Open Access
ARTICLE
Lower Limb Muscle Forces in Table Tennis Footwork during Topspin Forehand Stroke Based on the OpenSim Musculoskeletal Model: A Pilot Study
1 Faculty of Sports Science, Ningbo University, Ningbo, 315211, China
2 Faculty of Engineering, University of Pannonia, Veszprém, H8201, Hungary
3 Savaria Institute of Technology, EötvösLoránd University, Szombathely, H9700, Hungary
4 Doctoral School on Safety and Security Sciences, Obuda University, Budapest, 1034, Hungary
* Corresponding Authors: Dong Sun. Email: ; Yaodong Gu. Email:
Molecular & Cellular Biomechanics 2022, 19(4), 221-235. https://doi.org/10.32604/mcb.2022.027285
Received 23 October 2022; Accepted 06 December 2022; Issue published 27 December 2022
Abstract
Introduction: Footwork is one of the training contents that table tennis players and coaches focus on. This study aimed to gain a thorough understanding of the muscle activity of the table tennis footwork and creating a musculoskeletal model to investigate the muscle forces, joint kinematic, and joint kinetic characteristics of the footwork during topspin forehand stroke. Methods: Six male table tennis athletes (height: 171.98 ± 4.97 cm; weight: 68.77 ± 7.86 kg; experience: 10.67 ± 1.86 years; age: 22.50 ± 1.64 years) performed chasse step and one-step footwork to return the ball from the coach by topspin forehand stroke. The kinematics, kinetics, and muscle activity of the lower limb were recorded by the motion capture, force platform, and Electromyography (EMG) system. Statistical parametric mapping (SPM) analysis was used to investigate any difference between the chasse step and one-step footwork during the stroke. Results and Conclusion: The muscle force of the biceps femoris long head (p < 0.001), lateral gastrocnemius (p < 0.001), vastus lateralis (p < 0.001), vastus medial (p < 0.001), rectus femoris (p < 0.001), and tibia anterior (p < 0.001) of the chasse step were significantly greater than the one-step footwork during the early stroke phase (stance). At the end of the stroke phase (push-off), the muscle force of the biceps femoris long head (p < 0.001), medial gastrocnemius (p < 0.001), lateral gastrocnemius (p < 0.001), rectus femoris (p < 0.001), and tibias anterior (p < 0.001) in the chasse step footwork was significantly greater than the one-step footwork. The muscle force of the ankle plantar flexor and valgus muscle groups in the one-step was significantly greater than in the chasse step. Besides, the moment and angle of hip flexion (p = 0.001) and axial rotation (p = 0.009) were significantly greater for the chasse step than the one-step footwork, as well as the ankle plantarflexion angle (p < 0.001) and moment (p < 0.001) of the one-step footwork were significantly higher than the chasse step footwork. The results of this study can be applied to movement control and injury prevention in table tennis footwork.Keywords
Table tennis is a highly competitive racket sport, as one of the most popular racket sports attract a large number of players worldwide [1,2]. Technical-tactical skills were recognized as the most important element in enhancing the performance of athletes in table tennis [3,4]. And the performance requirements of the players and the intensity and speed of the movements have been enhanced due to changes in rules and equipment in table tennis [5]. As a result, further obtaining biomechanical information about athletes’ bodies through modern technology and revealing the internal mechanisms of joints and muscles during movement is significant for athletes and coaches.
Sports biomechanics is the quantitative study and analysis of the mechanics of professional athletes in general sports [6]. A large number of studies have been reported on the progress of biomechanical research in table tennis [2,4,7]. Bańkosz et al. [8] investigated the kinematics information of topspin backhand between Chinese and Polish female table tennis athletes and they investigated the different playing styles of the group’s study and coaching system. He et al. [2] compared the kinematic information of topspin forehand between different level table tennis athletes, they investigated the key difference that the ankle joint played. MalagoliLanzoni et al. [9] investigated the kinematics information of table tennis rackets during topspin forehand strokes. The same research team compared the kinematics of table tennis players using the long line and cross-court topspin forehand loop. They mentioned a significantly larger flexion of the right knee at the moment of maximum racket velocity during the long line [10]. These studies have shed some light on the mechanisms underlying the topspin forehand stroke technique in table tennis from a kinematic perspective. In addition, several studies have revealed the intrinsic influence of kinetics on table tennis topspin stroke techniques. Lino et al. [11] reported the significance of mechanical energy generation and transfer to the speed of the racket during the topspin forehand between the different level athletes. The same research team investigated the effects of racket mass and stroke speed on the kinetics of the upper limb and trunk in table tennis backhand topspin stroke [12]. These authors’ studies reveal the mechanisms of energy production and transfer during topspin ball-striking movement and the effect on racket speed. And provide theoretical guidance for the optimization of stroke skills, especially topspin forehand stroke. Meanwhile, these studies have provided strong evidence that biomechanical research is of great importance for the optimization and development of table tennis.
