Open Access
ARTICLE
Effect of a Double Helical Spring Decompression Structure Backpack on the Lumbar Spine Biomechanics of School-Age Children: A Finite Element Study
1 Faculty of Sports Science, Ningbo University, Ningbo, 315211, China
2 Doctoral School on Safety and Security Science, Óbuda University, Budapest, 1034, Hungary
3 Faculty of Engineering, University of Szeged, Szeged, 6724, Hungary
4 Anta Sports Sciences Laboratory, Anta Group, Xiamen, 362212, China
* Corresponding Authors: Dong Sun. Email: ; Yaodong Gu. Email:
Molecular & Cellular Biomechanics 2023, 20(1), 35-47. https://doi.org/10.32604/mcb.2023.041016
Received 07 April 2023; Accepted 23 May 2023; Issue published 20 June 2023
Abstract
Background: A children’s backpack is one of the important school supplies for school-age children. Long-term excessive weight can cause spinal deformity that cannot be reversed. This study compared a double helical spring decompression structure backpack (DHSB) with a traditional backpack (TB) to explore the optimization of decompression devices on upper body pressure. The finite element (FE) method was then used to explore the simulation of lumbar stress with different backpacks, in order to prove that DHSB can reduce the influence of backpack weight on lumbar vertebrae, avoid the occurrence of muscle discomfort and spinal deformity in children; Methods: 18 male children subjects (age: 12.5 ± 0.6 years; height: 145.5 ± 1.9 cm; bodyweight: 40.8 ± 3.1 kg) ran with DHSB and TB at a speed of 3.3 ± 0.2 m/s. Flexible pressure sensors were used to measure the pressure on the shoulder, back, and waist during running. The pressure data was then inputted into the FE model to simulate the effect of carrying different backpacks on the stress of the lumbar intervertebral disc (IVD); Result: There was a significant difference in shoulder and waist peak pressure between the DHSB and TB during the running posture. At a speed of 3.3 ± 0.2 m/s, the peak pressure of the shoulder and waist decreased. After finite element analysis, it was found that carrying DHSB on the back could effectively reduce the intervertebral disc pressure between L4-5 and L5-S1 by 27.9% and 34.1%, respectively; Conclusion: DHSB can effectively reduce the pressure on the shoulder and waist when children are running and can reduce the influence of backpacks on children’s posture to a certain extent. By finite element analysis, it is found that carrying DHSB can effectively reduce the stress of the lumbar intervertebral disc, and the damage to lumbar vertebrae is lower than with a TB.Graphic Abstract
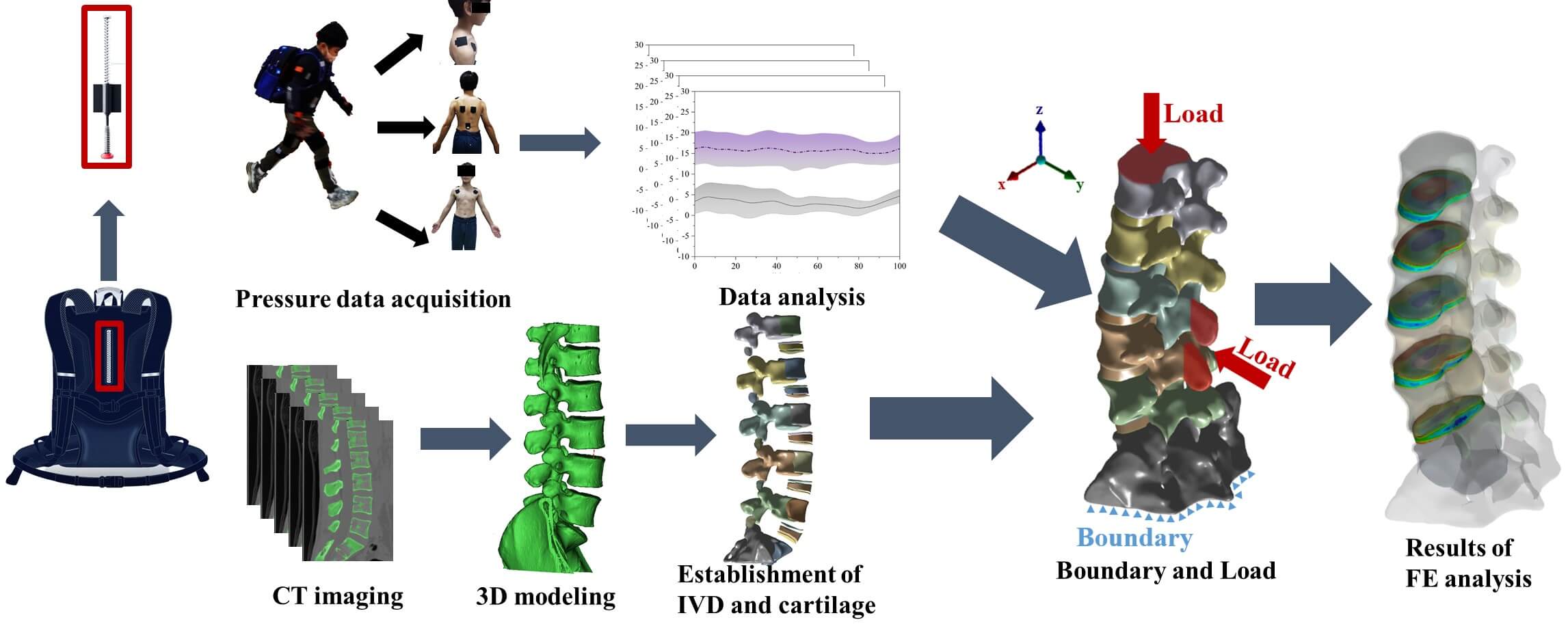
Keywords
With the number of adolescents suffering from scoliosis, hunchback, and musculoskeletal disorders increasing every year, schools and families are paying more attention to the health of adolescents. Backpacks are the most suitable tool for carrying everyday school equipment because they can distribute the load symmetrically [1]. However, carrying a heavy backpack can lead to a variety of pain associated with musculoskeletal disorders and postural dysfunction [2]. Whittfield et al. [3] found that stress from carrying a heavy backpack is one of the most important factors contributing to skeletal muscle pain. Prolonged weight bearing can be harmful to the human spine, lumbar region, and body balance [4]. School-age children are susceptible to musculoskeletal effects, so carrying an excessively heavy backpack can lead to serious physical injuries. The results of Makcie et al. [5] indicated that the weight of the backpack has the greatest effect on shoulder stress and pressure, based on their study of four different school backpacks.
