Open Access
ARTICLE
Icotinib Enhanced Radiosensitization of Nasopharyngeal Carcinoma by Inhibiting the Expression of Epidermal Growth Factor Receptor
1
Department of Radiotherapy, Taizhou Central Hospital (Taizhou University Hospital), Taizhou, China
2
Department of Radiotherapy, Ningbo Medical Center Lihuili Hospital, Ningbo, China
3
WeiMai Technology Limited Company, Hangzhou, China
* Corresponding Author: Wei Hu. Email:
# These authors contributed equally to this work
Oncologie 2022, 24(3), 553-563. https://doi.org/10.32604/oncologie.2022.023025
Received 06 April 2022; Accepted 21 June 2022; Issue published 19 September 2022
Abstract
Epidermal growth factor receptor (EGFR) is frequently overexpressed in multiple malignancies. Icotinib (IH), a new EGFR tyrosine kinase inhibitor, enhances radiosensitivity in various types of cancer, but its effect on nasopharyngeal carcinoma (NPC) remains unclear. Total 115 NPC tissue sections and 30 nasopharyngitis tissue sections were enrolled. The correlation of EGFR expression and clinicopathologic features of NPC was analyzed. Survival analysis was calculated by using univariate and multivariate regression analysis. A radioresistant NPC cell line, CNE-2R, was established with a gradient irradiation schedule. Cell viability, colony formation and EGFR expression of CNE-2/2R cells were examined. Significant higher expression of EGFR was observed in NPC tissues than chronic nasopharyngitis lesions. EGFR expression was significantly correlated with both tumor stage (P < 0.001) and tumor-node-metastasis stage of NPC (P = 0.006). EGFR expression was an independent prognostic factor of disease-free survival (P = 0.047) and the overall survival of NPC (P = 0.016). Cell viability was higher in CNE-2R than CNE-2 on days 1, 2, 4, and 6 after radiation of 4 Gy. The colony number of CNE-2R was significantly higher than that of CNE-2 (P < 0.05), while IH enhanced the radiosensitizing effect of CNE-2R with lower survival fraction (P < 0.05). EGFR mRNA and protein expression levels were significantly higher in CNE-2R cells compared to CNE-2 cells, but significantly decreased after IH treatment (all P < 0.05). In conclusion, high EGFR expression is a poor prognostic factor for NPC patients. IH enhances the radiosensitivity of CNE-2R cells and reduce EGFR expression.Keywords
Nasopharyngeal carcinoma (NPC) is a heterogeneous epithelial tumor in the head and neck [1]. NPC is highly prevalent in East and Southeast Asia with a high incidence rate of 15–50 cases per 100,000 population compared to Western countries [2]. According to different histological subtypes, non-keratinizing subtype is more prevalent in the southern China and squamous cell cancers are frequently observed in the USA and Europe [3]. Radiotherapy with or without chemotherapy is the predominant treatment modality for NPC, especially for local advanced NPC. Distant metastasis and local progression contribute to poor outcome of NPC [4,5]. Residual irradiated NPC cells with high radioresistance is the most common cause of unfavorable prognosis [6,7]. Furthermore, severe therapeutic side effects, including myelosuppression and mucositis, frequently lead to the reduction of treatment tolerance and the quality of life [8]. Therefore, it is important to explore new effective therapeutic strategies that increase radiosensitivity and reduce side effects.