In table tennis competitions, athletes have to play good strategies to win the match [13]. Footwork is one of the core skills that table tennis players need to master. Athletes have to return to the ready position for the next movement during the match. Good footwork plays an important role in balancing dynamic stability and agility [7]. Biomechanical research on footwork in table tennis has received a lot of attention in recent years. Yang et al. [14] compared the kinematics information of the chasse step between male and female athletes. The range of motion and joint angle of the knee is significantly greater in male athletes than in female athletes in the movement of topspin stroke using the chasse step. He et al. [15] compared the plantar kinetics characteristics between the one-step and chasse step footwork during topspin stroke based on the one-dimensional statistical parameter mapping (SPM 1d), they reported that in addition to using cushioning strategies to reduce the load on the dominant leg during landing, athletes can also achieve greater weight transfer by enhancing plantarflexion. These scholarly studies reveal the mechanisms underlying the kinematic characteristics of joints, ground reaction forces, and plantar kinetic information in the forehand topspin movement with the chasse step, one-step, and cross-step footwork. However, due to experimental conditions and technical limitations, previous biomechanical studies on footwork techniques have focused less on muscle activity, such as information on the muscle forces of the athlete when performing different footwork.
The application of technological innovation tools plays an important role in investigating biomechanical evidence [16,17]. The study of muscle force information enables further understanding of the mechanisms and functions inherent in motor tasks, as well as providing diagnostic and therapeutic tools for sports injuries and rehabilitation in the field of clinical medicine [18]. Such as the example of gait pattern in cerebral palsy treatment and the recovery process for injured athletes [19]. The musculoskeletal model consists of bones connected by joints, to which the muscles are attached, forcing the joints to move [20–22]. Musculoskeletal models used in conjunction with non-invasive measurement methods allow the strength of individual muscles to be obtained for several motor tasks [19]. The use of the OpenSim modeling environment to construct musculoskeletal models to explore the biomechanical characteristics of movement has been widely used [20,23]. However, to our knowledge, there is very limited research related to the use of creating musculoskeletal models to explore muscle force information for table tennis footwork. To gain a thorough understanding of the muscle activity information of the table tennis footwork technique and to further reveal its intrinsic mechanisms and functions, this study aimed to create a musculoskeletal model using OpenSim software to investigate the muscle forces, joint kinematic, and joint kinetic characteristics between the chasse step and one-step during topspin forehand stroke. The study can provide the guidelines to be applied to movement control and injury prevention in table tennis footwork. It was hypothesized that the different footwork would exhibit distinct muscle force, joint kinematics, and joint kinetics character.
Six male national-level table tennis athletes (height: 171.98 ± 4.97 cm; weight: 68.77 ± 7.86 kg; experience: 10.67 ± 1.86 years; age: 22.50 ± 1.64 years) from Ningbo University were recruited to participate in this study. All subjects were free from any neuromuscular injury within 6 months, while all subjects were right-handed. Before the start of the formal experiment, all subjects were fully informed of the purpose, process, and requirements of the study, and all subjects provided informed consent. The Ethics Committee of Ningbo University approved this study (RAGH202108223005.7).
2.2 Experimental Protocol and Equipment
This experiment was carried out in the biomechanics laboratory of the Ningbo University Research Academy of Grand Health. An eight-camera Vicon motion capture system (Oxford Metrics, Ltd., Oxford, UK) was used with a force platform (Kistler, Switzerland) to record the kinematics and kinetics data of subjects during movement. The kinematics and kinetics information was captured at 200 and 1000 Hz, respectively. Besides, an electromyography (EMG) system (Delsys, Boston, MA, USA) was used to record the muscle activity at 1000 Hz. Muscle force and activation of five muscles of the right leg were recorded in this study (Medial gastrocnemius, Lateral gastrocnemius, Semitendinosus, Rectus femoris, and Tibialis anterior). The mid-point of each muscle was select for attaching the EMG electrodes shown in Fig. 1. The Gait2392 model was selected to complete the musculoskeletal modeling in the OpenSim (Stanford University, Stanford, CA, USA), with the thirty-nine reflective markers (12.5 mm in diameter) placement shown in Fig. 1.