The weight of the backpack is crucial for the growth of the adolescent spine. Research showed that when the backpack weight increases to 15% of the body weight [6], adolescents have difficulty maintaining normal body posture, which can result in loss of balance and pain in the shoulders, back, and lumbar region [7,8]. Therefore, the weight of the backpack can directly or indirectly affect the spinal health and growth and development of adolescents. To better understand the relationship between adolescent weight bearing and body balance, spinal curvature, and health problems such as low back pain, further research is needed. Grimmer et al. [9] suggested that there is a significant difference in the absolute difference in an atlantooccipital joint between adolescents carrying and not carrying backpacks, indicating that backpacks affect the spine of children. There is also a large body of research on backpack weight limits for adolescents. Chansirinukor et al. [10] found that the forward tilt angle of the trunk increased when adolescents carried a backpack weighing 15% of their body weight. The greater angle is used to stabilize the center of gravity and maintain body balance [11,12]. It is shown that the student changes the position of the center of gravity by leaning forward to counteract the backward twisting force caused by the weight of the backpack and to maintain balance in their body. Goh et al. [13] collected data on the lumbosacral joint in adolescents walking with a backpack weighing 15% and 30% of their body weight. They found that carrying a backpack weighing 15% and 30% of body weight increased the strength of the lumbosacral joints by 26.7% and 64.0%, respectively, compared to walking without a backpack. This increase in strength may lead to back pain and spinal injury.
There have been numerous studies focused on designing backpacks for weight reduction. For example, Cottalorda et al. [14] found that the shape of the backpack can effectively distribute weight and improve overall comfort. Amiri et al. [15] used a user-centered design approach combined with normal standards and ergonomic features to create a backpack that significantly reduces payload on the shoulders and neck. Putra et al. [16] from Bandung Institute of Technology, Indonesia, designed a suspended backpack to allow relative motion between the backpack and the back, minimizing the impact caused by the up- and-down movement of the backpack when walking or running. However, these designs typically use traditional lumbar straps to transfer gravity, without considering the impact of backpacks on human balance and low back pain.
The current measurement techniques for the lumbar spine are limited by ethical constraints and the complexity of human structures. Non-invasive or minimally invasive modalities such as imaging measurements, morphological measurements, myoelectric recordings, and ultrasound measurements are used to measure the response of individual structures to physical shape, but they cannot accurately and quantitatively describe the forces on the lumbar spine. With the advancements in biomechanics and computer software and hardware, FE technology can provide a comprehensive and accurate representation of the changes in the spine in response to forces, including the biomechanical changes of every detail. FE technology overcomes the limitations of human body measurement and provides reliable guidance for clinical research, surgical planning, prognosis prediction, and development of implant materials. Therefore, it has been increasingly used in the field of spinal surgery research. Assuming that the DHSB backpack can optimize the pressure on the upper body, reduce the pressure on the shoulders and waist of children during walking and running, minimize the impact of the backpack on their posture, and alleviate stress on the intervertebral discs. SO, this study employs the FE method to investigate the effect of carrying a double helical spring decompression structure backpack (DHSB) on the biomechanics of the lumbar spine and to provide guidance and suggestions for students’ daily backpack selection from a biomechanical perspective.
Research on backpacks that protect the spine of adolescents has focused on the effects of the backpack on the lumbar region of the adolescent spine, as well as on the design of the backpack from a force-saving perspective, but few studies have combined the two. This study is novel in that it compares a new backpack design, the Double Helical Spring Decompression Structure Backpack (DHSB), with a traditional backpack (TB) in terms of reducing pressure on children’s shoulders and waist. Additionally, the study uses the finite element analysis method to explore the effect of different backpacks on lumbar intervertebral disc stress. The design of backpacks is crucial for adolescent spinal health, as an unreasonable design can result in shoulder and low back pain [7]. In response to these issues, this paper aims to measure the effectiveness of a weight reduction backpack that can reduce the load on the shoulders and waist and accurately transfer the weight of the backpack to the lower extremities, thereby reducing pain in the lower back and protecting the health of the spine.
