Open Access
REVIEW
The Emerging Role of Lymphocyte Activation Gene 3 in the Cancer Immunotherapy
1
Department of Pathology, School of Basic Medical Sciences, Wuhan University, Wuhan, 430071, China
2
Department of Oncology, Renmin Hospital of Wuhan University, Wuhan, 430060, China
* Corresponding Author: Honglei Chen. Email:
Oncologie 2022, 24(4), 665-678. https://doi.org/10.32604/oncologie.2022.023641
Received 07 May 2022; Accepted 07 November 2022; Issue published 31 December 2022
Abstract
The emergence of tumor immunotherapy, especially immune checkpoint inhibitors (ICIs), has brought new life to cancer treatment, and ICIs can effectively treat various tumors. Among the immune anti-cancer therapies, PD-1 is undoubtedly the hot target after CTLA-4, but due to the ineffectiveness of PD-1 in treating certain tumors, researchers have shifted their focus to other combination targets, such as LAG-3, TIM3, IDO-1, etc. One of these promising targets is LAG-3, a target with multiple clinical trials, which has increasingly shown to be an inhibitory co-receptor that plays a vital role in autoimmunity, cancer immunity, and anti-infection immunity. Immune combination therapy with LAG-3 is a hot topic, but its limitations in clinical application are also evident. This review briefly describes the molecular structure, biological functions of LAG-3 in the immune cells and cancer cells and prognosis significance, and then an overview of relevant ongoing clinical trials.Keywords
During the past several decades, the rapid development of cancer immunotherapy, especially the emergence of immune checkpoint inhibitors (ICIs), has equipped a novel and powerful “weapon” for cancer therapy [1]. ICIs therapy is one of the most extensively studied immunotherapies currently. It regulates T cell activities by co-inhibition or costimulatory signal transmission, thus inducing the anti-cancer effect of T lymphocytes [2]. Co-inhibitory receptors, such as cytotoxic T-lymphocyte-associated protein-4 (CTLA-4), programmed cell death 1 (PD-1), and programmed cell death-ligand 1 (PD-L1), exert a key function in modulating T cell responses and have confirmed to be efficacious targets for chronic diseases. The expression of constitutive co-inhibitory receptors on T cells can suppress effector T cell responses. Unfavorably, a larger proportion of cancer patients remain no response to ICI treatment. Using new ICIs combined with current immunotherapies may ameliorate clinical consequences [3,4]. Lymphocyte-activation gene 3 (LAG-3) is an inhibitory molecule with multiple biological effects on T cell functions and is regarded as a promising immune checkpoint receptor. High expression of LAG-3 is detected in the tumor-infiltrating lymphocytes (TILs), natural killer cells, and plasmacytoid dendritic cells, and partakes in different cancers’ immune escape mechanisms [5,6]. In the activated lymphocytes, LAG-3 expression involves the negative modulation of cell activation/proliferation to assure effectively the immune response. Inhibition of LAG-3 can regain the cytotoxicity of T cells, thereby boosting the killing effect on cancer cells. Simultaneously, LAG-3 can also enhance the function of regulatory T cells (Tregs) to suppress the immune response. Therefore, LAG-3 can be regarded as a more appealing target than other immune checkpoint proteins. This review briefly describes the molecular function of LAG-3, focusing on recent advances in LAG-3-associated immunotherapy and the prognosis significance of various cancers.
2 Molecular Structure and Biological Functions of LAG-3
LAG-3, also called CD223, was first identified by Frédéric Triebel’s team in 1990 [7], a transmembrane type I protein containing 498 amino acids and belonging to the immunoglobulin superfamily. LAG-3 includes three parts: an extracellular domain, a transmembrane domain, and a cytoplasmic domain. The extracellular domain of LAG-3 has about 20% amino acid homology with CD4. LAG-3 has four Ig-like domains called domain 1 (D1) to domain 4 (D4), and CD4 also has four Ig-like domains. Furthermore, the LAG-3 gene is localized on chromosome 12 (12P13), which is similar to the CD4 molecule localized on the chromosome. Therefore, CD4 and LAG-3 are firmly linked, but their functions are contrary [8,9].
LAG-3 is similar to the protein sequence of the CD4 receptor. One of its ligands is the major histocompatibility complex MHC (major histocompatibility complex) class II molecules with an affinity even stronger than CD4 [10,11]. LAG-3 could directly and precisely engage with intact human leukocyte antigen class II (HLA-II) heterodimers [12]. LAG-3 can downregulate the proliferation, activation, and dynamic balance of T cells by interrupting the connection of CD4 with MHC II through binding to MHC II molecules. LAG-3 also strengthens Tregs function, conserves the stability of the internal environment, and partakes in the function of immunomodulation, which intimately links to the occurrence and development of cancers [11,13]. In addition, it has been shown that the binding of LAG-3 to MHC II molecules leads to immunosuppression of CX3CR1+ tissue-resident macrophages [14]. Inhibition of LAG-3 using therapeutic antibodies relieves the suppression of T cells and enhances the body’s immune response. The soluble molecule of LAG-3 (sLAG-3) first provokes antigen-presenting cell activity via MHC II signaling and then impels an antigen-specific T-cell response [15]. However, there is controversy as to whether MHC II molecules alone regulate the roles of immune suppression of LAG-3 [16]. For example, LAG-3 can restrain CD8+ T cell proliferation without the involvement of MHC II, and thus LAG-3 exerts an immunosuppressive function that mediated by other unknown ligands [17]. LAG-3 binding to stable peptide-MHC class II (pMHCII) restrains T cell function, and then represses autoimmunity and anti-cancer immunity. Therefore, stable pMHCII-LAG-3 interaction is a promising therapeutic target in human diseases [18].
Fibrinogen-like protein 1 (FGL1) is an essential functional ligand for LAG-3 and revealed that this LAG-3-FGL1 pathway is independent of the B7-H1-PD-1 tumor immune escape pathway, and blocking this pathway can cooperate with anti-PD-1 therapy [19]. In addition, galectin-3 (GAL-3) is a galectin-binding soluble lectin widely expressed in various cell types and can regulate antigen-specific T-cell activation. LAG-3 can be extensively glycosylated and is considered a suitable target for binding to GAL-3 (Fig. 1). Moreover, LAG-3 expression closely correlates with GAL-3, and the interaction between the two further promotes the suppression of cytotoxic T lymphocytes (CTLs) in the tumor microenvironment (TME) [20,21]. Liver sinusoidal endothelial cell lectin (LSECtin) belongs to the c-type lectin receptor superfamily and strongly expresses in hepatocytes and melanoma cells. LSECtin has an anti-cancer immunosuppressive effect similar to LAG-3. LSECtin binding to LAG-3 reduces interferon (IFN)-γ production by activated T cells. LSECtin usually expresses in cancer cells, such as melanoma cells, involved in the cancer-immune escape, and boosts cancer growth [22,23].
Figure 1: The biological functions of LAG-3 and its ligands in the tumor microenvironment
The binding of LAG-3 to MHC II molecules leads to immunosuppression of T cells. LAG-3 also strengthens the suppressive activity of Tregs. The LAG-3-FGL1 pathway is independent of the B7-H1-PD-1 pathway. LAG-3 combined with GAL-3 further promotes CTLs’ suppression in the TME. LSECtin from cancer cells binding to LAG-3 reduces IFN-γ production by activated T cells. FS118 is a surrogate murine bispecific antibody [mLAG-3/PD-L1 mAb (2)], that can simultaneously block the immune suppression mediated by LAG-3 and PD-L1, and ameliorate T-cell activity.