Figure 1: Illustration of the placement of the reflective markers and EMG electrodes on three sides. The reflective markers were shown as the red point, and the EMG electrodes were shown as the blue rectangle
Subjects were asked to wear tights and match table tennis shoes during the experiment and to use uniform rackets with the DHC Hurricane 3 and Butterfly Tenergy 05 Max rubber sheets, as well as uniform table tennis balls to complete the test on a professional table tennis table.
At first, subjects completed 10 min of running and 5 min of static stretching at an adaptive speed in the playground. Secondly, the selected muscle surface skin was cleaned to avoid affecting the accuracy of EMG data (the skin surface of the selected muscle is first shaved with a razor, and then the skin is cleaned with an alcohol swab), and then the maximal voluntary contraction (MVC) collection of all muscles was completed. Subjects were required to stand on the force platform to finish the static coordinates collection. Before the formal test, subjects were allowed to complete five test tasks as a way of helping them quickly familiarize themselves with the laboratory environment.
As shown in Fig. 2A, the process of “a–i” was performed in the chasse step footwork, and the process of “j–n” was performed in the one-step footwork. In the chasse step footwork, the “a” shows the ready position. The “a–b” and “e–g” show the backward phase of the first stroke process and second stroke process, respectively. The “b–d” and “g–i” show the forward phase of the first stroke process and second stroke process, respectively. In the one-step footwork, the “j” shows the ready position, the “j–l” shows the backward phase, as well as the “l–n” shows the forward phase.
Figure 2: (A) Illustration of chasse step and one step footwork. (B) Illustration of experiment process of a table tennis stroke
In the formal test, the coach was asked to shoot the ball with normal served to the first impact zone (25 cm * 30 cm) and final impact zone (25 cm * 30 cm), respectively. Subjects were asked to perform topspin forehand by the one-step and chasse step footwork to stroke the ball from the coach to the target area (25 cm * 30 cm), as shown in Fig. 2B. The length and width of the impact zone and target area are set concerning previous studies [13]. Subjects were asked to complete three successful strokes by chasse step footwork, then complete three successful strokes by one-step footwork. The motion capture system, EMG system, and force platform were connected by electrical signals to achieve the multi-parameter synchronous acquisition of the data of EMG, kinetic, and kinematic. The motion smoothness was judged by the subjects themselves, as well as the quality and effect of the ball play were supervised by a qualified table tennis coach. Experimental operators can also evaluate the validity of data collection based on data performance.
The surface electromyography (EMG) signals recorded in the experiment were converted into activation through RMS processing (0 indicates no activation and 1 indicates full activation), then it was compared with the activation obtained by the OpenSim optimization algorithm. A 4th-order band-pass filter between 10 and 500 Hz was applied to the EMG data before it was full-wave-rectified, as well as a 10 Hz low-pass filter was used to smooth data.
Fig. 3 showed the data process. Kinematics and ground reaction force (GRF) data of chasse step and one-step footwork during stroke were acquired and identified by the Vicon Nexus 1.8.6 software. The data was exported into a c3d. format file by the Vicon Nexus software, then performs coordinate system conversion, low-pass filtering, data extraction, and format conversion for kinematics and ground reaction force data by MATLAB R2019a (The MathWorks, Natick, MA, USA).
Figure 3: Flowchart of data processing
In MATLAB R2019a, perform the following steps: (1) converted the coordinate system of the kinematics and GRF data to the subsequent simulations coordinate system. (2) Use the 6 and 30 Hz fourth-order zero-phase lag Butterworth low-pass filters to filter the marker trajectory and the GRF. (3) The kinematics and GRF data of chasse step and one-step footwork during stroke were extracted and converted to the trc. and mot. formats required by the OpenSim simulation software.
In OpenSim, perform the following steps: (1) Import the statics model and obtain the anthropometric model of subjects by the scale tool. Identify the starting and ending point of muscle, as well as ensure the moment arms consistent with the subjects’ limb length [20,24]. (2) Calculate the kinematics data of the one-step and chasse step footwork during a stroke by the inverse kinematics tool (IK) and created a motion file (mot). Then, import the markers and GRF files by the inverse dynamics tool and calculate the joint moment of the subjects. (3) Smoothing the kinematics data by the residual reduction algorithm to improve the preciseness of the dynamic data to be consistent with the kinematics and kinetics data measured in the experimental. (4) Calculate the muscle activation and the muscle force by the computed muscle control (CMC) with the smoothed kinematics data calculated in the last step [20,25].