18 school-age students were recruited in this study, without scoliosis and lumbar spine-related diseases. Using Gpower [17] to calculate the required sample size for this experiment. Inform the whole experimental procedure in detail and sign the informed agreement. The results of the descriptive analysis of the data on the basic conditions of age, height, and body mass of the subjects were: age (12.5 ± 0.6) years; height (145.5 ± 1.9) cm; and body mass (40.8 ± 3.1) kg.
2.2 Double Helical Spring Decompression Structure Backpack (DHSB)
Fig. 1 shows a picture of the backpack weight reduction system. The backpack shoulder straps are connected to the back panel through an elastic material connecting rod. The distance between the backpack and the body’s main axis is reduced, and the weight of the backpack is precisely transferred to the lower extremities through the hip belt. When adolescents walk with a DHSB, the DHSB sways up and down with the body’s gait. The elastic rod will store elastic potential energy with the downward movement of the DHSB and release elastic potential energy upward, thus reducing the downward impact of the backpack on the body. At the same time, the elastic potential energy stored in the elastic rod transfers the weight to the lower limbs of the body through the spring device of the hip belt, thus achieving the purpose of weight reduction.
Figure 1: Schematic diagram of double helical spring decompression structure backpack (DHSB)
2.3 Pressure Test of Shoulder and Waist
Flexible pressure sensors (China) were placed on each side of the subject’s shoulder, waist, and back at a frequency of 1200 HZ. Due to the need to measure pressure data in the posterior lumbar spine, a flexible pressure transducer was placed in the middle part of the subject’s lumbar spine (L3–L4 lumbar spinous process), as shown schematically in Fig. 2. Subjects performed running movements while carrying a backpack with and without a double helical spring decompression structure. The weight of the backpack is 15% of the body weight. Subjects performed a 10 min warm-up run first and adapted to the experimental environment. Subjects were then asked to perform the experimental test at a speed of 3.3 ± 0.2 m/s [17], running speed was recorded using a Brower timing device (Beower Timing System, Draper, UT, USA), and the subjects were considered to have a successful acquisition with a natural running posture and an error of 5% or less from the set speed. Each subject collected no less than eight successful data, with a running distance of 100 m. After each 100 m running, the subjects rested for 10 min. The peak pressure data of the shoulder, back, and lumbar region were recorded during each running cycle.
Figure 2: Distribution of flexible pressure sensors
Data were selected from a 12-year-old boy weighing 45 kg. The imaging data of the lumbar spine curved segments from L1 to S1. The patient had no history of surgery in the relevant area, no congenital scoliosis or neurogenic scoliosis, and no osteotomy was performed. Two-dimensional CT images of 785 spines were obtained using Somatom Sensation16 spiral CT (Siemens, Munich, Germany) with transverse scans on lumbar segments from T11 to S1 under 0.75 mm slices. All CT images were saved in DICOM format. This study was done with the knowledge of the subject and was supported by the Institutional Ethics Committee of Ningbo University (No. RAGH2022022300316).
Conversion of imaging 2D images into 3D models using Mimics 20.0 (Materialise, Leuven, Belgium). Uneven surfaces were processed using Geomagic Studio 2013 (Geomagic, Inc., Research Triangle Park, NC, USA). Each part was then imported separately into SolidWorks 2020 (SolidWorks Corporation, Massachusetts, USA) to form solid parts. Based on the anatomical features of the lumbar spine, the annulus fibrosus, nucleus pulposus, end plate, and articular cartilage were created in SolidWorks 2020, as shown in Fig. 3. In Ansys Workbench 19.0 (ANSYS, Inc., Canonsburg, USA), the model is meshed and given the boundary load, and the FE analysis is carried out.
Figure 3: Experimental flow and FA modeling
Model validation is an important part of FE, and previous studies have described FE models of the normal lumbar spine [18]. According to the physiological anatomical model of the lumbar spine, the nucleus pulposus of the intervertebral disc (IVD) and the annulus fibrosus not separate under load, so the contact between them is set to “Bonded”. The vertebral body and intervertebral joints slide under a certain load, so the contact condition between the vertebral body and the intervertebral joints is set to “Frictional”. The coefficient of friction was determined to be 0.01 in this study due to the presence of articular synovial fluid [19]. All components were defined as elastomers, and bone structures and IVDs were simulated as isotropic elastic materials. The material properties of bone, IVD, and ligaments were determined from experimental literature, and the material parameters of bone and soft tissues are shown in Table 1 [20]. Different materials are distinguished according to their modulus of elasticity and Poisson’s ratio.
Under physiological conditions, 2/3 of the total weight of the lumbar spine and the DHSB was axially loaded with 345 N (the patient’s weight was 450 N, and the weight of the backpack was converted to 67.5 N) [21]. Boundary constraints were applied to the displacement and rotation of all nodes at the base of the S1 vertebral body in all directions. Based on the lumbar pressure values of 7.84 and 11.86 N measured by the flexible pressure transducer, the pressure was applied to the L3 and L4 spines for ease of calculation, as shown in Fig. 3.
A finite element study on the lumbar spine necessitates various simplifications and assumptions that can significantly impact the results. As such, experimental validation of the model is necessary. Rohlmann et al. [22] used a spine tester [23] to apply pure moments of 3.75 Nm in the three main planes, which simulate flexion/extension, left and right lateral bending, and left and right axial rotation, to load lumbar spines.