2.3 Biological Function of LAG-3
T cells play a key role in antiviral and antitumor immune responses. Proper activation of antigen-specific T cells causes their clonal expansion and the acquirement of effector functions. For example, CTLs enable lyse target cells specifically. In TME or chronic infectious diseases, T cells become dysfunctional: T cells lose their proliferative capacity in antigens and gradually fail to produce cytokines and lyse target cells [24]. This dysfunctional state is known as “exhaustion” (a molecular signature of exhausted T cells). During chronic viral infections or cancer, sustained activation of T cells induces co-expression of immunosuppressive molecules including LAG-3, PD-1, T cell immunoglobulin-3 and mucin structural domain 3 (TIM-3), T cell immunoreceptor with Ig and ITIM domains (TIGIT) and CD160, contributing to T cell incompetence and even apoptosis [25]. LAG-3 expression on activated T cells is increased by regulating interleukin (IL)-2, IL-7, and IL-12, but not IL-4, IL-6, IL-10, tumor necrosis factor (TNF), and IFN-γ [26]. Interestingly, multiple transcriptional regulators such as nuclear factor of activated T cells (NFAT), thymocyte selection-associated high mobility group box protein (TOX), nuclear receptor subfamily 4 group A (NR4A) interact with other inhibitory coreceptors. They augment the expression levels of LAG-3 when overexpressed in T cells [27–33].
In the TME, infiltrating immune cells including various lymphocytes that assault cancer cells or help them survive in the host immune system. LAG-3 as well as other inhibitory coreceptors such as PD-1 and TIM-3 are highly expressed in the immune or cancer cells of TME, inducing depletion and loss of antitumor T cells that play a vital role in cancer immune escape. Higher LAG-3 and TIM-3 levels are significantly detected, compared to PD-1 and CTLA-4 levels in the exhausted T cells of triple-negative breast cancer (TNBC) [34]. Simultaneously, genetic blockade of the above three ICIs and high expression of CD56 on chimeric antigen receptor T (CAR-T) cells suppress tumor growth [35]. Therefore, uncovering the relationship between immune checkpoints (ICs) and infiltrating immune cells is essential to prefiguring the response to ICIs [36].
Furthermore, in the different infectious diseases, autoimmune diseases, and cancers, a novel subset of natural regulatory plasma cells with LAG-3 expression can curb immunity through IL-10 production [37]. LAG-3 expression in cancer and immune cells is regulated epigenetically via DNA methylation, which is correlated with the distinct immune cell infiltrates and overall survival (OS) of clear cell renal cell carcinomas (RCCs) [38]. The above results demonstrated further detecting LAG-3 DNA methylation as a predictor for response to LAG-3 inhibitors.
In addition, sLAG-3 has different roles from LAG-3 [25]. sLAG-3 can promote dendritic cells (DCs) to mature [39] and assault cancer cells [40]. The mechanisms uncover that sLAG-3 can activate phosphatidyl inositol 3-kinase/Akt, p42/44 extracellular signal-regulated protein kinase, and p38 mitogen-activated protein kinase pathways to trigger DC functional maturation [41]. The soluble levels of five co-inhibitory immune checkpoint molecules, including CTLA-4, PD-1/PD-L1, TIM-3, and LAG-3 significantly increase in the patients with basal cell carcinoma [42] and Xeroderma Pigmentosum associated malignancies [43]. Moreover, Eftilagimod alpha (EFTI), a soluble LAG-3 protein has good tolerance in combination with pembrolizumab (PD-1 antagonist), which confirms the satisfactory antitumor activity of patients with metastatic melanoma [44]. The above results underscore the therapeutic promise of ICIs and the potential of these proteins as predictors of cancer therapy response and prognosis.
3 Progress of LAG-3 in the Cancer Immunity
LAG-3 protein and mRNA can be observed in various cancers and correlate with clinicopathological parameters and prognosis significance. Moreover, the combination of anti-LAG-3 plus anti-PD-1/PD-L1 antibodies acts upon CD8 T cells, promotes IFN-γ production, and enhances cytotoxic capacity, which has a better therapeutic response for cancer immunotherapy.
3.1 The Expression of LAG-3 and Its Clinical Significance in the Different Cancers
Except for PD-1 and CTLA4, LAG-3 is one of the next generations of co-inhibitory ICs, and has a clinical transformation possibility. LAG-3 expression is located in the cell membranes of activated CD4+ and CD8+ T cells as well as Tregs, but no expression on naive T cells in the infection and cancer [45,46]. In addition, it also expresses in natural killer (NK) cells, B cells and DCs [47]. Overexpression of LAG-3 is detected on the surface of Th1 cells, and regarded as its marker molecule. LAG-3 is closely associated to the formation of Th1 cells and can be upregulated by IL-12. The binding of LAG-3 to the corresponding ligand (sharing the same ligand with CD4) can increase the secretion of IFN-γ and overexpression of IL-2 receptor in Th1 cells, hence fostering the activation and proliferation of Th1 cells [48]. LAG-3 expression in the TME has also been correlated with increased tumor mutational burden (TMB) [49]. For example, a subset of patients with colorectal cancer with high microsatellite instability (MSIhi), exhibit higher TMB and higher levels of immunogenic neoantigen [50]. Multiple ICs including LAG-3, PD-1, PD-L1, CTLA4 and indoleamine 2,3-dioxygenase (IDO) are increasingly expressed in the TME of these MSIhi cancers, compared with in microsatellite stable cancers [49]. The above results may demonstrate why MSIhi cancers are not naturally eradicated, despite a hostile immune microenvironment [49].
The co-inhibitory ICs such as CTLA4, PD-1, TIM-3 and LAG-3 are correlated with T cell exhaustion and immune escape in most tumors, with different levels of expression and different functions. Relatively high levels of LAG-3 expression are observed in bladder cancer, cervical cancer, breast cancer, brain and CNS tumors, and leukemia, compared to the PD-1, CTLA4, and TIM-3 [36]. LAG-3 protein is highly expressed on TILs, associated with higher pathological grade, larger tumor size and positive lymph node status in human head and neck squamous cell carcinoma (HNSCC) [51]. The expression of LAG-3 and TIM-3 is also examined in the TME of 57 patients with classical Hodgkin lymphoma (HL), compared with PD-L1 and PD-1. These results demonstrated that targeting LAG-3 or TIM-3 in combination with an anti-PD-1 antibody may be a choice for treating relapsed/refractory classical HL [52]. Furthermore, LAG-3 is not only expressed in the TILs of non-small cell lung cancer (NSCLC) patients, but also is ectopically expressed in cancer cells and related to the TNM stage [53]. Different expression levels of PD-L1, TIM-3, LAG-3, IL-10 and transforming growth factor-β (TGF-β) are detected in the glioma-associated microglia/macrophages (GAMs) among the patients with glioblastoma. The results also identified that GAMs are the dominant infiltrating immune cells, moreover, GAMs, exhausted T cells, Tregs, and nonfunctional NK cells all promote local immune suppressive TME [54]. CTLA-4 expression is enriched in orbitally invasive basal cell carcinoma (BCC) compared with nodular forms of BCC, whereas LAG-3 expression conforms no difference between these entities [55]. In a study of hepatocellular carcinoma (HCC), the levels of LAG-3, FGL1, PD-L1, and CD8+ T cells have been detected in the peripheral blood of 143 patients with HCC by multiplex immunofluorescence. The results showed that the expression levels of FGL1 and LAG-3 in HCC tissues are higher than those in adjacent normal liver tissues, while the expression levels of PD-L1 and CD8+ are decreased. The high level of FGL1 is closely related to the high density of LAG-3+ cells, but not to PD-L1 [56]. The above demonstrated that the FGL1-LAG-3 pathway is a prospective immunotherapeutic target and can be synergistic with PD-1/PD-L1. Another exciting result found that overexpression of LAG-3 is associated with high TMB, Epstein-Barr virus (EBV) and human papillomavirus (HPV) or endogenous retrovirus (ERV3–2) infection, in multiple cancers. Moreover, LAG-3 inhibitors may be more therapeutic effect than PD-1 pathway blockade in subsets of HPV+ HNSCC, RCC, and glioblastoma [57]. These results may provide an experimental basis for guiding the ongoing clinical trials of LAG-3 blockade.