For statistical parametric mapping (SPM) analysis, lower limb joint angle and moment, as well as the muscle force of the chasse step and one-step footwork during stroke were extracted. All data of the stroke phase was expended into a time series curve of 101 data points by a MATLAB custom script. The open-source SPM1d paired samples t-test script was used to analyze the difference in joint angle, joint moment, and muscle force between the chasse step and one-step footwork during stroke [20,26,27]. The significance level was set at p < 0.05 in this study.
As shown in Fig. 4, the lower limb muscle EMG signals and activations from OpenSim optimization at the chasse step and one-step footwork during stroke were compared. Including biceps femoris long head (biceps femoris lh), lateral gastrocnemius, medial gastrocnemius, rectus femoris, semitendinosus, tibialis anterior, vastus lateralis, and vastus medialis. According to Fig. 4, The lower lime muscle activation of the chasse step and one-step footwork during stroke calculated by the musculoskeletal model was similar to the surface EMG signal recorded in the experiment, which indicated that the OpenSim model data in this study was relatively reliable.
Figure 4: Comparison of lower limb muscle EMG signals and activations from OpenSim Optimization between the chasse step and one step during stroke in table tennis
Fig. 5 showed the SPM analysis result of the lower limb joint angle of the chasse step and one-step footwork during the stroke. Fig. 6 showed the SPM analysis result of a joint moment of the chasse step and one-step footwork during the stroke. Fig. 7 showed the SPM analysis result of muscle force of the chasse step and the one-step footwork during the stroke. The ankle plantarflexion joint angle and moment of one-step footwork were significantly higher than chasse step footwork in the 50.51%–87.75% (p < 0.001), 55.75%–87.00% (p < 0.001) stroke phase, respectively. The subtalar joint valgus angle and moment of in the one-step footwork were significantly higher than the chasse step footwork in the 18.5%–100% (p < 0.001) and 56.71%–86.47% stroke phase (p < 0.001), respectively. The knee flexion angle and moment of the one-step footwork were significantly higher than the chasse step footwork in the 42.83%–100% (p < 0.001), and 48.57%–87.21% stroke phase (p < 0.001), respectively. However, the knee flexion angle and moment in 8.61%–30.19% (p = 0.008) and 2.82%–40.97% (p < 0.001) stroke phase of the chasse step were significantly higher than the one-step footwork. Besides, the hip flexion angle in the 16.55%–49.23% stroke phase (p = 0.001) and the hip extension moment in the 32.63%–58.37% stroke phase (p < 0.001) are significantly greater in the chasse step than the one-step footwork. The chasse step footwork shows a significantly higher angle of hip abduction in the 59.77%–69.80% stroke phase (p = 0.009). The chasse step footwork hip external rotation angle in 48.20%–73.85%, 93.05%–100% stroke phase and moment in 58.84%–77.88%, 86.87%–100% stroke phase was significantly higher than the one-step footwork, respectively.
Figure 5: Illustration of the result between the chasse step and one step showing the statistical parametric mapping outputs for the lower limb joint angle during the stroke phase. Grey-shaded areas indicate that there are significant differences (p < 0.05) between the chasse step and one step
Figure 6: Illustration of the result between the chasse step and one step showing the statistical parametric mapping outputs for the lower limb joint moment during the stroke phase. Grey-shaded areas indicate that there are significant differences (p < 0.05) between the chasse step and one step
Figure 7: Illustration of the results between the chasse step and one step showing the statistical parametric mapping outputs for the lower limb muscle force during the stroke phase. Grey-shaded areas indicate that there are significant differences (p < 0.05) between the chasse step and one step
The muscle force of the biceps femoris in the chasse step footwork was significantly greater than the one-step footwork in the 24.42%–50.87% (p < 0.001) and 88.04%–100% (p < 0.001) stroke phase. The muscle force of the lateral gastrocnemius in the chasse step footwork in 9.52%–47.54% (p < 0.001) and 86.89%–99.68% (p < 0.001) stroke phase as well as medial gastrocnemius muscle force in 28.55%–51.56% (p < 0.001) and 89.67%–100% (p < 0.001) stroke phase is significantly greater than one step. The muscle force of the medial gastrocnemius and the lateral gastrocnemius of the one-step footwork was significantly greater than the chasse step footwork in 63.75%–81.78% and 61.13%–82.29% stroke phase, respectively. Lateral vastus muscle forces in the 8.67%–43.45% (p < 0.001) and medial vastus muscle forces in the 8.40%–44.10% (p < 0.001) stroke phase in chasse step were significantly greater than the one-step footwork. The rectus femoris muscle force in the 16.54%–34.49% (p < 0.001) and 87.14%–100% (p < 0.001) stroke phase in the chasse step was significantly greater than one-step, but significantly less than one-step footwork in the 44.14%–56.65% (p < 0.001) and 65.88%–80.87% (p < 0.001) stroke phase. The semitendinosus muscle force in the chasse step was significantly greater than one-step footwork during the 29.45%–52.01% (p < 0.001) stroke phase. The tibialis anterior muscle force in the chasse step during 14.93%–39.56% (p < 0.001), 51.43%–65.58% (p < 0.001) and 88.74%–100% (p < 0.001) stroke phase was significantly greater than one-step, but significantly less than one-step footwork in the 71.24%–82.61% (p < 0.001) stroke phase.