In the study, the L5 vertebra was immobilized, and the moments were applied to the L1 vertebra. After implanting a telemetered internal spinal fixation device, the measurements were repeated. The data from this experiment was compared with that from a non-linear finite element (FE) study conducted under the same loading conditions, as well as with in vivo experiments and lumbar spine FE data reported by Zander et al. [24], to validate the model.
We developed a finite element model of the lumbar spine based on CT scans of healthy patients in this study. Material properties were assigned to the ligaments, bones, and other components of the model, in order to simplify the calculation of the whole model mesh, the tetrahedron is used to divide the mesh, the mesh size of articular cartilage and endplate is 1 mm mesh, and the mesh size of other parts is 1.5 mm. In addition, 693123 cells and 1030258 nodes are implemented. Convergence analysis is performed by resetting element size control until the estimation error is less than 4% [25]. Once the model was validated, we established lumbar models. Using the finite element method, we analyzed the effect of backpack weight on the lumbar spine.
3.1 Pressure Distribution of the Backpack
The study’s results regarding the pressure values of different parts of the two backpacks are presented in Table 2 which revealed statistical differences in the peak pressure of the shoulder and waist during running stance phases between DHSB and TB. The peak pressure of the shoulder decreased significantly (p = 0.046) under the DHSB condition, while the peak pressure of the waist also decreased significantly (p = 0.026). The DHSB had 45.34%, 46.75%, and 7.91% of the pressure accounted for by the shoulder, back, and waist, respectively. In contrast, the TB had 49.94%, 40.32%, and 9.73% of the stress in the shoulder, back, and lumbar region, respectively.
The study recorded the range of motion (ROM) of the lumbar spine at L1-L5 under six pure torque conditions of 3.5NM. The results were compared with in vitro experimental data reported by Rohlmann et al. [22] and FE simulation data by Zander et al. [24]. The ROM obtained by the FE model in this study is generally consistent with the average value of the in vitro experiments, as evidenced by its standard deviation, and exhibits a similar changing trend.
After model validation, the model is valid, and the established model includes 11053681 C3D4 solid units, 1923894 nodes of the 3D FE model, and a complete 3D FE model of the L1-S1 vertebral body is established. The FE model includes fine reconstructions of the various vertebral bodies, IVD, upper and lower articular cartilage, and all ligaments and other anatomical structures, and the model is shown in Fig. 4.
Figure 4: Established FE model
Applied the lumbar pressure measured in the experiment to the FE model, the pressure on the lumbar spine from the DHSB and the TB was 7.84 and 11.86 N, respectively, applied to L3 and L4 from the posterior aspect of the spinous process of the lumbar spine. The FE stress distribution diagram shows that the stresses are mainly concentrated in the front and rear of the IVD fiber ring. DHSB has little effect on IVD pressure between L12 and L23. Fig. 5 shows that the DHSB can effectively reduce the pressure of the backpack on the lumbar spine. The IVD stress between L3-S1 was reduced by 10.7%, 27.9%, and 34.1%, respectively. DHSB can effectively reduce the pressure on the IVD.
Figure 5: Stress distribution diagram and maximum stress value of IVD
The effects of backpacks on children’s body posture have been widely studied, with previous research mainly focusing on the relationship between backpacks and musculoskeletal discomfort [8,26,27], changes in body posture [10,11,28–30], and balance ability [31]. Various countermeasures have been proposed, including limiting backpack weight [31,32] and adjusting the center height of the backpack [33,34]. However, the aim of this study was to compare the effects of a DHSB with a TB on reducing pressure on children’s shoulders and waist, as well as on lumbar intervertebral disc stress. Our results showed that carrying DHSB can effectively reduce the pressure on children’s shoulder and waist when running, and can also reduce the influence of backpacks on children’s posture. Furthermore, our finite element analysis revealed that DHSB can effectively reduce the stress on the lumbar intervertebral disc and cause less damage to the lumbar spine compared to TB.
The results of this study showed that the difference in pressure between the DHSB and the TB was statistically significant, implying that the pressurized structure of the DHSB can effectively reduce the pressure on the shoulders and lumbar region. The unique decompression design of DHSB makes the backpack sway up and down with the body’s gait when the youth is running with the backpack, that is, the elastic rod will store elastic potential energy with the downward movement of the backpack and release elastic potential energy upward, thus reducing the downward impact of the backpack on the body. At the same time, the elastic potential energy is stored in the elastic rod and transferred to the body’s lower limbs through the spring device at the hip belt, thus achieving the purpose of weight reduction. It was found that overweight backpacks and other external forces may affect the formation of good body posture in children [35]. Dianat et al. [29] studied the relationship between children’s backpack weight and musculoskeletal discomforts and showed that children’s age was negatively correlated with the probability of musculoskeletal discomforts symptoms, and the probability of symptoms was negatively correlated, especially in children aged 11–14 years old, whose spine is at a critical stage of adolescent development, are more prone to adverse changes in body posture due to faulty backpacks. This study did not investigate the effect of pack weight on posture. Chansirinukor et al. [10] found significant differences in Vanio-vertebral angle and sagittal shoulder posture angle in standing conditions without weight bearing and with 15% body weight bearing compared to each other. The angle of Vranio-vertebral Angle and sagittal shoulder posture angle were significantly reduced in children with 15% body weight compared to the unload. Ramprasad et al. [36] compared the changes in body posture of prepubertal children in different weight-bearing standing states (0% body weight, 5% body weight, 10% body weight, 15% body weight, 20% body weight, 25% body weight) and found that all body posture indicators changed with increasing weight and that significant changes in TA and lower limb angle occurred in adolescents when weight bearing reached 5% body weight. Significant changes in Vranio-vertebral angle were observed in adolescents when weight bearing reached 15% of body weight; the Vranio-vertebral angle decreased from 55.11° (0% body weight) to 51.49° (25% body weight) [37]. In a study by Mosaad et al. [38] showed significant changes in body posture in children around 10 weeks of age with a load of 7.5% of body weight.