3.2 The Prognostic Role of LAG-3 in the Different Cancers
Immune checkpoint molecules act as crucial regulators of the immune response, prompting the emergence of ICIs strategies. However, the predictive value of LAG-3 varies widely across cancers, implying significant heterogeneity among tumors even with the same immune phenotype. Furthermore, the different expression patterns of LAG-3 may closely correlate with cancer prognosis and treatment response, providing valuable directions when selecting chemotherapeutic agents (Table 1).
In recent five years, many studies have reported that high expression of LAG-3 in TILs may be tightly related to the poor prognosis, adjuvant chemotherapy resistance and immune escape of some cancers, such as oral SCC [58], EBV-positive and MLH1-defective gastric carcinoma [59], bladder cancer [60], HCC [56], pancreatic ductal adenocarcinoma [61], salivary gland carcinoma [62], and soft tissue sarcoma [63]. Moreover, LAG-3 expressed in the tumor cells also can predict poor prognosis in clear cell RCCs [38]. Surprisingly, patients with co-expression of PD-1, TIM-3, LAG-3, and B and T lymphocyte attenuator (BTLA) mRNA can reflect poor PFS and OS in diffuse large B-cell lymphoma, not otherwise specified (DLBCL, NOS) [64]. Another study identified that both CTLA-4 and LAG-3 mRNA expression levels are independent predictors of poor prognosis in acute myeloid leukemia patients [65].
In the different subtypes of the same cancer, the LAG-3 has different, even paradoxical prognosis significance. For example, serum LAG-3 had a close association with prolonged survival in patients with ER-positive breast cancer [66]. High expression of LAG-3 in TILs favors the outcome of invasive breast carcinomas, notwithstanding their correlation with poor conventional pathologic parameters [67], and improves OS and recurrence-free survival (RFS) in TNBC [68]. But high expression of LAG-3 combined with PD-L1 in the post-neoadjuvant chemotherapical residual tissues predicts poor prognosis of patients with TNBC [69], and high expression of LAG-3 in TILs is an independent predictor of poor prognosis of TNBC and Her-2-enriched subtype [70,71]. The above results conveyed a complicated correlation between LAG-3 and the ER status in breast cancer.
In addition, high expression LAG-3 in TILs is associated with improved OS of esophageal adenocarcinoma [72], but predicts poor prognosis of esophageal SCC [73]. Moreover, a high LAG-3/CD8 index correlates with a worse prognosis of lung adenocarcinoma [74]. However, high LAG-3, but not high LAG-3/CD8 index, predicts improved progression-free survival (PFS) of lung squamous cell carcinomas [74] and disease-specific survival of NSCLC [75]. Furthermore, patients with NSCLC who co-express LAG-3 and PD-L1 in tumor cells display more incredibly durable clinical benefits and better long-term survival following anti-PD-1 therapy [76]. The above results suggested that the prognostic significance of high LAG-3 in TILs has a histotype-dependent in NSCLC and esophageal carcinoma. Another interesting results demonstrated that CD274, LAG3, and IDO1 expressions in TILs significantly forecast better disease-free survival (DFS), and regard as independent prognostic factors of colon cancer with MSI-high [77]. Paradoxically, high expression LAG-3 in TILs is independently associated with poor prognosis, but high expression LAG-3 in tumor cells can predict improved prognosis of colorectal cancer with stage I–III [78]. High expression LAG-3 mRNA also has the paradoxical prognosis significance of serous ovarian cancer [36,79]. Taken together, LAG-3 protein and mRNA in the immune cells and tumor cells have different predictive prognosis significance in the different cancer types, and histotype-dependent patterns. However, the contradictory results about the prognostic value of LAG-3 expression in the same cancer types need more research to confirm.
3.3 Anti-LAG-3 Antibody in the Cancer Immunotherapy
Currently, the field of oncology emphasizes the effectiveness of cancer immunotherapy. Notably, ICs including CTLA4, PD-1, LAG-3, and TIM-3 are considered vital regulators of the immune response, prompting the emergence of immune checkpoint-targeted therapy [36]. These immune checkpoint molecules can down-regulate T-cell function and amplify T-cell exhaustion. It is a promising therapeutic strategy that targets these molecules to enhance T-cell activity.
Although only a minority of patients with cancer have complete responses, the clinical benefits of immunotherapeutic approaches to cancer have been acquired. Combination immunotherapy proposes an attractive avenue for the development of more efficient cancer treatments by increasing the effectiveness and duration of tumor-specific T-cell responses [80]. Due to PD-1 and LAG-3 regulating tumor immune responses, it’s necessary to develop therapies focused on PD-1 and LAG-3 inhibition to improve immune responses in cancer patients. A study has evaluated the safety, tolerability, pharmacokinetics, and pharmacodynamics of combining soluble LAG-3 protein EFTI with PD-1 antagonist pembrolizumab. The combination of EFTI with pembrolizumab is well tolerated and has good antitumor activity [44]. The anti-PD-1/LAG-3 bispecific antibody binds to and internalizes CD4+ T cells, thereby enhancing their effector function (release of granzyme B and IFN-γ) in tumor cells and completely suppressing the tumor in a mouse model. However, treatment data are shown only for pancreatic cancer [44,81]. In the co-culture assay with autologous melanoma cell lines and expanded TILs, anti-LAG-3 combined with anti-PD-1 improves therapy effects through CD8 T cells to induce IFN-γ production and increase cytotoxic capacity [82]. Co-expressing activating (ICOS) and inhibitory (LAG-3, PD-1) molecules are regarded as TAI cell subsets. Via agonistic and antagonist antibodies to co-targeted these molecules on the TAI cells in vivo, they can enhance PD-L1 blockade therapy. Consequently, combined immunotherapy can target these TAI cells and lead to improved survival [80].
