOI 10.1007/s11060-018-2924-3. [Google Scholar] [CrossRef]
40. Sromek, M., Rymkiewicz, G., Paziewska, A., Szafron, L. M., Kulecka, M. et al. (2021). A set of 17 microRNAs common for brain and cerebrospinal fluid differentiates primary central nervous system lymphoma from non-malignant brain tumors. Biomolecules, 11(9), 1395. DOI 10.3390/biom11091395. [Google Scholar] [CrossRef]
41. Zajdel, M., Rymkiewicz, G., Sromek, M., Cieslikowska, M., Swoboda, P. et al. (2019). Tumor and cerebrospinal fluid microRNAs in primary central nervous system lymphomas. Cancers, 11(11), 1647. DOI 10.3390/cancers11111647. [Google Scholar] [CrossRef]
42. Altieri, R., Barbagallo, D., Certo, F., Broggi, G., Ragusa, M. et al. (2021). Peritumoral microenvironment in high-grade gliomas: From FLAIRectomy to microglia-glioma cross-talk. Brain Sciences, 11(2), 200. DOI 10.3390/brainsci11020200. [Google Scholar] [CrossRef]
Cite This Article
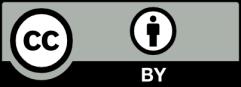
This work is licensed under a Creative Commons Attribution 4.0 International License , which permits unrestricted use, distribution, and reproduction in any medium, provided the original work is properly cited.