As the weight of the backpack increases, the gravitational force on the body also increases. When the weight increases to a certain level, if the pulling force of the backpack belt is not enough, the body will lean back or fall back, and then the body will adjust its posture and increase the back support to maintain balance [2]. The increased weight of the backpack, to a certain extent, limits the mobility of the neck, head, and spine, leaving the muscles in a state of long-term stretching, which can easily lead to muscle and joint damage. Meanwhile, the weight of the backpack was mainly concentrated on the shoulders, and studies on the relationship between children’s age and musculoskeletal discomforts showed a negative correlation between children’s age and the probability of symptoms, with an increased risk of muscle damage [39]. Compared to a low pack-centered position, the high pack-centered position allowed for greater mobility of the erector spine and trapezius muscles in the subjects [40]. According to the principle of conservation of energy, the pulling force of the backpack belt is equal to the combined force of the gravity of the backpack and the back thrust. The smaller the angle between the center of gravity at the point of force of the shoulder joint and the torso, the smaller the back support force [41]. When the backpack’s gravity is constant, a smaller back support force leads to less tension on the backpack belt. It has also been suggested that L3 is closer to the body’s center of gravity than T7, which facilitates the regulation of the body’s self-center of gravity [28]. Using a high carrying way can lead to spinal curvature, anterior pelvic tilt, and limitations in the ability of muscles to work. The addition of fixed straps cannot only reduce the shaking of the backpack but also effectively alleviate the changes in body posture, which means that the fixed straps, especially the waist belt, bear a certain weight of the backpack [42]. In this study, we investigated the effect of DHSB on disc stress with the finite element method and found that the backpack reduced the lumbar stress and could effectively reduce the pressure of IVD, providing some protective effect on the discs [43].
This study discovered that carrying an overweight backpack (more than 10% of body weight) can lead to poor posture in children, resulting in forward tilting of the trunk and neck, back pain, and increased risk of falling [44,45]. On the other hand, this study shows that carrying a backpack designed with a DHSB can optimize the pressure on the shoulders and waist. Additionally, by using finite element analysis, it was found that carrying a DHSB backpack can also reduce stress on the intervertebral discs of the lumbar spine, as shown in Fig. 5. Therefore, carrying a DHSB backpack not only helps optimize the distribution of weight and pressure on the shoulders and back, thus reducing the negative effects of carrying a backpack, but it also helps protect the lumbar spine.
There are some limitations in this study. The single-subject approach is a commonly used study design in finite element analysis. However, one of its limitations is that it cannot fully capture the influence of variability in subject geometry. This is because finite element models are based on a single subject’s anatomy, and may not accurately represent the anatomical variations that exist between different individuals. It is important to acknowledge this limitation when interpreting results from finite element analysis studies and to consider its implications for clinical applications. Further research is needed to develop more robust modeling techniques that can account for individual differences in subject anatomy and better capture the complexities of the human body. The tested flexible pressure sensors did not cover the whole pressure of the shoulder, waist, and back. At the same time, the established finite element model did not take into account the influence of muscles on the spine [46], and the material properties used were all linear material properties, so the finite element material data and muscle simulation should be further improved to improve the accuracy of the finite element simulation [47]. Also, the studies in question have focused exclusively on isolated factors, with little attention paid to exploring potential interactions among multiple factors.
DHSB can effectively reduce the pressure on children’s shoulders and waist when running and reduce the impact of backpacks on children’s posture compared to a TB. Finite element analysis revealed that carrying a DHSB backpack could effectively reduce the stress on the lumbar disc and cause less damage to the lumbar spine than a TB. The findings of this study can be useful for future research in backpack design, particularly in the development of decompression devices to reduce the pressure on the upper body and lumbar spine. Moreover, the study can inform clinical practice by highlighting the potential benefits of using DHSB to reduce the risk of spinal deformity and musculoskeletal discomfort in school-age children. The study’s findings can also be useful in educating parents, teachers, and school administrators about the importance of selecting appropriate backpacks for children to promote healthy spinal development.
Funding Statement: This study was sponsored by Zhejiang Key Research and Development Program (Grant Number: 2021C03130), Zhejiang Province Science Fund for Distinguished Young Scholars (Grant Number: LR22A020002), Ningbo Key Research and Development Program (Grant Number: 20222ZDYF020016), Public Welfare Science and Technology Project of Ningbo, China (Grant Number: 2021S134), Ningbo Natural Science Foundation (Grant Numbers: 2022J065, 2022J120) and K. C. Wong Magna Fund in Ningbo University.