Chasse step and one-step footwork are highly repetitive movements in table tennis that helps athletes to reach an appropriate position and area to execute topspin forehands [1,27–29]. This study uses an individualized OpenSim musculoskeletal model to reveal the joint angle, joint moment, and muscle force characteristics of the lower limb joints during a stroke with the chasse step and the one-step footwork, which further investigate the internal mechanism of energy transfer and biomechanical in table tennis footwork and the risk of possible sports injury. The key findings of this study were that the muscle force of the biceps femoris long head, lateral gastrocnemius, vastus lateralis, vastus medial, rectus femoris, and tibia anterior of the chasse step was significantly greater than the one-step footwork during the early stroke phase (stance). At the end of the stroke phase (push-off), the muscle force of the biceps femoris long head, medial gastrocnemius, lateral gastrocnemius, rectus femoris, and tibias anterior in the chasse step footwork was significantly greater than the one-step footwork. The muscle force of the ankle plantar flexor and valgus muscle groups in the one-step was significantly greater than in the chasse step. Besides, the moment and angle of hip flexion and axial rotation were significantly greater for the chasse step than the one-step footwork, as well as the ankle plantarflexion angle and moment of the one-step footwork were significantly higher than the chasse step footwork. The results of this study were consistent with our hypothesis that both footwork had significant differences in muscle strength, joint angles, and moments. This study can provide theoretical guidance for motion control and injury prevention to table tennis players and coaches.
Overall, the moment and angle of hip flexion and axial rotation were significantly greater for the chasse step than for the one-step footwork, which is consistent with previous studies [7,28]. Compared to one-step footwork, the chasse step requires a greater distance and is accompanied by a full-body weight transfer. Hip flexion and axial rotation moments add to the racket’s maximum acceleration by the kinetic chain that follows the proximal-to-distal segmental sequences [28,30,31], and the transfer of whole body weight creates greater energy transfer, further enhancing the stroke impact. The ankle plantarflexion angle and moment of the one-step footwork were significantly greater than those of the chasse step footwork, which was inconsistent with previous studies [15,28], and the possible reason was that the difference in the distance traveled in the one-step footwork and the difference in ball speed resulted in athlete adjustment different motor control strategies of the lower limb. The muscle force of the biceps femoris lh, lateral gastrocnemius, vastus lateralis, vastus medial, rectus femoris, and tibia anterior of the chasse step is significantly greater than the one-step footwork during the early stroke phase (stance). At the end of the stroke phase (push-off), the muscle force of the biceps femoris lh, medial and lateral gastrocnemius, rectus femoris, and tibias anterior in the chasse step footwork is significantly greater than the one-step footwork. This may be because the chasse step footwork is to quickly reach the target area to complete the stroke movement by transferring the weight of the whole body through the large movement of the full feet, which results in a more powerful load on landing, so the lower limb muscles are required to provide more powerful muscle force to maintain the stability of the joints and the quality of the movements, this finding consistent with previous studies [13,15,32]. The large-scale transfer of whole-body weight leads to a large-scale transfer in the center of gravity, so at the end of the stroke phase, the lower limb muscles need to provide stronger muscle force to prepare for the next transfer in the center of gravity. A high-quality hitting movement through continuous movement in all directions in a limited area, and the stronger lower limb driving force provide the basis for this rapid and frequent change of directional movement, which is consistent with previous research [33]. Besides, to perform a high-rotation, low-height forehand topspin, the player must bend the knee strongly [34], suggesting important contributions from knee flexor and extensor muscle groups such as the biceps femoris, vastus medialis, vastus lateralis, rectus femoris. Racquet athletes rely more on the movement of the dominant leg [28]. This can lead to a high muscle asymmetry degree in the extremities of racket athletes, and greater asymmetry of muscle can disrupt the movement rhythm and increase the sports injury risk [28,35]. The greater knee flexion angle and moment in the chasse step footwork during the early stroke phase (stance) means that is more effective in joint rotation which promotes energy accumulation. Greater knee flexion allows athletes to better utilize energy transfer throughout the kinematic chain to achieve a proper velocity of the racket based on the proximal-to-distal segmental sequences [36]. And the agreed result has been reported in a previous study [28]. Also, according to Fig. 6, the change in knee moment for the chasse step footwork shows a faster rate of energy transfer, which may also explain the flexibility of the chasse step relative to the one-step footwork. However, attention should also be paid to the risk of sports injuries caused by excessive knee fatigue that may result from prolonged training with the chasse step footwork. The larger loading magnitudes on the ankle and knee joint found in the chasse step and cross-step footwork during the topspin forehand would predispose the table tennis athletes to overuse conditions such as jumper knee [37] and ankle sprain injuries [38].