Both LAG-3 and one of its ligands, GAL-3, are the targets of emerging immunotherapies. LAG-3 in TILs and GAL-3 in cancer cells are easily observed in nonmethylated mismatch repair-deficient endometrial cancers. Moreover, LAG-3+ lymphocytes have a positive correlation with GAL-3 expression [83]. Herein, immunotherapies targeting LAG-3 and GAL-3 are beneficial in a subset of endometrial cancers. Another interesting research found that FS118 is a newly bispecific, tetravalent antibody [mAb (2)] against human LAG-3 and PD-L1, which has the potential to revive exhausted immune cells and defeat resistance mechanisms of PD-L1 blockade. Further, FS118 can simultaneously bind to LAG-3 and PD-L1 with higher affinity and better activity, compared to the single parts of the mAb (2) in blocking LAG-3- and PD-L1-mediated immune suppression. It reveals an innovative advantage of the bispecific approach over a combination of mAbs and substantiates the further development of FS118 for treating cancer patients [84]. Furthermore, another study showed that the antitumor efficacy with the triple combination of TIM-3, PD-1, and LAG-3 mAbs is much better than any two antibodies. Because the triple combination can synergetically enhance the levels of granzyme B and CD8+ TILs. These data demonstrate that the upregulation of multiple co-inhibitory checkpoint molecules can synchronously restrain the function of hyperactivated T cells in the TME. Thus, it is essential for the combined blockade of PD-1, TIM-3, and LAG-3 for cancer immunotherapy [85]. Besides, therapeutic cancer vaccines can fight against cancer by strengthening the endogenous immune system of patients. For example, the dominant adjuvant effects of polyinosinic-polycytidylic acid poly (I:C) plus LAG-3-Ig (soluble recombinant protein of LAG-3 extracellular domain fused with human IgG Fc region) are associated with an enhanced TILs in the P815 mouse tumor tissues and Th1-type cytokine production of tumor-reactive T cells. Moreover, poly (I:C) plus LAG-3-Ig can downregulate the expression of PD-1, LAG-3, and TIGIT on P1A-specific T cells, which can prevent T cell exhaustion. So, poly (I:C) plus LAG-3-Ig are an innovative combination of adjuvants that can collaboratively amplify the antitumor responses of cancer vaccines by stimulating tumor-specific T-cell responses [86].
3.4 Clinical Trials Targeting LAG-3
Antitumor-related clinical trials related to LAG-3, such as soluble LAG3 Ig (IMP321), anti-LAG3 mAb (BMS986016), and LAG-525 are currently undergoing [87]. LAG-3 as a cancer immune target is also underway in Phase I clinical trials, for example, a single-center trial for hematologic malignancies (Clinical Trial No. NCT02061761). Another single-agent LAG3-specific antibody against solid tumors and a combination trial of LAG-3 blockade and PD-1 blockade (ClinicalTrials.gov, No. NCT01968109) are also underway. The action mechanism of LAG-3-specific monoclonal antibody is to enhance the activity of tumor-specific effector T cells. Although the biological function of LAG-3 is not as extensively studied as PD-1, shreds evidence that the pleiotropic effects of LAG-3 can activate other mechanisms of LAG-3 blockade [88]. Presently, immune combination therapies are now in full swing, with several clinical trials coincidentally combining LAG-3 monoclonal antibody and PD-1 monoclonal antibody, such as Phase I/II study of the LAG-3 inhibitor ieramilimab (LAG525), which is well tolerated as monotherapy and in combination with spartalizumab (anti-PD-1 antibodies). Aid the combined therapy has the modest antitumor activity of patients with advanced/metastatic solid tumors (ClinicalTrials.gov, No. NCT02460224) [89]. In another Phase II/III study, relatlimab (a LAG-3-blocking antibody) and nivolumab (a PD-1-blocking antibody) in combination confirm a greater benefit to PFS than the block of PD-1 alone in patients with previously untreated metastatic melanoma, and demonstrate no new safety signals (ClinicalTrials.gov, No. NCT03470922) [90].
However, a significant concern with dual drug combinations is drug toxicity and patient tolerance, so immune combination therapies have a long way before clinical translation.
4 Conclusion and Future Perspective
The advent of cancer immunotherapy, especially ICIs, has revolutionized cancer therapy, and LAG-3 is anticipated to be a promising cancer therapy target. Oncology therapies associated with CTLA-4 and PD-1/PD-L1 have achieved extraordinary results. However, the clinical significance of LAG-3 remains undefined. The signaling mechanism of LAG-3 is unclear, and its ligands’ biological function is puzzling. LAG-3 plays a key role in the development and progress of cancer. Many studies have found that LAG-3 expression in TILs and TCs may be closely correlated with poor prognosis, chemotherapy resistance and immune evasion in different kinds of cancer; and its prognostic significance is a histotype-dependent pattern, but the contradictory prognostic value of LAG-3 expression in the same cancer types need more evidence to clarify. Currently, drugs targeting LAG-3 are mainly used in combination with PD-1 or alone. Still, combination immunotherapy can improve the effectiveness and duration of tumor-specific T-cell responses, clearly providing an attractive avenue for more effective cancer treatment. In vitro and in vivo mice tumor models, such as colorectal cancer, ovarian cancer, melanoma, and lymphocytic leukemia, simultaneous blockade of PD-1 or PD-L1 and LAG-3 can reverse immune suppression and enhance T-cell activity, thus having a therapeutic response for cancer immunotherapy. Antibody-mediated blockers of LAG-3 and/or combination with PD-1/PD-L1 blockade in cancer patients are currently underway. However, more detailed and comprehensive elucidation of the functional properties of LAG-3 is needed to scientifically and rationally develop LAG-3 targeting strategies to achieve better clinical results and benefit more patients.
Author Contributions: Conceived and designed the analysis: Pen M., Chen HL.; Collected the data: Cui X.; Contributed data or analysis tools: Cui X.; Wrote the paper: Cui X., Chen HL.; All authors reviewed the results and approved the final version of the manuscript.
Ethics Approval and Informed Consent Statement: Not applicable.
Funding Statement: The authors received no specific funding for this study.
Conflicts of Interest: The authors declare that they have no conflicts of interest to report regarding the present study.