Epidermal growth factor receptor (EGFR) is a transmembrane glycoprotein that belongs to ErbB receptor tyrosine kinase family, which enhances cancer cell proliferation, differentiation, metastasis, and angiogenesis [9]. EGFR overexpression has been demonstrated in NPC, and the inhibition of EGFR signal pathway could improve the radiosensitivity of NPC [10,11]. Huang et al. [12] reported that concurrent administration of EGFR monoclonal antibodies nimotuzumab and celecoxib enhanced the radiosensitivity of NPC cells. Clinical studies have shown that cetuximab or nimotuzumab enhanced the efficacy of radiotherapy with better safety [12,13]. Icotinib hydrochloride (IH) was first developed by Zhejiang Bata Pharma Ltd (Hangzhou, Zhejiang, China) and has been approved by the China Food and Drug Administration (CFDA) as an oral EGFR inhibitor in June 2011 [14]. As a small molecule inhibitor of EGFR, IH increased radiosensibility in several malignant tumors. Zhang et al. [15] found that pretreatment with IH reduced the survival and increased radiation-induced apoptosis of lung cancer cells. Similar results were observed in colorectal cancer and cervical cancer cells [16,17]. However, it is still unknown whether IH cause radiosensitization of NPC cells. In our previous study, IH (125 mg/day) combined with intensity-modulated radiation therapy (IMRT) showed good efficacy and safety in patients with locally advanced NPC [18]. Therefore, in this study we aimed to investigate the expression of EGFR and the correlations with the clinicopathologic features and prognosis in NPC patients, and further explore how IH enhances radiosensitization of NPC cells.
This study was approved by the Institutional Review Board Committees of Taizhou Central Hospital (Taizhou University Hospital). All the enrolled patients signed informed consent. Total 115 primary NPC paraffin-embedded specimens were collected between January 2011 and October 2017, while chronic nasopharyngitis lesions (n = 30) were collected as the control to better assess the correlation of EGFR expression with nasopharyngeal lesions. All the patients were pathologically diagnosed with immunohistochemistry, and NPC patients with distant metastasis were excluded at the time of diagnosis. According to the 7th edition of the American Joint Committee on Cancer staging system, tumor-node-metastasis (TNM) staging score was determined. All the patients received standard treatment with radiotherapy, chemotherapy, or chemoradiotherapy. Patients with other malignant disease history were excluded. The median age of 115 patients was 54 years (ranging from 30 to 82 years), and the detailed characteristics are summarized in Table 1. The average follow-up was 53.7 ± 25.1 months (range: 10.6–99 months).
2.2 Immunohistochemical Staining
Paraffin-embedded slides of 4 μm were dewaxed in xylene and rehydrated through a graded series of ethanol. Antigen retrieval was performed by boiling the slides with citrate buffer (pH = 6) at 120°C for 5 min, and peroxidase activity was blocked using a 3% hydrogen peroxide solution at room temperature for 15 min. After that, the samples were incubated with normal serum, and then with antibodies against EGFR (18986-1-AP, Proteintech Group, Wuhan, China) at 4°C overnight. After washing with 0.01 M of phosphate-buffered saline (PBS) solution, the samples were incubated with labeled goat anti-rabbit secondary antibody (SA00001-2, Proteintech Group, Wuhan, China) for 60 min at 37°C. The samples were washed, stained with diaminobenzidine solution, and then counterstained with hematoxylin. EGFR staining was semi-qualitatively assessed by two pathologists according to positive staining intensity and the percentage of stained cells. EGFR expression was graded as follows: negative; >5%–25%, (1+); >25%–50%, (2+); and >50%, (3+). Negative and 1+ grades were classified as low EGFR expression, and 2+ and 3+ grades were classified as high EGFR expression.
Human NPC cell line CNE-2 was obtained from American Type Culture Collection (Manassas, VA, USA), and maintained in Roswell Park Memorial Institute (RPMI)-1640 medium (Invitrogen Life Technologies, Carlsbad, CA, USA) supplemented with 10% fetal bovine serum (Hyclone, Logan, UT, USA) and 1% penicillin/streptomycin in a humidified incubator at 37°C. IH was purchased from Zhejiang Beta Pharma, Ltd. (Hangzhou, China) and dissolved in 100% dimethyl sulfoxide (DMSO) to a final concentration of 40 mg/ml and stored at −20°C.
2.4 Generation of Radioresistant Clone
When CNE-2 cells reached approximately 60% confluence, the cells were subjected to 2 Gy using Siemens clinical linear accelerator (Primus H, 6MV-X, dosage rate of 200 cGy/min, source skin distance 100 cm, and irradiation field of 10 * 10 centimeter). The procedures were repeated three times with a gradient model of 2, 4, 6, and 8 Gy to the total dose of 60 Gy in 12 months, and the surviving cells were cultured and named CNE-2R. Another flask of CNE-2 cells was cultured synchronously as a control cell line without radiation exposure.