Combine Figs. 5–7 we can observe that the one-step footwork was significantly higher than the chasse step footwork on the parameters of the joint angle and joint moment of plantar flexion and valgus, as well as the muscle force of the plantar flexor and valgus muscle groups. It means that greater muscle force of the medial and lateral gastrocnemius in the one-step footwork provides greater plantarflexion moments and angles. This may explain why gastrocnemius muscle activity is higher when the heel take-off due to plantar flexion during stroke [39,40]. Besides, the significantly higher knee flexion angle was observed in one-step footwork, greater knee flexion and greater plantarflexion allow athletes to better utilize energy transfer throughout the kinematic chain to achieve a proper velocity of racket based on the proximal-to-distal segmental sequences [36].
However, there are a few limitations to this study. Firstly, joint forces were not examined in this study. In future research, the joint contact forces of the knee and ankle joints during forehand topspin stroke need to be detected and considered to accurately diagnose and prevent sports injuries. Secondly, this study did not detect the racket velocity, upper limb movement, and the velocity of the ball shooted by the coach. In future research, we will consider combining the racket movement with the biomechanical information of the whole body joints and muscle force information to further explore the key information of table tennis movement. Thirdly, there are only male participants in this research, so the result of this research hasn’t considered the gender factor. Finlay, the sample size of this research has to be mentioned. There was a total of six national-level players who joined this study, finding a large number of athletes of the same level was not easy. Due to the limitation of the sample size, the practical application of the results of this study may require further support from future studies.
The lower limb muscle force, joint angle, and moment of table tennis footwork during topspin forehand stroke were investigated based on the OpenSim musculoskeletal model and statistical parametric mapping analysis. The results of this study can be applied to movement control and injury prevention in table tennis footwork. Based on the results of this study, we recommend (1) strengthening the knee flexor and extensor muscle groups, such as the rectus femoris, biceps femoris, vastus lateralis, vastus medialis, medial gastrocnemius, and lateral gastrocnemius, to strengthen the chasse step footwork during landing to create a stable backward phase, (2) strengthen the hip flexor/extensor muscles, brings a gain to the racket’s maximum acceleration; (3) Strengthen the plantarflexor muscle groups, such as the medial gastrocnemius and lateral gastrocnemius, thereby enhancing the power transfer of the one-step footwork at the end stroke phase during topspin forehand; (4) Strengthen the muscle strength training of the non-dominant legs of table tennis players to reduce the risk of sports injuries.
Authorship: Study conception and design: Yuqi He, Shirui Shao, Yaodong Gu. Data collection: Yuqi He, Xiaoyi Yang. Analysis and interpretation of results: Yuqi He, Gusztáv Fekete, Xuanzhen Cen, Yang Song. Draft manuscript preparation: Yuqi He, Dong Sun, Yaodong Gu. All authors reviewed the results and approved the final version of the manuscript.
Funding Statement: This study was sponsored by the Major Program of the National Natural Science Foundation of China (19ZDA352), Zhejiang Provincial Key Research and Development Program of China (2021C03130), Zhejiang Provincial Natural Science Foundation of China for Distinguished Young Scholars (LR22A020002), Philosophy and Social Sciences Project of Zhejiang Province, China (22QNYC10ZD, 22NDQN223YB), Educational Science Planning Project of Zhejiang Province (2021SCG083), Public Welfare Science & Technology Project of Ningbo, China (2021S134) and K. C. Wong Magna Fund in Ningbo University.
Conflicts of Interest: The authors declare that they have no conflicts of interest to report regarding the present study.