References
1. Galluzzi, L., Vacchelli, E., Bravo-San Pedro, J. M., Buque, A., Senovilla, L. et al. (2014). Classification of current anticancer immunotherapies. Oncotarget, 5(24), 12472–12508. DOI 10.18632/oncotarget.2998. [Google Scholar] [CrossRef]
2. Sharma, P., Allison, J. P. (2015). The future of immune checkpoint therapy. Science, 348(6230), 56–61. DOI 10.1126/science.aaa8172. [Google Scholar] [CrossRef]
3. Anderson, A. C., Joller, N., Kuchroo, V. K. (2016). Lag-3, Tim-3, and TIGIT: Co-inhibitory receptors with specialized functions in immune regulation. Immunity, 44(5), 989–1004. DOI 10.1016/j.immuni.2016.05.001. [Google Scholar] [CrossRef]
4. Andrews, L. P., Yano, H., Vignali, D. A. A. (2019). Inhibitory receptors and ligands beyond PD-1, PD-L1 and CTLA-4: Breakthroughs or backups. Nature Immunology, 20(11), 1425–1434. DOI 10.1038/s41590-019-0512-0. [Google Scholar] [CrossRef]
5. Burugu, S., Gao, D., Leung, S., Chia, S. K., Nielsen, T. O. (2017). LAG-3+ tumor infiltrating lymphocytes in breast cancer: Clinical correlates and association with PD-1/PD-L1+ tumors. Annals of Oncology, 28(12), 2977–2984. DOI 10.1093/annonc/mdx557. [Google Scholar] [CrossRef]
6. Ascione, A., Arenaccio, C., Mallano, A., Flego, M., Gellini, M. et al. (2019). Development of a novel human phage display-derived anti-LAG3 scFv antibody targeting CD8+ T lymphocyte exhaustion. BMC Biotechnology, 19(1), 67. DOI 10.1186/s12896-019-0559-x. [Google Scholar] [CrossRef]
7. Triebel, F., Jitsukawa, S., Baixeras, E., Roman-Roman, S., Genevee, C. et al. (1990). LAG-3, a novel lymphocyte activation gene closely related to CD4. Journal of Experimental Medicine, 171(5), 1393–1405. DOI 10.1084/jem.171.5.1393. [Google Scholar] [CrossRef]
8. Maruhashi, T., Sugiura, D., Okazaki, I. M., Okazaki, T. (2020). LAG-3: From molecular functions to clinical applications. Journal for ImmunoTherapy of Cancer, 8(2), e001014. DOI 10.1136/jitc-2020-001014. [Google Scholar] [CrossRef]
9. Lui, Y., Davis, S. J. (2018). LAG-3: A very singular immune checkpoint. Nature Immunology, 19(12), 1278–1279. DOI 10.1038/s41590-018-0257-1. [Google Scholar] [CrossRef]
10. Wang, J., Ti, Y., Wang, Y., Guo, G., Jiang, H. et al. (2018). LAG-3 represents a marker of CD4+ T cells with regulatory activity in patients with bone fracture.Immunological Investigations, 47(5), 492–503. DOI 10.1080/08820139.2018.1458107. [Google Scholar] [CrossRef]
11. Workman, C. J., Rice, D. S., Dugger, K. J., Kurschner, C., Vignali, D. A. (2002). Phenotypic analysis of the murine CD4-related glycoprotein, CD223 (LAG-3). European Journal of Immunology, 32(8), 2255–2263. DOI 10.1002/1521-4141(200208)32:8<2255::AID-IMMU2255>3.0.CO;2-A. [Google Scholar] [CrossRef]
12. Moerdler, S., Ewart, M., Friedman, D. L., Kelly, K., Pei, Q. et al. (2020). LAG-3 is expressed on a majority of tumor infiltrating lymphocytes in pediatric hodgkin lymphoma. Leukemia & Lymphoma, 62(3), 1–8. DOI 10.1080/10428194.2020.1839651. [Google Scholar] [CrossRef]
13. Huard, B., Prigent, P., Tournier, M., Bruniquel, D., Triebel, F. (1995). CD4/major histocompatibility complex class II interaction analyzed with CD4-and lymphocyte activation gene-3 (LAG-3)-Ig fusion proteins. European Journal of Immunology, 25(9), 2718–21. DOI 10.1002/eji.1830250949. [Google Scholar] [CrossRef]
14. Bauché, D., Joyce-Shaikh, B., Jain, R., Grein, J., Ku, K. S. et al. (2018). LAG3+ regulatory T cells restrain interleukin-23-producing CX3CR1+ gut-resident macrophages during group 3 innate lymphoid cell-driven colitis. Immunity, 49(2), 342–352.e5. DOI 10.1016/j.immuni.2018.07.007. [Google Scholar] [CrossRef]
15. Cook, K. D., Whitmire, J. K. (2016). LAG-3 confers a competitive disadvantage upon antiviral CD8+ T cell responses. The Journal of Immunology, 197(1), 119–127. DOI 10.4049/jimmunol.1401594. [Google Scholar] [CrossRef]
16. He, Y., Wang, Y., Zhao, S., Zhao, C., Zhou, C. et al. (2018). sLAG-3 in non-small-cell lung cancer patients’ serum. OncoTargets and Therapy, 11, 4781–4784. DOI 10.2147/ott.S164178. [Google Scholar] [CrossRef]
17. Andrews, L. P., Marciscano, A. E., Drake, C. G., Vignali, D. A. (2017). LAG3 (CD223) as a cancer immunotherapy target. Immunological Reviews, 276(1), 80–96. DOI 10.1111/imr.12519. [Google Scholar] [CrossRef]
18. Maruhashi, T., Sugiura, D., Okazaki, I. M., Shimizu, K., Maeda, T. K. et al. (2022). Binding of LAG-3 to stable peptide-MHC class II limits T cell function and suppresses autoimmunity and anti-cancer immunity. Immunity, 55(5), 912–924.e8. DOI 10.1016/j.immuni.2022.03.013. [Google Scholar] [CrossRef]
19. Wang, J., Sanmamed, M. F., Datar, I., Su, T. T., Ji, L. et al. (2019). Fibrinogen-like protein 1 is a major immune inhibitory ligand of LAG-3. Cell, 176(1–2), 334–347.e12. DOI 10.1016/j.cell.2018.11.010. [Google Scholar] [CrossRef]
20. Dumic, J., Dabelic, S., Flogel, M. (2005). Galectin-3: An open-ended story. Biochim Biophys Acta, 1760(4), 616–635. DOI 10.1016/j.bbagen.2005.12.020. [Google Scholar] [CrossRef]
21. Kouo, T., Huang, L., Pucsek, A. B., Cao, M., Solt, S. et al. (2015). Galectin-3 shapes antitumor immune responses by suppressing CD8+ T cells via LAG-3 and inhibiting expansion of plasmacytoid dendritic cells. Cancer Immunology Research, 3(4), 412–423. DOI 10.1158/2326-6066.CIR-14-0150. [Google Scholar] [CrossRef]
22. Liu, W., Tang, L., Zhang, G., Wei, H., Cui, Y. et al. (2004). Characterization of a novel C-type lectin-like gene, LSECtin: Demonstration of carbohydrate binding and expression in sinusoidal endothelial cells of liver and lymph node. Journal of Biological Chemistry, 279(18), 18748–18758. DOI 10.1074/jbc.M311227200. [Google Scholar] [CrossRef]
23. Xu, F., Liu, J., Liu, D., Liu, B., Wang, M. et al. (2014). LSECtin expressed on melanoma cells promotes tumor progression by inhibiting antitumor T-cell responses. Cancer Research, 74(13), 3418–3428. DOI 10.1158/0008-5472.CAN-13-2690. [Google Scholar] [CrossRef]
24. McKinney, E. F., Lee, J. C., Jayne, D. R., Lyons, P. A., Smith, K. G. (2015). T-cell exhaustion, co-stimulation and clinical outcome in autoimmunity and infection. Nature, 523(7562), 612–626. DOI 10.1038/nature14468. [Google Scholar] [CrossRef]
25. Triebel, F. (2003). LAG-3: A regulator of T-cell and DC responses and its use in therapeutic vaccination. Trends in Immunology, 24(12), 619–622. DOI 10.1016/j.it.2003.10.001. [Google Scholar] [CrossRef]