Author Contributions: All the authors participated in the creation of this manuscript. F.L., Q.Z., and D.S. were responsible for recruiting subjects; F.L., Q.Z. were responsible for modeling and finite element analysis; F.L., H.C., and I.B. were responsible for data processing; F.L. and Q.Z. were responsible for manuscript writing; D.S. and Y.G. were responsible for manuscript revision. All authors read, provided feedback, and approved the submitted version. All authors have read and agreed to the published version of the manuscript.
Availability of Data and Materials: The data used to support the findings of this study are included in the article. Please contact the relevant author if you would like to acquire the numerical dataset used to conduct the research described in the paper.
Conflicts of Interest: The authors declare that they have no conflicts of interest to report regarding the present study.
References
1. Hong, Y., Lau, T., Li, J. (2003). Effects of loads and carrying methods of school bags on movement kinematics of children during chair walking. Research in Sports Medicine, 11(1), 33–49. https://doi.org/10.1080/0308348 [Google Scholar] [CrossRef]
2. Ramprasad, M., Alias, J., Raghuveer, A. K. (2010). Effect of backpack weight on postural angles in preadolescent children. Indian Pediatrics, 47(7), 575–580. https://doi.org/10.1007/s13312-010-0130-2 [Google Scholar] [PubMed] [CrossRef]
3. Whittfield, J., Legg, S. J., Hedderley, D. I. (2005). Schoolbag weight and musculoskeletal symptoms in New Zealand secondary schools. Applied Ergonomics, 36(2), 193–198. https://doi.org/10.1016/j.apergo.2004.10.004 [Google Scholar] [CrossRef]
4. Calvo-Muñoz, I., Kovacs, F. M., Roqué, M., Seco-Calvo, J. (2020). The association between the weight of schoolbags and low back pain among schoolchildren: A systematic review, meta-analysis and individual patient data meta-analysis. European Journal of Pain, 24(1), 91–109. https://doi.org/10.1002/ejp.1471 [Google Scholar] [PubMed] [CrossRef]
5. Mackie, H. W., Stevenson, J. M., Reid, S. A., Legg, S. J. (2005). The effect of simulated school load carriage configurations on shoulder strap tension forces and shoulder interface pressure. Applied Ergonomics, 36(2), 199–206. https://doi.org/10.1016/j.apergo.2004.10.007 [Google Scholar] [PubMed] [CrossRef]
6. Mackenzie, W. G., Sampath, J. S., Kruse, R. W., Sheir-Neiss, G. J. (2003). Backpacks in children. Clinical Orthopaedics and Related Research (1976–2007), 409, 78–84. https://doi.org/10.1097/01.blo.0000058884.03274.d9 [Google Scholar] [PubMed] [CrossRef]
7. Brzęk, A., Dworrak, T., Strauss, M., Sanchis-Gomar, F., Sabbah, I. et al. (2017). The weight of pupils’ schoolbags in early school age and its influence on body posture. BMC Musculoskeletal Disorders, 18(1), 1–11. https://doi.org/10.1186/s12891-017-1462-z [Google Scholar] [CrossRef]
8. Al-Saleem, S. A., Ali, A., Ali, S. I., Alshamrani, A. A., Almulhem, A. M. (2016). A study of school bag weight and back pain among primary school children in Al-Ahsa, Saudi Arabia. Epidemiology (Sunnyvale), 6(1). https://doi.org/10.4172/2161-1165.1000222 [Google Scholar] [PubMed] [CrossRef]
9. Grimmer, K. A., Williams, M. T., Gill, T. K. (1999). The associations between adolescent head-on-neck posture, backpack weight, and anthropometric features. Spine, 24(21), 2262. https://doi.org/10.1097/00007632-199911010-00015 [Google Scholar] [PubMed] [CrossRef]
10. Chansirinukor, W., Wilson, D., Grimmer, K., Dansie, B. (2001). Effects of backpacks on students: Measurement of cervical and shoulder posture. Australian Journal of Physiotherapy, 47(2), 110–116. https://doi.org/10.1016/S0004-9514(14)60302-0 [Google Scholar] [PubMed] [CrossRef]
11. Chow, D., Ou, Z., Wang, X., Lai, A. (2010). Short-term effects of backpack load placement on spine deformation and repositioning error in schoolchildren. Ergonomics, 53(1), 56–64. https://doi.org/10.1080/00140130903389050 [Google Scholar] [CrossRef]
12. Southard, S. A., Mirka, G. A. (2007). An evaluation of backpack harness systems in non-neutral torso postures. Applied Ergonomics, 38(5), 541–547. https://doi.org/10.1016/j.apergo.2006.08.007 [Google Scholar] [CrossRef]
13. Goh, J., Thambyah, A., Bose, K. (1998). Effects of varying backpack loads on peak forces in the lumbosacral spine during walking. Clinical Biomechanics, 13(1), S26–S31. https://doi.org/10.1016/S0268-0033(97)00071-5 [Google Scholar] [PubMed] [CrossRef]
14. Cottalorda, J., Bourelle, S., Gautheron, V., Kohler, R. (2004). Backpack and spinal disease: Myth or reality? Revue de Chirurgie Orthopédique et Réparatrice de l’Appareil Moteur, 90(3), 207–214. https://doi.org/10.1016/S0035-1040(04)70096-3 [Google Scholar] [PubMed] [CrossRef]