References
1. Fuchs, M., Liu, R., MalagoliLanzoni, I., Munivrana, G., Straub, G. et al. (2018). Table tennis match analysis: A review. Journal of Sports Science, 36(23), 2653–2662. DOI 10.1080/02640414.2018.1450073. [Google Scholar] [CrossRef]
2. He, Y., Lyu, X., Sun, D., Baker, J. S., Gu, Y. (2021). The kinematic analysis of the lower limb during topspin forehand loop between different level table tennis athletes. PeerJ, 9(7), e10841. DOI 10.7717/peerj.10841. [Google Scholar] [CrossRef]
3. MalagoliLanzoni, I., Katsikadelis, M., Straub, G., Djokic, Z. (2019). Footwork technique used in elite table tennis matches. International Journal of Racket Sports Science, 1(2), 44–48. [Google Scholar]
4. Iordan, D. A., Mocanu, G. D., Mocanu, M. D., Munteanu, C., Constantin, G. B. et al. (2021). Age-related, sport-specific dysfunctions of the shoulder and pelvic girdle in athletes table tennis players. Observational study. Balneo and PRM Research Journal, 12(4), 337–344. [Google Scholar]
5. Pradas, F., de la Torre, A., Carrasco, L., Muñoz, D., Courel-Ibáñez, J. et al. (2021). Anthropometric profiles in table tennis players: Analysis of sex, age, and ranking. Applied Sciences, 11(2), 876. DOI 10.3390/app11020876. [Google Scholar] [CrossRef]
6. Bini, R., Daly, L., Kingsley, M. (2020). Changes in body position on the bike during seated sprint cycling: Applications to bike fitting. European Journal of Sport Science, 20(1), 35–42. DOI 10.1080/17461391.2019.1610075. [Google Scholar] [CrossRef]
7. Wong, D. W. C., Lee, W. C. C., Lam, W. K. (2020). Biomechanics of table tennis: A systematic scoping review of playing levels and maneuvers. Applied Sciences, 10(15), 5203. DOI 10.3390/app10155203. [Google Scholar] [CrossRef]
8. Bańkosz, Z., Winiarski, S. (2021). The application of statistical parametric mapping to evaluate differences in topspin backhand between chinese and polish female table tennis players. Applied Bionics and Biomechanics, 5555874. DOI 10.1155/2021/5555874. [Google Scholar] [CrossRef]
9. MalagoliLanzoni, I., Bartolomei, S., Di Michele, R., Gu, Y., Baker, J. S. et al. (2021). Kinematic analysis of the racket position during the table tennis top spin forehand stroke. Applied Sciences, 11, 5178. DOI 10.3390/app11115178. [Google Scholar] [CrossRef]
10. MalagoliLanzoni, I., Bartolomei, S., di Michele, R., Fantozzi, S. (2018). A kinematic comparison between long-line and cross-court top spin forehand in competitive table tennis players. Journal of Sports Sciences, 36(23), 2637–2643. DOI 10.1080/02640414.2018.1456394. [Google Scholar] [CrossRef]
11. Iino, Y., Kojima, T. (2011). Kinetics of the upper limb during table tennis topspin forehands in advanced and intermediate players. Sports Biomechanics, 10(4), 361–377. [Google Scholar]
12. Iino, Y., Kojima, T. (2016). Effect of the racket mass and the rate of strokes on kinematics and kinetics in the table tennis topspin backhand. Journal of Sports Sciences, 34(8), 721–729. DOI 10.1080/02640414.2015.1069377. [Google Scholar] [CrossRef]
13. Zhou, H., He, Y., Yang, X., Ren, F., Ugbolue, U. C. et al. (2021). Comparison of kinetic characteristics of footwork during stroke in table tennis: Cross-step and chasse step. Journal of Visualized Experiments, 172, e62571. DOI 10.3791/62571. [Google Scholar] [CrossRef]
14. Yang, X., He, Y., Shao, S., Baker, J. S., István, B. et al. (2021). Gender differences in kinematic analysis of the lower limbs during the chasse step in table tennis athletes. Healthcare, 9(6), 703. DOI 10.3390/healthcare9060703. [Google Scholar] [CrossRef]
15. He, Y., Sun, D., Yang, X., Fekete, G., Baker, J. S. et al. (2021). Lower limb kinetic comparisons between the chasse step and one step footwork during stroke play in table tennis. PeerJ, 9(7), e12481. DOI 10.7717/peerj.12481. [Google Scholar] [CrossRef]
16. Gu, C., Chen, J., Lin, J., Lin, S., Wu, W. et al. (2022). The impact of eye-tracking games as a training case on students’ learning interest and continuous learning intention in game design courses: Taking flappy bird as an example. Learning and Motivation, 78(16), 101808. DOI 10.1016/j.lmot.2022.101808. [Google Scholar] [CrossRef]