26. Sun, H., Sun, C., Xiao, W. (2014). Expression regulation of co-inhibitory molecules on human natural killer cells in response to cytokine stimulations. Cytokine, 65(1), 33–41. DOI 10.1016/j.cyto.2013.09.016. [Google Scholar] [CrossRef]
27. Shin, H., Blackburn, S. D., Intlekofer, A. M., Kao, C., Angelosanto, J. M. et al. (2009). A role for the transcriptional repressor blimp-1 in CD8+ T cell exhaustion during chronic viral infection. Immunity, 31(2), 309–320. DOI 10.1016/j.immuni.2009.06.019. [Google Scholar] [CrossRef]
28. Man, K., Gabriel, S. S., Liao, Y., Gloury, R., Preston, S. et al. (2017). Transcription factor IRF4 promotes CD8+ T cell exhaustion and limits the development of memory-like T cells during chronic infection. Immunity, 47(6), 1129–1141.e5. DOI 10.1016/j.immuni.2017.11.021. [Google Scholar] [CrossRef]
29. Wu, J., Zhang, H., Shi, X., Xiao, X., Fan, Y. et al. (2017). Ablation of transcription factor IRF4 promotes transplant acceptance by driving allogenic CD4+ T cell dysfunction. Immunity, 47(6), 1114–1128.e6. DOI 10.1016/j.immuni.2017.11.003. [Google Scholar] [CrossRef]
30. Adrianzen, Herrera, D., Ashai, N., Perez-Soler, R., Cheng, H. (2019). Nanoparticle albumin bound-paclitaxel for treatment of advanced non-small cell lung cancer: An evaluation of the clinical evidence. Expert Opinion on Pharmacotherapy, 20(1), 95–102. DOI 10.1080/14656566.2018.1546290. [Google Scholar] [CrossRef]
31. Chen, J., Lopez-Moyado, I. F., Seo, H., Lio, C. J., Hempleman, L. J. et al. (2019). NR4A transcription factors limit CAR T cell function in solid tumours. Nature, 567(7749), 530–534. DOI 10.1038/s41586-019-0985-x. [Google Scholar] [CrossRef]
32. Khan, O., Giles, J. R., McDonald, S., Manne, S., Ngiow, S. F. et al. (2019). TOX transcriptionally and epigenetically programs CD8+ T cell exhaustion. Nature, 571(7764), 211–218. DOI 10.1038/s41586-019-1325-x. [Google Scholar] [CrossRef]
33. Liu, X., Wang, Y., Lu, H., Li, J., Yan, X. et al. (2019). Genome-wide analysis identifies NR4A1 as a key mediator of T cell dysfunction. Nature, 567(7749), 525–529. DOI 10.1038/s41586-019-0979-8. [Google Scholar] [CrossRef]
34. Xu, L., Li, C. (2022). Single-cell transcriptome analysis reveals the M2 macrophages and exhausted T cells and intratumoral heterogeneity in triple-negative breast cancer. Anti-Cancer Agents in Medicinal Chemistry, 22(2), 294–312. DOI 10.2174/1871520621666210618100857. [Google Scholar] [CrossRef]
35. Zou, F., Lu, L., Liu, J., Xia, B., Zhang, W. et al. (2019). Engineered triple inhibitory receptor resistance improves anti-tumor CAR-T cell performance via CD56. Nature Communication, 10(1), 4109. DOI 10.1038/s41467-019-11893-4. [Google Scholar] [CrossRef]
36. Tu, L., Guan, R., Yang, H., Zhou, Y., Hong, W. et al. (2020). Assessment of the expression of the immune checkpoint molecules PD-1, CTLA4, TIM-3 and LAG-3 across different cancers in relation to treatment response, tumor-infiltrating immune cells and survival. International Journal of Cancer, 147(2), 423–439. DOI 10.1002/ijc.32785. [Google Scholar] [CrossRef]
37. Lino, A. C., Dang, V. D., Lampropoulou, V., Welle, A., Joedicke, J. et al. (2018). LAG-3 inhibitory receptor expression identifies immunosuppressive natural regulatory plasma cells. Immunity, 49(1), 120–133.e9. DOI 10.1016/j.immuni.2018.06.007. [Google Scholar] [CrossRef]
38. Klümper, N., Ralser, D. J., Bawden, E. G., Landsberg, J., Zarbl, R. et al. (2020). LAG3 (LAG-3, CD223) DNA methylation correlates with LAG3 expression by tumor and immune cells, immune cell infiltration, and overall survival in clear cell renal cell carcinoma. Journal for Immuno Therapy of Cancer, 8(1), e000552. DOI 10.1136/jitc-2020-000552. [Google Scholar] [CrossRef]
39. Cappello, P., Triebel, F., Iezzi, M., Caorsi, C., Quaglino, E. et al. (2003). LAG-3 enables DNA vaccination to persistently prevent mammary carcinogenesis in HER-2/neu transgenic BALB/c mice. Cancer Research, 63(10), 2518–2525. [Google Scholar]
40. Buisson, S., Triebel, F. (2003). MHC class II engagement by its ligand LAG-3 (CD223) leads to a distinct pattern of chemokine and chemokine receptor expression by human dendritic cells. Vaccine, 21(9–10), 862–868. DOI 10.1016/s0264-410x(02)00533-9. [Google Scholar] [CrossRef]
41. Andreae, S., Buisson, S., Triebel, F. (2003). MHC class II signal transduction in human dendritic cells induced by a natural ligand, the LAG-3 protein (CD223). Blood, 102(6), 2130–2137. DOI 10.1182/blood-2003-01-0273. [Google Scholar] [CrossRef]
42. Malinga, N. Z., Siwele, S. C., Steel, H. C., Kwofie, L. L. I., Meyer, P. W. A. et al. (2022). Systemic levels of the soluble co-inhibitory immune checkpoints, CTLA-4, LAG-3, PD-1/PD-l1 and TIM-3 are markedly increased in basal cell carcinoma. Translational Oncology, 19, 101384. DOI 10.1016/j.tranon.2022.101384. [Google Scholar] [CrossRef]
43. Kgokolo, M. C. M., Anderson, K., Siwele, S. C., Steel, H. C., Kwofie, L. L. I. et al. (2022). Elevated levels of soluble CTLA-4, PD-1, PD-L1, LAG-3 and TIM-3 and systemic inflammatory stress as potential contributors to immune suppression and generalized tumorigenesis in a cohort of South African xeroderma pigmentosum patients. Frontiers in Oncology, 12, 819790. DOI 10.3389/fonc.2022.819790. [Google Scholar] [CrossRef]
44. Atkinson, V., Khattak, A., Haydon, A., Eastgate, M., Roy, A. et al. (2020). Eftilagimod alpha, a soluble lymphocyte activation gene-3 (LAG-3) protein plus pembrolizumab in patients with metastatic melanoma. Journal for ImmunoTherapy of Cancer, 8(2), e001681. DOI 10.1136/jitc-2020-001681. [Google Scholar] [CrossRef]
45. Durham, N. M., Nirschl, C. J., Jackson, C. M., Elias, J., Kochel, C. M. et al. (2014). Lymphocyte activation gene 3 (LAG-3) modulates the ability of CD4 T-cells to be suppressed in vivo. PLoS One, 9(11), e109080. DOI 10.1371/journal.pone.0109080. [Google Scholar] [CrossRef]
46. Pena, J., Jones, N. G., Bousheri, S., Bangsberg, D. R., Cao, H. (2014). Lymphocyte activation gene-3 expression defines a discrete subset of HIV-specific CD8+ T cells that is associated with lower viral load. AIDS Research and Human Retroviruses, 30(6), 535–541. DOI 10.1089/AID.2012.0195. [Google Scholar] [CrossRef]
47. Kisielow, M., Kisielow, J., Capoferri-Sollami, G., Karjalainen, K. (2005). Expression of lymphocyte activation gene 3 (LAG-3) on B cells is induced by T cells. European Journal of Immunology, 35(7), 2081–2088. DOI 10.1002/eji.200526090. [Google Scholar] [CrossRef]