CNE-2/2R cells in the exponential phase were seeded in 96-well plates at a density of 5,000 cells/well. CNE-2/2R cells were irradiated with the dose of 4 Gy. On days 1, 2, 4, and 6 after irradiation, CNE-2/2R cells were treated with 20 µl/well of 3-(4, 5-diethyl-2-thiazolyl)-2, 5-diphenyltetrazolium bromide (5 mg/ml) for 4 h at 37°C, resulting in the generation of formazan crystals. DMSO (150 µl/well) was added to dissolve the formazan and OD value was measured in a microplate reader (Safe Heart, SHE 3000) at a wavelength of 490 nm.
In addition, cells were treated by IH with different final concentrations (0.013, 0.025, 0.05, 0.1, 0.2, and 0.4 mg/ml) for 24 h, MTT assay was performed, and the inhibition rate was calculated as: (1 − OD value of experimental group/control group OD) × 100%. The IC20 (20% inhibitory concentration) was selected as the subsequent experimental concentration.
CNE-2/2R cells in the exponential phase were seeded in 6-well plates with appropriate densities (300–3,000/well), and were incubated in the medium containing IH (IC20 concentration) or DMSO for 24 h. After substituting with complete growth medium, the plates were irradiated with 0, 2, 4, 6, 8, and 10 Gy, depending on cell number, and cultured for 10 to 14 days until macroscopic colonies (>50 cells per colony) appeared. After Giemsa staining, the colonies were counted under an inverted microscope. The plating efficiency (PE) was calculated as the fraction of colonies counted divided by the numbers of cells. The survival fraction (SF) was then calculated as colonies counted/(cells seeded × PE/100). Eventually, the data were fitted to a single hit multiple targets equation, SF = 1 − [1 − exp(−D/D0)]N. The cell survival curve was obtained, and radiosensitivity parameters (D0, Dq, N, SF2) were compared.
2.7 Reverse Transcription Polymerase Chain Reaction
Total RNA was extracted from cell lysis solution by Trizol reagent (Invitrogen, Carlsbad, CA, USA). PCR was performed at 95°C for 3 min, followed by 40 cycles of 95°C for 30 s, 55°C for 30 s, and 72°C for 1 min, using Thermo Scientific™ DreamTaq™ Green PCR Master Mix (Thermo Scientific) and following primers (BioSune Biotechnology, Shanghai, China): EGFR forward primer, CGAAAGCCAACAAGGAAAT; reverse primer, CAAACAGTCACCCCGTAGC. GAPDH forward primer, GAAGGTCGGAGTCAACGGAT; reverse primer, CCTGGAAGATGGTGATGGG. The relative expression level was calculated with the 2−ΔΔCt method. GAPDH was utilized as an internal control for normalization.
Protein lysates were collected from cells, separated by 10% sodium dodecyl sulfate polyacrylamide gel electrophoresis (SDS-PAGE) and transferred to polyvinylidene difluoride membranes. After blocking with 5% bovine serum albumin for 1 h, the membranes were incubated with EGFR and GAPDH antibodies (Proteintech Group, Wuhan, China) overnight at 4°C. Following washing with tris-buffered saline with Tween (TBST) three times, the membranes were incubated with secondary antibody labeled with horseradish peroxidase. The bands were finally visualized using ECL method. GAPDH was used as loading control.
Statistical analysis was performed with PASW Statistics V18.0 software and Free Statistics software version 1.3. All quantitative data were represented as mean values ± standard deviation, and statistical differences between groups were analyzed using the t-test. The correlation between EGFR expression and clinical parameters was calculated with the Pearson’s chi-squared (χ2) test. Survival analysis was conducted according to the Kaplan-Meier method and Cox proportional hazards regression analysis. P < 0.05 was considered to be significant.
3.1 EGFR Expression in NPC Lesions and Chronic Nasopharyngitis Lesions
EGFR positive staining was observed in the membrane and cytoplasm region in both NPC (Fig. 1A) and nasopharyngitis (Fig. 1B) tissue section. Overall, EGFR expression was positive in 96 of 115 (83.5%) NPC lesions, and in 7 of 30 (23.3%) chronic nasopharyngitis lesions. According to the grade definition, high and low levels of EGFR expression were noted in 73 (63.48%) and 42 (36.52%) NPC patients, and in 5 (16.7%) and 25 (83.3%) chronic nasopharyngitis patients, respectively, with significant difference (P < 0.001).