15. Amiri, M., Dezfooli, M. S., Mortezaei, S. R. (2012). Designing an ergonomics backpack for student aged 7–9 with user centred design approach. Work, 41(Supplement 1), 1193–1201. https://doi.org/10.3233/WOR-2012-0303-1193 [Google Scholar] [CrossRef]
16. Putra, N. K., Sriwarno, A. B. (2010). Dynamic modeling and simulation of the suspended-load backpack to obtain optimal suspension parameters and reducing effect of ground reaction force. 6th World Congress of Biomechanics (WCB 2010), pp. 111–114. Singapore, Springer. [Google Scholar]
17. Chen, H., Shao, E., Sun, D., Xuan, R., Baker, J. S. et al. (2022). Effects of footwear with different longitudinal bending stiffness on biomechanical characteristics and muscular mechanics of lower limbs in adolescent runners. Frontiers in Physiology, 13, 907016. https://doi.org/10.3389/fphys.2022.907016 [Google Scholar] [PubMed] [CrossRef]
18. Zhang, Q., Zhang, Y., Chon, T. E., Baker, J. S., Gu, Y. (2022). Analysis of stress and stabilization in adolescent with osteoporotic idiopathic scoliosis: Finite element method. Computer Methods in Biomechanics and Biomedical Engineering, 26(1). https://doi.org/10.1080/10255842.2022.2044803 [Google Scholar] [PubMed] [CrossRef]
19. Zheng, J., Yang, Y., Lou, S., Zhang, D., Liao, S. (2015). Construction and validation of a three-dimensional finite element model of degenerative scoliosis. Journal of Orthopaedic Surgery and Research, 10(1), 189. https://doi.org/10.1186/s13018-015-0334-1 [Google Scholar] [PubMed] [CrossRef]
20. Zhang, Q., Chon, T., Zhang, Y., Baker, J. S., Gu, Y. (2021). Finite element analysis of the lumbar spine in adolescent idiopathic scoliosis subjected to different loads. Computers in Biology and Medicine, 136, 104745. https://doi.org/10.1016/j.compbiomed.2021.104745 [Google Scholar] [PubMed] [CrossRef]
21. Zhang, L., Zhang, Q., Zhang, Y., Arthur, M., Teo, E. C. et al. (2022). The effect of concave-side intertransverse ligament laxity on the stress of AIS lumbar spine based on finite element method. Bioengineering, 9(12), 724. https://doi.org/10.3390/bioengineering9120724 [Google Scholar] [PubMed] [CrossRef]
22. Rohlmann, A., Neller, S., Bergmann, G., Graichen, F., Claes, L. et al. (2001). Effect of an internal fixator and a bone graft on intersegmental spinal motion and intradiscal pressure in the adjacent regions. European Spine Journal, 10(4), 301–308. https://doi.org/10.1007/s005860100295 [Google Scholar] [CrossRef]
23. Wilke, H. J., Claes, L., Schmitt, H., Wolf, S. (1994). A universal spine tester for in vitro experiments with muscle force simulation. European Spine Journal, 3(2), 91–97. https://doi.org/10.1007/BF02221446 [Google Scholar] [PubMed] [CrossRef]
24. Zander, T., Rohlmann, A., Calisse, J., Bergmann, G. (2001). Estimation of muscle forces in the lumbar spine during upper-body inclination. Clinical Biomechanics, 16, S73–80. https://doi.org/10.1016/S0268-0033(00)00108-X [Google Scholar] [PubMed] [CrossRef]
25. Zhang, Q., Zhang, Y., Chon, T. E., Baker, J. S., Gu, Y. (2023). Analysis of stress and stabilization in adolescent with osteoporotic idiopathic scoliosis: Finite element method. Computer Methods in Biomechanics and Biomedical Engineering, 26(1), 12–24. https://doi.org/10.1080/10255842.2022.2044803 [Google Scholar] [PubMed] [CrossRef]
26. Mohd, K., Zailina, H., Shamsul, B., Nurul, M., Mohd, M. et al. (2010). Neck, upper back and lower back pain and associated risk factors among primary school children. Journal of Applied Sciences, 10(5), 431–435. https://doi.org/10.3923/jas.2010.431.435 [Google Scholar] [CrossRef]
27. Farhood, H. F. (2013). Low back pain in schoolchildren: The role of school bag weight and carrying way. Journal Natural Sciences Research, 3(8), 156–164. [Google Scholar]
28. Grimmer, K., Dansie, B., Milanese, S., Pirunsan, U., Trott, P. (2002). Adolescent standing postural response to backpack loads: A randomised controlled experimental study. BMC Musculoskeletal Disorders, 3, 1–10. https://doi.org/10.1186/1471-2474-3-10 [Google Scholar] [PubMed] [CrossRef]
29. Dianat, I., Javadivala, Z., Allahverdipour, H. (2011). School bag weight and the occurrence of shoulder, hand/wrist and low back symptoms among Iranian elementary schoolchildren. Health Promotion Perspectives, 1, 76–85. https://doi.org/10.5681/hpp.2011.008 [Google Scholar] [CrossRef]
30. Hundekari, J., Chilwant, K., Vedpathak, S., Wadde, S. (2013). Does alteration in backpack load affects posture of school children. Group, 2, 10–20. [Google Scholar]
31. Costello, K. E., Matrangola, S. L., Madigan, M. L. (2012). Independent effects of adding weight and inertia on balance during quiet standing. Biomedical Engineering OnLine, 11(1), 1–13. https://doi.org/10.1186/1475-925X-11-20 [Google Scholar] [PubMed] [CrossRef]