48. Sierro, S., Romero, P., Speiser, D. E. (2011). The CD4-like molecule LAG-3, biology and therapeutic applications. Expert Opinion on Therapeutic Targets, 15(1), 91–101. DOI 10.1517/14712598.2011.540563. [Google Scholar] [CrossRef]
49. Llosa, N. J., Cruise, M., Tam, A., Wicks, E. C., Hechenbleikner, E. M. et al. (2015). The vigorous immune microenvironment of microsatellite instable colon cancer is balanced by multiple counter-inhibitory checkpoints. Cancer Discovery, 5(1), 43–51. DOI 10.1158/2159-8290.CD-14-0863. [Google Scholar] [CrossRef]
50. Shan, C., Li, X., Zhang, J. (2020). Progress of immune checkpoint LAG-3 in immunotherapy. Oncology Letters, 20(5), 207. DOI 10.3892/ol.2020.12070. [Google Scholar] [CrossRef]
51. Deng, W. W., Mao, L., Yu, G. T., Bu, L. L., Ma, S. R. et al. (2016). LAG-3 confers poor prognosis and its blockade reshapes antitumor response in head and neck squamous cell carcinoma. Oncoimmunology, 5(11), e1239005. DOI 10.1080/2162402x.2016.1239005. [Google Scholar] [CrossRef]
52. El Halabi, L., Adam, J., Gravelle, P., Marty, V., Danu, A. et al. (2020). Expression of the immune checkpoint regulators LAG-3 and TIM-3 in classical hodgkin lymphoma. Clinical Lymphoma Myeloma & Leukemia, 21(4), 257–266.e3. DOI 10.1016/j.clml.2020.11.009. [Google Scholar] [CrossRef]
53. Ma, C., Sun, X., Shen, D., Sun, Y., Guan, N. et al. (2020). Ectopic expression of LAG-3 in non-small-cell lung cancer cells and its clinical significance. Journal of Clinical Laboratory Analysis, 34(6), e23244. DOI 10.1002/jcla.23244. [Google Scholar] [CrossRef]
54. Fu, W., Wang, W., Li, H., Jiao, Y., Huo, R. et al. (2020). Single-cell atlas reveals complexity of the immunosuppressive microenvironment of initial and recurrent glioblastoma. Frontiers in Immunology, 11, 835. DOI 10.3389/fimmu.2020.00835. [Google Scholar] [CrossRef]
55. Lajoie, J. E., Lau, K. W., Adam, A. P., Wladis, E. J. (2020). Cytotoxic T-lymphocyte-associated protein-4 and lymphocyte activation gene-3 expression in orbitally-invasive vs. nodular basal cell carcinoma. Ophthalmic Plastic and Reconstructive Surgery, 37(3S), S109–S111. DOI 10.1097/iop.0000000000001853. [Google Scholar] [CrossRef]
56. Guo, M., Yuan, F., Qi, F., Sun, J., Rao, Q. et al. (2020). Expression and clinical significance of LAG-3, FGL1, PD-l1 and CD8+T cells in hepatocellular carcinoma using multiplex quantitative analysis. Journal of Translational Medicine, 18(1), 306. DOI 10.1186/s12967-020-02469-8. [Google Scholar] [CrossRef]
57. Panda, A., Rosenfeld, J. A., Singer, E. A., Bhanot, G., Ganesan, S. (2020). Genomic and immunologic correlates of LAG-3 expression in cancer. Oncoimmunology, 9(1), 1756116. DOI 10.1080/2162402x.2020.1756116. [Google Scholar] [CrossRef]
58. Wang, H., Mao, L., Zhang, T., Zhang, L., Wu, Y. et al. (2019). Altered expression of TIM-3, LAG-3, IDO, PD-L1, and CTLA-4 during nimotuzumab therapy correlates with responses and prognosis of oral squamous cell carcinoma patients. Journal of Oral Pathology & Medicine, 48(8), 669–676. DOI 10.1111/jop.12883. [Google Scholar] [CrossRef]
59. Lv, K., Li, R., Cao, Y., Gu, Y., Liu, X. et al. (2021). Lymphocyte-activation gene 3 expression associates with poor prognosis and immunoevasive contexture in epstein-barr virus-positive and MLH1-defective gastric cancer patients. International Journal of Cancer, 148(3), 759–768. DOI 10.1002/ijc.33358. [Google Scholar] [CrossRef]
60. Zeng, H., Zhou, Q., Wang, Z., Zhang, H., Liu, Z. et al. (2020). Stromal LAG-3+ cells infiltration defines poor prognosis subtype muscle-invasive bladder cancer with immunoevasive contexture. Journal for Immuno Therapy of Cancer, 8(1), e000651. DOI 10.1136/jitc-2020-000651. [Google Scholar] [CrossRef]
61. Seifert, L., Plesca, I., Muller, L., Sommer, U., Heiduk, M. et al. (2021). LAG-3-expressing tumor-infiltrating T cells are associated with reduced disease-free survival in pancreatic cancer. Cancers, 13(6), 1297. DOI 10.3390/cancers13061297. [Google Scholar] [CrossRef]
62. Arolt, C., Meyer, M., Ruesseler, V., Nachtsheim, L., Wuerdemann, N. et al. (2020). Lymphocyte activation gene 3 (LAG3) protein expression on tumor-infiltrating lymphocytes in aggressive and TP53-mutated salivary gland carcinomas. Cancer Immunology Immunotherapy, 69(7), 1363–1373. DOI 10.1007/s00262-020-02551-6. [Google Scholar] [CrossRef]
63. Que, Y., Fang, Z., Guan, Y., Xiao, W., Xu, B. et al. (2019). LAG-3 expression on tumor-infiltrating T cells in soft tissue sarcoma correlates with poor survival. Cancer Biology & Medicine, 16(2), 331–340. DOI 10.20892/j.issn.2095-3941.2018.0306. [Google Scholar] [CrossRef]
64. Liu, X. Y., Yuan, X. L., Ma, R. J., Xu, H., Yang, S. W. et al. (2020). Expression of PD-1, TIM-3, LAG-3 and BTLA in diffuse large B-cell lymphoma and its effect on prognosis. Chinese Medical Journal, 100(36), 2846–2853. DOI 10.3760/cma.j.cn112137-20200107-00045. [Google Scholar] [CrossRef]
65. Radwan, S. M., Elleboudy, N. S., Nabih, N. A., Kamal, A. M. (2020). The immune checkpoints cytotoxic T lymphocyte antigen-4 and lymphocyte activation gene-3 expression is up-regulated in acute myeloid leukemia. HLA, 96(1), 3–12. DOI 10.1111/tan.13872. [Google Scholar] [CrossRef]
66. Triebel, F., Hacene, K., Pichon, M. F. (2006). A soluble lymphocyte activation gene-3 (sLAG-3) protein as a prognostic factor in human breast cancer expressing estrogen or progesterone receptors. Cancer Letter, 235(1), 147–153. DOI 10.1016/j.canlet.2005.04.015. [Google Scholar] [CrossRef]
67. Bagbudar, S., Karanlik, H., Cabioglu, N., Bayram, A., Ibis, K. et al. (2022). Prognostic implications of immune infiltrates in the breast cancer microenvironment: The role of expressions of CTLA-4, PD-1, and LAG-3. Applied Immunohistochemistry & Molecular Morphology, 30(2), 99–107. DOI 10.1097/PAI.0000000000000978. [Google Scholar] [CrossRef]
68. Stovgaard, E. S., Kumler, I., List-Jensen, K., Roslind, A., Christensen, I. J. et al. (2022). Prognostic and clinicopathologic associations of LAG-3 expression in triple-negative breast cancer. Applied Immunohistochemistry & Molecular Morphology, 30(1), 62–71. DOI 10.1097/PAI.0000000000000954. [Google Scholar] [CrossRef]
69. Wang, Y., Dong, T., Xuan, Q., Zhao, H., Qin, L. et al. (2018). Lymphocyte-activation gene-3 expression and prognostic value in neoadjuvant-treated triple-negative breast cancer. Journal of Breast Cancer, 21(2), 124–133. DOI 10.4048/jbc.2018.21.2.124. [Google Scholar] [CrossRef]