Figure 1: Immunohistochemical staining intensity (1+, 2+, 3+) of EGFR. (A) Primary NPC lesions. (B) Chronic nasopharyngitis lesions
3.2 Association of EGFR Expression and Clinical Parameters in NPC Patients
The association between EGFR expression and clinical parameters of NPC patients was shown in Table 1. The results demonstrated that EGFR expression level in NPC lesions was significantly correlated with T stage (P < 0.001) and TNM stage (P = 0.006). No correlation was observed between EGFR expression and gender, age, tumor differentiation, and lymph node status.
The 5-year disease-free survival (DFS, defined as the time from the end of therapy to the first event of either recurrent disease or death) and overall survival (OS, defined as the time from the end of therapy to the death from any cause or the end of follow-up) rate in 115 NPC patients was 72.7% and 75.3%, respectively. Patients with high EGFR expression had a poorer DFS (62.6% and 87.1%, P = 0.049) and OS (69.2% and 87.9%, P = 0.042) than those with low expression (Table 2, Fig. 2). After adjusting for prognostic factors, univariate and multivariate Cox proportional regression analysis showed that EGFR expression (HR: 3.10; 95% confidence interval (CI) [1.02–9.44], P = 0.047) and TNM stage (HR: 3.31; 95% CI [1.06–10.31], P = 0.039) were independent prognostic factors for DFS (Table 3). Similarly, EGFR expression (HR: 3.05; 95% CI [1.23–7.59], P = 0.016), age (HR: 3.16; 95% CI [1.37–7.31], P = 0.007) and TNM stage (HR: 3.16; 95% CI [1.17–8.52], P = 0.023) were found to be independent prognostic factors for OS.
Figure 2: Kaplan-Meier survival analysis for NPC patients. (A) Comparison of disease-free survival between patients with high and low EGFR expression (P = 0.049). (B) Comparison of overall survival between patients with high and low EGFR expression (P = 0.042)
3.3 IH Enhanced Radiosensitivity of CNE-2R Cells
CNE-2R cell line was generated by graded doses of irradiation. The morphology of CNE-2/2R cells was distinguished under an inverted microscope (Figs. 3A and 3B). CNE-2 cells grew in a multilateral manner, while CNE-2R cells had a spindle or fusiform shape. CNE-2R cells exhibited an advantage in cell proliferation compared with CNE-2 cells. After radiation of 4 Gy, the OD value of CNE-2R was higher than that of CNE-2 at day 1 (0.510 ± 0.0346, 0.367 ± 0.0351, P = 0.007), day 2 (1.070 ± 0.151, 0.623 ± 0.031, P = 0.007), day 4 (1.157 ± 0.227, 0.713 ± 0.091, P = 0.035), and day 6 (1.367 ± 0.083, 1.117 ± 0.055, P = 0.036) (Fig. 3C). MTT assay showed that IC20 value of IH was 0.04 mg/ml (Fig. 3D).
Figure 3: Distinguished morphology and viability of CNE-2R cells. (A) CNE-2 cells with a multilateral manner; (B) CNE-2R cells with the shape of spindle or fusiform; (C) Cell viability at different time points was determined by MTT assay; (D) Cell viability of CNE-2 after treatment with different concentrations of IH
Clonogenic assay showed significant difference in radiosensitivity between CNE-2 and CNE-2R (Table 3 and Fig. 4A). In response to radiation, there was a dose-dependent reduction of clonogenic cell survival. The colony number of CNE-2R was significantly higher than that of CNE-2 (P < 0.05), while IH enhanced the radiosensitizing effect of CNE-2R with an apparently lower survival fraction (1.414 times, P < 0.05).