32. Brackley, H. M., Stevenson, J. M. (2004). Are children’s backpack weight limits enough? A critical review of the relevant literature. Spine, 29(19), 2184–2190. https://doi.org/10.1097/01.brs.0000141183.20124.a9 [Google Scholar] [CrossRef]
33. Devroey, C., Jonkers, I., Becker, A., Lenaerts, G., Spaepen, A. (2007). Evaluation of the effect of backpack load and position during standing and walking using biomechanical, physiological and subjective measures. Ergonomics, 50(5), 728–742. https://doi.org/10.1080/00140130701194850 [Google Scholar] [CrossRef]
34. Bobet, J., Norman, R. (1984). Effects of load placement on back muscle activity in load carriage. European Journal of Applied Physiology and Occupational Physiology, 53(1), 71–75. https://doi.org/10.1007/BF00964693 [Google Scholar] [CrossRef]
35. Khalil, A. L., Qato, A. (2012). The influence of backpacks on students backs: A cross-sectional study of schools in Tulkarm district (Master’s Thesis). An-Najah National University, Nablus, Palestine. [Google Scholar]
36. Ramprasad, M., Alias, J., Raghuveer, A. (2010). Effect of backpack weight on postural angles in preadolescent children. Indian Pediatrics, 47(7), 575–580. https://doi.org/10.1007/s13312-010-0130-2 [Google Scholar] [PubMed] [CrossRef]
37. Copley, P. C., Tilliridou, V., Kirby, A., Jones, J., Kandasamy, J. (2019). Management of cervical spine trauma in children. European Journal of Trauma and Emergency Surgery, 45(5), 777–789. https://doi.org/10.1007/s00068-018-0992-x [Google Scholar] [CrossRef]
38. Mosaad, D. M., Abdel-Aziem, A. A. (2015). Backpack carriage effect on head posture and ground reaction forces in school children. Work, 52(1), 203–209. https://doi.org/10.3233/WOR-152043 [Google Scholar] [PubMed] [CrossRef]
39. Song, Q., Yu, B., Zhang, C., Sun, W., Mao, D. (2014). Effects of backpack weight on posture, gait patterns and ground reaction forces of male children with obesity during stair descent. Research in Sports Medicine, 22(2), 172–184. https://doi.org/10.1080/15438627.2014.881823 [Google Scholar] [PubMed] [CrossRef]
40. Orantes-Gonzalez, E., Heredia-Jimenez, J., Beneck, G. J. (2017). Children require less gait kinematic adaptations to pull a trolley than to carry a backpack. Gait & Posture, 52, 189–193. https://doi.org/10.1016/j.gaitpost.2016.11.041 [Google Scholar] [PubMed] [CrossRef]
41. Snijders, C. J., Hermans, P. F., Niesing, R., Spoor, C. W., Stoeckart, R. (2004). The influence of slouching and lumbar support on iliolumbar ligaments, intervertebral discs and sacroiliac joints. Clinical Biomechanics, 19(4), 323–329. https://doi.org/10.1016/j.clinbiomech.2004.01.006 [Google Scholar] [CrossRef]
42. Mallakzadeh, M., Javidi, M., Azimi, S., Monshizadeh, H. (2016). Analyzing the potential benefits of using a backpack with non-flexible straps. Work, 54(1), 11–20. https://doi.org/10.3233/WOR-162293 [Google Scholar] [CrossRef]
43. Ji, Y., Zhang, Q., Song, Y., Hu, Q., Fekete, G. et al. (2022). Biomechanical characteristics of 2 different posterior fixation methods of bilateral pedicle screws: A finite element analysis. Medicine, 101(36), e30419. https://doi.org/10.1097/MD.0000000000030419 [Google Scholar] [PubMed] [CrossRef]
44. Negrini, S., Carabalona, R. (2002). Backpacks on! Schoolchildren’s perceptions of load, associations with back pain and factors determining the load. Spine, 27(2), 187–195. https://doi.org/10.1097/00007632-200201150-00014 [Google Scholar] [PubMed] [CrossRef]
45. Bajin, M., Kojić, M., Romanov, R., Ahmetović, Z. (2022). Neglected problem: Influence of school bag on lumbar segment in children. Frontiers in Pediatrics, 10, 1045666. https://doi.org/10.3389/fped.2022.1045666 [Google Scholar] [PubMed] [CrossRef]
46. Ge, T., Hu, B., Zhang, Q., Xiao, J., Wu, X. et al. (2023). Biomechanical evaluation of two-level oblique lumbar interbody fusion combined with posterior four-screw fixation: A finite element analysis. Clinical Neurology and Neurosurgery, 225(1), 107597. https://doi.org/10.1016/j.clineuro.2023.107597 [Google Scholar] [PubMed] [CrossRef]
47. Zhang, Q., Zhang, Y., Huang, J., Teo, E. C., Gu, Y. (2022). Effect of displacement degree of distal chevron osteotomy on metatarsal stress: A finite element method. Biology, 11(1), 127. https://doi.org/10.3390/biology11010127 [Google Scholar] [PubMed] [CrossRef]
Cite This Article
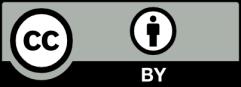
This work is licensed under a Creative Commons Attribution 4.0 International License , which permits unrestricted use, distribution, and reproduction in any medium, provided the original work is properly cited.