70. Liu, Q., Qi, Y., Zhai, J., Kong, X., Wang, X. et al. (2021). Molecular and clinical characterization of LAG3 in breast cancer through 2994 samples. Frontiers in Immunology, 12, 599207. DOI 10.3389/fimmu.2021.599207. [Google Scholar] [CrossRef]
71. Asano, Y., Kashiwagi, S., Takada, K., Ishihara, S., Goto, W. et al. (2022). Clinical significance of expression of immunoadjuvant molecules (LAG-3, TIM-3, OX-40) in neoadjuvant chemotherapy for breast cancer. Anticancer Research, 42(1), 125–136. DOI 10.21873/anticanres.15466. [Google Scholar] [CrossRef]
72. Gebauer, F., Kramer, M., Bruns, C., Schlosser, H. A., Thelen, M. et al. (2020). Lymphocyte activation gene-3 (LAG3) mRNA and protein expression on tumour infiltrating lymphocytes (TILs) in oesophageal adenocarcinoma. Journal of Cancer Research and Clinical Oncology, 146(9), 2319–2327. DOI 10.1007/s00432-020-03295-7. [Google Scholar] [CrossRef]
73. Wang, W., Chen, D., Zhao, Y., Zhao, T., Wen, J. et al. (2019). Characterization of LAG-3, CTLA-4, and CD8+ TIL density and their joint influence on the prognosis of patients with esophageal squamous cell carcinoma. The Annals of Translational Medicine, 7(23), 776. DOI 10.21037/atm.2019.11.38. [Google Scholar] [CrossRef]
74. Shepherd, D. J., Tabb, E. S., Kunitoki, K., Zhang, M. L., Kem, M. et al. (2021). Lymphocyte-activation gene 3 in non-small-cell lung carcinomas: Correlations with clinicopathologic features and prognostic significance. Modern Pathology, 35(5), 615–624. DOI 10.1038/s41379-021-00974-9. [Google Scholar] [CrossRef]
75. Hald, S. M., Rakaee, M., Martinez, I., Richardsen, E., Al-Saad, S. et al. (2018). LAG-3 in non-small-cell lung cancer: Expression in primary tumors and metastatic lymph nodes is associated with improved survival. Clinical Lung Cancer, 19(3), 249–259.e2. DOI 10.1016/j.cllc.2017.12.001. [Google Scholar] [CrossRef]
76. Jung, E. H., Jang, H. R., Kim, S. H., Suh, K. J., Kim, Y. J. et al. (2021). Tumor LAG-3 and NY-ESO-1 expression predict durable clinical benefits of immune checkpoint inhibitors in advanced non-small cell lung cancer. Thoracic Cancer, 12(5), 619–630. DOI 10.1111/1759-7714.13834. [Google Scholar] [CrossRef]
77. Lee, S. J., Jun, S. Y., Lee, I. H., Kang, B. W., Park, S. Y. et al. (2018). CD274, LAG3, and IDO1 expressions in tumor-infiltrating immune cells as prognostic biomarker for patients with MSI-high colon cancer. Journal of Cancer Research and Clinical Oncology, 144(6), 1005–1014. DOI 10.1007/s00432-018-2620-x. [Google Scholar] [CrossRef]
78. Al-Badran, S. S., Grant, L., Campo, M. V., Inthagard, J., Pennel, K. et al. (2021). Relationship between immune checkpoint proteins, tumour microenvironment characteristics, and prognosis in primary operable colorectal cancer. Journal of Pathology Clinical Research, 7(2), 121–134. DOI 10.1002/cjp2.193. [Google Scholar] [CrossRef]
79. James, N. E., Miller, K., LaFranzo, N., Lips, E., Woodman, M. et al. (2021). Immune modeling analysis reveals immunologic signatures associated with improved outcomes in high grade serous ovarian cancer. Frontiers in Oncology, 11, 622182. DOI 10.3389/fonc.2021.622182. [Google Scholar] [CrossRef]
80. Beyrend, G., van der Gracht, E., Yilmaz, A., van Duikeren, S., Camps, M. et al. (2019). PD-L1 blockade engages tumor-infiltrating lymphocytes to co-express targetable activating and inhibitory receptors. Journal for Immuno Therapy of Cancer, 7(1), 217. DOI 10.1186/s40425-019-0700-3. [Google Scholar] [CrossRef]
81. Cebada, J., Flores, A., Bandala, C., Lizaliturri-Flores, I., Villa-Ruano, N. et al. (2020). Bispecific anti-PD-1/LAG-3 antibodies for treatment of advanced or metastatic solid tumors: A patent evaluation of US2018326054. Expert Opinion on Therapeutic Patents, 30(7), 487–494. DOI 10.1080/13543776.2020.1767071. [Google Scholar] [CrossRef]
82. Gestermann, N., Saugy, D., Martignier, C., Tillé, L., Fuertes Marraco, S. A. et al. (2020). LAG-3 and PD-1 + LAG-3 inhibition promote anti-tumor immune responses in human autologous melanoma/T cell co-cultures. Oncoimmunology, 9(1), 1736792. DOI 10.1080/2162402x.2020.1736792. [Google Scholar] [CrossRef]
83. Friedman, L. A., Ring, K. L., Mills, A. M. (2020). LAG-3 and GAL-3 in endometrial carcinoma: Emerging candidates for immunotherapy. International Journal of Gynecological Pathology, 39(3), 203–212. DOI 10.1097/pgp.0000000000000608. [Google Scholar] [CrossRef]
84. Kraman, M., Faroudi, M., Allen, N. L., Kmiecik, K., Gliddon, D. et al. (2020). FS118, a bispecific antibody targeting LAG-3 and PD-L1, enhances T-cell activation resulting in potent antitumor activity. Clinical Cancer Research, 26(13), 3333–3344. DOI 10.1158/1078-0432.Ccr-19-3548. [Google Scholar] [CrossRef]
85. Yang, M., Du, W., Yi, L., Wu, S., He, C. et al. (2020). Checkpoint molecules coordinately restrain hyperactivated effector T cells in the tumor microenvironment. Oncoimmunology, 9(1), 1708064. DOI 10.1080/2162402x.2019.1708064. [Google Scholar] [CrossRef]
86. Kano, Y., Iguchi, T., Matsui, H., Adachi, K., Sakoda, Y. et al. (2016). Combined adjuvants of poly (I:C) plus LAG-3-Ig improve antitumor effects of tumor-specific T cells, preventing their exhaustion. Cancer Science, 107(4), 398–406. DOI 10.1111/cas.12861. [Google Scholar] [CrossRef]
87. Wang, M., Du, Q., Jin, J., Wei, Y., Lu, Y. et al. (2021). LAG3 and its emerging role in cancer immunotherapy. Clinical and Translational Medicine, 11(3), e365. DOI 10.1002/ctm2.365. [Google Scholar] [CrossRef]
88. He, Y., Rivard, C. J., Rozeboom, L., Yu, H., Ellison, K. et al. (2016). Lymphocyte-activation gene-3, an important immune checkpoint in cancer. Cancer Science, 107(9), 1193–1197. DOI 10.1111/cas.12986. [Google Scholar] [CrossRef]
89. Schoffski, P., Tan, D. S. W., Martin, M., Ochoa-de-Olza, M., Sarantopoulos, J. et al. (2022). Phase I/II study of the LAG-3 inhibitor ieramilimab (LAG525) ± anti-PD-1 spartalizumab (PDR001) in patients with advanced malignancies. Journal for ImmunoTherapy of Cancer, 10(2), e003776. DOI 10.1136/jitc-2021-003776. [Google Scholar] [CrossRef]
90. Tawbi, H. A., Schadendorf, D., Lipson, E. J., Ascierto, P. A., Matamala, L. et al. (2022). Relatlimab and nivolumab vs. nivolumab in untreated advanced melanoma. New England Journal of Medicine, 386(1), 24–34. DOI 10.1056/NEJMoa2109970. [Google Scholar] [CrossRef]
Cite This Article
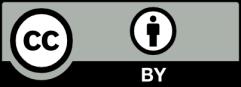
This work is licensed under a Creative Commons Attribution 4.0 International License , which permits unrestricted use, distribution, and reproduction in any medium, provided the original work is properly cited.