Figure 4: IH enhanced radiosensitivity of CNE-2R cells. (A) After colony formation assay, survival fraction curve was conducted for CNE-2, CNE-2R, and CNE-2R+IH groups. (B) mRNA expression level of EGFR in CNE-2, CNE-2R, and CNE-2R+IH groups. (C) Western blot analyses of EGFR in CNE-2, CNE-2R, and CNE-2R+IH groups. (D) Densitometry of EGFR protein expression in CNE-2, CNE-2R, and CNE-2R+IH groups. *P < 0.05, **P < 0.01
3.4 EGFR Expression in CNE-2/2R Cells
PCR assay indicated that the mRNA expression level of EGFR was 1.703 times higher in the CNE-2R cells than the CNE-2 cells (P = 0.001), while the expression of EGFR was significantly decreased after IH treatment (CNE-2R+IH vs. CNE-2R, P = 0.014) (Fig. 4B). Western blot assay demonstrated that EGFR protein expression was significantly elevated in CNE-2R cells compared with CNE-2 cells (P < 0.001), but a significant declination was observed after IH treatment (P = 0.004) (Figs. 4C and 4D). These results revealed that EGFR expression was higher in the radioresistant cell lines than the parental cell line and IH could downregulate EGFR expression.
In this study we reported higher expression of EGFR in NPC tissues than chronic nasopharyngitis lesions. We found that EGFR expression was an independent prognostic factor of disease-free survival and overall survival of NPC. IH enhanced the radiosensitivity of CNE-2R cells. EGFR mRNA and protein expression levels were significantly higher in CNE-2R cells compared to CNE-2 cells, but significantly decreased after IH treatment.
Although NPC is sensitive to radiotherapy, local recurrence and distant metastasis remain the leading causes of treatment failure. Enhancing the radiosensitivity with chemotherapy drugs is a conventional method for locally advanced NPC treatment [6,7]. However, severe side effects may affect and even interrupt chemoradiotherapy procedure [19]. It was reported that the modest median survival of recurrent metastatic NPC was only 11-22 months [20]. EGFR is highly expressed in a majority of human epithelial carcinomas, including NPC [21]. Up to date, a number of studies have demonstrated that EGFR inhibitors can improve the radiosensitivity in many malignant tumors [15–17,22,23]. However, few studies have focused on the radiosensitivity effect of IH in NPC, which is the aim of the study based on our previous study [18].
In this study, EGFR expression was presented in 83.5% of NPC patients, in contrast to 23.3% of chronic nasopharyngitis patients, with a significant difference. Further analysis showed that EGFR expression was significantly associated with TNM stage and T stage, but not gender, age, tumor differentiation, and lymph node status. In univariate and multivariate Cox proportional regression analysis, two parameters (EGFR expression and TNM stage) and three parameters (EGFR expression, age and TNM stage) were identified as independent prognostic factors for DFS and OS, respectively, while EGFR expression was the common factor. Zhang et al. [24] reported that EGFR expression was detected in 89.5% of NPC patients and correlated with T stage.
In addition, EGFR expression was an independent prognostic factor, three-year survival rates were lower in EGFR-positive (75.8%) patients than in EGFR-negative patients (91.2%). A meta-analysis demonstrated that the pooled HRs for both OS and DFS were higher than 2, indicating prognostic value of EGFR [25]. Another meta-analysis including 22 studies reported similar results [10].
Radioresistant cell lines can be established via cumulative exposure to irradiation. In our study, the radioresistant cell line CNE-2R was successfully obtained using a gradient irradiation schedule with accumulated doses of 60 Gy. Compared with CNE-2, CNE-2R cells had stronger ability of proliferation and colony formation, indicating higher radioresistance, and we observed higher EGFR expression in CNE-2R cells than CNE-2 cells. When CNE-2R was exposed to IH plus irradiation, EGFR expression and colony-forming ability were reduced. Notably, the expression of EGFR was decreased sequentially in CNE-2R, CNE-2R+IH and CNE-2 cells, but the relationship between survival fraction and EGFR expression did not seem to correspond completely, perhaps due to other factors that affect the survival of NPC cells. Nevertheless, these results demonstrated that IH improved the radiosensitivity of CNE-2R. Ma et al. [16] showed that IH effectively sensitized colorectal cancer cells to radiation. Wang et al. [17] reported that IH could significantly inhibit cell proliferation, redistribute cell cycle, enhance apoptosis and impair DNA damage response of Hela S3 cells following radiation.
While IMRT is commonly used for the treatment of NPC at non-metastatic stage, NPC at advanced stages should be managed using combined radio-chemotherapy regimen. In this study we only evaluated the effects of IH on radioresistant NPC cells. This is a limitation of this study. Further studies are needed to investigate the potential of IH on chemoresistant NPC cells to promote the application of IH on combined radio- and chemotherapy for advanced NPC. Currently, very few studies reported clinical application of IH for NPC. One phase I study showed the safety of IH in combination with IMRT in patients with NPC [18]. This provides the base for further clinical studies to compare the efficacy and safety of IH and commonly used EGFR inhibitors such as nimotuzumab to improve radio-chemotherapy of locally advanced NPC. Another limitation is that we only showed the association of decreased EGFR expression and increased radiosensitivity in NPC cells after treatment with IH. Further studies are necessary to explore the mechanisms by which IH promotes radiosensitivity of NPC cells. We should consider both genetic and epigenetic mechanisms that may contribute to the regulation of EGFR expression by IH [26,27].
In summary, high EGFR expression is a poor prognostic factor for NPC patients. CNE-2R cells obtained by gradient irradiation are distinguished from parental CNE-2 cells in viability, radiosensitivity, and EGFR expression. IH is capable of enhancing the radiosensitivity of CNE-2R cells and reducing EGFR expression. These findings may provide support for clinical application of IH radiosensitization in NPC radiotherapy.
Authors’ Contributions: Bo Wu and Hua-cai Xiong contributed equally to this work. Bo Wu, Hua-cai Xiong, Yong Wang, Shan-kun Zhao, Hong-sheng Lu, and Wei Hu collected and analyzed the data. All authors read and approved the final manuscript.
Ethics Approval and Informed Consent Statement: The present study was approved by the Local Institutional Review Boards of Taizhou Central Hospital (Taizhou University Hospital) (No. 2019-SC-019). All patients provided informed consent.
Availability of Data and Materials: The data in the present study are available from the corresponding author on reasonable request.
Funding Statement: This work was funded by the Medical and Health Science and Technology Program of Zhejiang Province (2020RC040).
Conflicts of Interest: The authors declare that they have no conflicts of interest to report regarding the present study.
References
1. Sung, H., Ferlay, J., Siegel, R. L., Laversanne, M., Soerjomataram, I. et al. (2021). Global cancer statistics 2020: GLOBOCAN estimates of incidence and mortality worldwide for 36 cancers in 185 countries. CA: A Cancer Journal for Clinicians, 71(3), 209–249. DOI 10.3322/caac.21660. [Google Scholar] [CrossRef]
2. Chen, Y. P., Chan, A. T. C., Le, Q. T., Blanchard, P., Sun, Y. et al. (2019). Nasopharyngeal carcinoma. Lancet, 394(10192), 64–80. DOI 10.1016/S0140-6736(19)30956-0. [Google Scholar] [CrossRef]
3. Kang, Y., He, W., Ren, C., Qiao, J., Guo, Q. et al. (2020). Advances in targeted therapy mainly based on signal pathways for nasopharyngeal carcinoma. Signal Transduction and Targeted Therapy, 5(1), 245. DOI 10.1038/s41392-020-00340-2. [Google Scholar] [CrossRef]
4. Chen, L., Hu, C. S., Chen, X. Z., Hu, G. Q., Cheng, Z. B. et al. (2017). Adjuvant chemotherapy in patients with locoregionally advanced nasopharyngeal carcinoma: Long-term results of a phase 3 multicentre randomised controlled trial. European Journal of Cancer, 75, 150–158. DOI 10.1016/j.ejca.2017.01.002. [Google Scholar] [CrossRef]
5. Lee, A. W. M., Tung, S. Y., Ng, W. T., Lee, V., Ngan, R. K. C. et al. (2017). A multicenter, phase 3, randomized trial of concurrent chemoradiotherapy plus adjuvant chemotherapy versus radiotherapy alone in patients with regionally advanced nasopharyngeal carcinoma: 10-year outcomes for efficacy and toxicity. Cancer, 123(21), 4147–4157. DOI 10.1002/cncr.30850. [Google Scholar] [CrossRef]
6. Yu, Y., Liang, H., Lv, X., Ke, L., Qiu, W. et al. (2018). Platinum-based concurrent chemotherapy remains the optimal regimen for nasopharyngeal carcinoma: A large institutional-based cohort study from an endemic area. Journal of Cancer Research and Clinical Oncology, 144(11), 2231–2243. [Google Scholar]
7. Hong, R. L., Hsiao, C. F., Ting, L. L., Ko, J. Y., Wang, C. W. et al. (2018). Final results of a randomized phase III trial of induction chemotherapy followed by concurrent chemoradiotherapy versus concurrent chemoradiotherapy alone in patients with stage IVA and IVB nasopharyngeal carcinoma-Taiwan Cooperative Oncology Group (TCOG) 1303 study. Annals of Oncology, 29(9), 1972–21979. [Google Scholar]
8. Sun, Y., Li, W. F., Chen, N. Y., Zhang, N., Hu, G. Q. et al. (2016). Induction chemotherapy plus concurrent chemoradiotherapy vs. concurrent chemoradiotherapy alone in locoregionally advanced nasopharyngeal carcinoma: A phase 3, multicentre, randomised controlled trial. The Lancet Oncology, 17(11), 1509–1520. [Google Scholar]
9. London, M., Gallo, E. (2020). Epidermal growth factor receptor (EGFR) involvement in epithelial-derived cancers and its current antibody-based immunotherapies. Cell Biology International, 44(6), 1267–1282. [Google Scholar]
10. Ooft, M. L., Braunius, W. W., Heus, P., Stegeman, I., van Diest, P. J. et al. (2015). Prognostic significance of the EGFR pathway in nasopharyngeal carcinoma: A systematic review and meta-analysis. Biomarkers in Medicine, 9(10), 997–1010. [Google Scholar]
11. Mitchell, R., Luwor, R., Burgess, A. (2018). Epidermal growth factor receptor: Structure-function informing the design of anticancer therapeutics. Experimental Cell Research, 371(1), 1–19. DOI 10.1016/j.yexcr.2018.08.009. [Google Scholar] [CrossRef]
12. Huang, J., Yuan, X., Pang, Q., Zhang, H., Yu, J. et al. (2018). Radiosensitivity enhancement by combined treatment of nimotuzumab and celecoxib on nasopharyngeal carcinoma cells. Drug Design, Development and Therapy, 12, 2223–2231. DOI 10.2147/DDDT. [Google Scholar] [CrossRef]
13. You, R., Sun, R., Hua, Y. J., Li, C. F., Li, J. B. et al. (2017). Cetuximab or nimotuzumab plus intensity-modulated radiotherapy versus cisplatin plus intensity-modulated radiotherapy for stage II-IVb nasopharyngeal carcinoma. International Journal of Cancer, 141(6), 1265–1276. DOI 10.1002/ijc.30819. [Google Scholar] [CrossRef]
14. Huang, H., Miao, H., Wang, J., Wu, D., Lei, Q. et al. (2021). Advances on anticancer new drugs in China and the USA in 2020: From ongoing trial to drug approval. Journal of the National Cancer Center, 1(4), 147–152. DOI 10.1016/j.jncc.2021.08.002. [Google Scholar] [CrossRef]
15. Zhang, S., Fu, Y., Wang, D., Wang, J. (2018). Icotinib enhances lung cancer cell radiosensitivity in vitro and in vivo by inhibiting MAPK/ERK and AKT activation. Clinical and Experimental Pharmacology and Physiology, 45(9), 969–977. DOI 10.1111/1440-1681.12966. [Google Scholar] [CrossRef]
16. Ma, H., Bi, J., Liu, T., Ke, Y., Zhang, S. et al. (2015). Icotinib hydrochloride enhances the effect of radiotherapy by affecting DNA repair in colorectal cancer cells. Oncology Reports, 33(3), 1161–1170. DOI 10.3892/or.2014.3699. [Google Scholar] [CrossRef]
17. Wang, X., Gu, Y., Liu, H., Shi, L., Sun, X. (2018). Icotinib hydrochloride enhances chemo- and radiosensitivity by inhibiting EGFR signaling and attenuating RAD51 expression and function in Hela S3 cells. OncoTargets and Therapy, 11, 1245–1258. DOI 10.2147/OTT. [Google Scholar] [CrossRef]
18. Hu, W., Wang, W., Yang, P., Zhou, C., Yang, W. et al. (2015). Phase I study of icotinib, an EGFR tyrosine kinase inhibitor combined with IMRT in nasopharyngeal carcinoma. International Journal of Clinical and Experimental Medicine, 8(9), 15675–15683. [Google Scholar]
19. Chen, Y., Tang, Z., Yu, M., Zhang, R., Dong, X. et al. (2020). Molecular biomarkers: Multiple roles in radiotherapy. BIOCELL, 44(4), 513–524. DOI 10.32604/biocell.2020.09422. [Google Scholar] [CrossRef]
20. Zhao, C., Miao, J., Shen, G., Li, J., Shi, M. et al. (2019). Anti-epidermal growth factor receptor (EGFR) monoclonal antibody combined with cisplatin and 5-fluorouracil in patients with metastatic nasopharyngeal carcinoma after radical radiotherapy: A multicentre, open-label, phase II clinical trial. Annals of Oncology, 30(4), 637–643. DOI 10.1093/annonc/mdz020. [Google Scholar] [CrossRef]
21. Kontani, K., Kuraishi, K., Hashimoto, S., Norimura, S., Hashimoto, N. et al. (2021). Prolonged survival in patients with human epidermal growth factor receptor-2-overexpressed metastatic breast cancer after targeted therapy is dominantly contributed by luminal-human epidermal growth factor receptor-2 population. Oncologie, 23(2), 229–239. DOI 10.32604/Oncologie.2021.016277. [Google Scholar] [CrossRef]
22. Marref, I., Reichling, C., Vendrely, V., Mouillot, T. (2018). Management of anal canal cancer in 2018. Oncologie, 20(5–6), 94–106. DOI 10.3166/onco-2018-0015. [Google Scholar] [CrossRef]
23. Zhang, D., Liu, H., Yi, Z., Lu, Y., Chen, Y. et al. (2021). Thymidylate synthase confers pemetrexed resistance of non-small cell lung cancer cells by EGFR/PI3K/AKT pathway. BIOCELL, 45(3), 617–625. DOI 10.32604/biocell.2021.012504. [Google Scholar] [CrossRef]
24. Zhang, P., Wu, S. K., Wang, Y., Fan, Z. X., Li, C. R. et al. (2015). MDM2, eIF4E and EGFR expression in nasopharyngeal carcinoma and their correlation with clinicopathological characteristics and prognosis: A retrospective study. Oncology Letters, 9(1), 113–118. DOI 10.3892/ol.2014.2631. [Google Scholar] [CrossRef]
25. Ma, X., Huang, J., Wu, X., Li, X., Zhang, J. et al. (2014). Epidermal growth factor receptor could play a prognostic role to predict the outcome of nasopharyngeal carcinoma: A meta-analysis. Cancer Biomarkers, 14(4), 267–277. DOI 10.3233/CBM-140401. [Google Scholar] [CrossRef]
26. Chen, X., Zhang, H., Li, L., Chen, W., Bao, T. et al. (2021). miR-5100 mediates migration and invasion of melanomatous cells in vitro via targeting SPINK5. Journal of Comprehensive Molecular Science and Genetics, 1(1), 14–23. [Google Scholar]
27. Wang, Z., Wu, R., Nie, Q., Bouchonville, K. J., Diasio, R. B. et al. (2021). Chromatin assembly factor 1 suppresses epigenetic reprogramming toward adaptive drug resistance. Journal of the National Cancer Center, 1(1), 15–22. DOI 10.1016/j.jncc.2020.12.003. [Google Scholar] [CrossRef]
Cite This Article
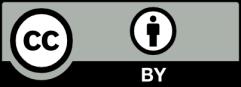
This work is licensed under a Creative Commons Attribution 4.0 International License , which permits unrestricted use, distribution, and reproduction in any medium, provided the original work is properly cited.