17. Merry, C., Baker, J. S., Dutheil, F., Ugbolue, U. C. (2022). Do kinematic study assessments improve accuracy & precision in golf putting? A comparison between elite and amateur golfers: A systematic review and meta-analysis. Physical Activity and Health, 6(1), 108–123. DOI 10.5334/paah.159. [Google Scholar] [CrossRef]
18. Trinler, U., Schwameder, H., Baker, R., Alexander, N. (2019). Muscle force estimation in clinical gait analysis using anybody and opensim. Journal of Biomechanics, 86, 55–63. DOI 10.1016/j.jbiomech.2019.01.045. [Google Scholar] [CrossRef]
19. Erdemir, A., McLean, S., Herzog, W., van den Bogert, A. J. (2007). Model-based estimation of muscle forces exerted during movements. Clinical Biomechanics, 22(2), 131–154. DOI 10.1016/j.clinbiomech.2006.09.005. [Google Scholar] [CrossRef]
20. Zhou, H., Xu, D., Quan, W., Liang, M., Ugbolue, U. C. et al. (2021). A pilot study of muscle force between normal shoes and bionic shoes during men walking and running stance phase using opensim. Actuators, 10(10), 274. DOI 10.3390/act10100274. [Google Scholar] [CrossRef]
21. Sun, D., Fekete, G., Baker, J. S., Mei, Q., István, B. et al. (2020). A pilot study of musculoskeletal abnormalities in patients in recovery from a unilateral rupture-repaired achilles tendon. International Journal of Environmental Research and Public Health, 17(13), 4642. DOI 10.3390/ijerph17134642. [Google Scholar] [CrossRef]
22. Yu, L., Mei, Q., Mohamad, N. I., Gu, Y., Fernandez, J. (2021). An exploratory investigation of patellofemoral joint loadings during directional lunges in badminton. Computers in Biology and Medicine, 132, 104302. DOI 10.1016/j.compbiomed.2021.104302. [Google Scholar] [CrossRef]
23. Alexander, N., Schwameder, H., Baker, R., Trinler, U. (2021). Effect of different walking speeds on joint and muscle force estimation using anybody and opensim. Gait & Posture, 90, 197–203. DOI 10.1016/j.gaitpost.2021.08.026. [Google Scholar] [CrossRef]
24. Delp, S. L., Anderson, F. C., Arnold, A. S., Loan, P., Habib, A. et al. (2007). Opensim: Open-sourcesoftware to create and analyze dynamic simulations of movement. IEEE Transactions on Biomedical Engineering, 54(11), 1940–1950. DOI 10.1109/TBME.2007.901024. [Google Scholar] [CrossRef]
25. Thelen, D. G., Anderson, F. C., Delp, S. L. (2003). Generating dynamic simulations of movement using computed muscle control. Journal of Biomechanics, 36(3), 321–328. DOI 10.1016/S0021-9290(02)00432-3. [Google Scholar] [CrossRef]
26. Xu, D., Zhou, H., Baker, J. S., István, B., Gu, Y. (2021). An investigation of differences in lower extremity biomechanics during single-leg landing from height using bionic shoes and normal shoes. Frontiers in Bioengineering and Biotechnology, 9, 679123. DOI 10.3389/fbioe.2021.679123. [Google Scholar] [CrossRef]
27. Zhang, H., Zhou, Z., Yang, Q. (2018). Match analyses of table tennis in China: A systematic review. Journal of Sports Sciences, 36(23), 2663–2674. DOI 10.1080/02640414.2018.1460050. [Google Scholar] [CrossRef]
28. Lam, W. K., Fan, J. X., Zheng, Y., Lee, W. C. C. (2019). Joint and plantar loading in table tennis topspin forehand with different footwork. European Journal of Sport Science, 19(4), 471–479. DOI 10.1080/17461391.2018.1534993. [Google Scholar] [CrossRef]
29. He, Y., Fekete, G. (2021). The effect of cryotherapy on balance recovery at different moments after lower extremity muscle fatigue. Physical Activity and Health, 5(1), 255–270. DOI 10.5334/paah.154. [Google Scholar] [CrossRef]
30. Bankosz, Z., Winiarski, S. (2018a). The evaluation of changes of angles in selected joints during topspin forehand in table tennis. Motor Control, 22(3), 314–337. [Google Scholar]
31. Bankosz, Z., Winiarski, S. (2018b). Correlations between angular velocities in selected joints and velocity of table tennis racket during topspin forehand and backhand. Journal of Sports Science and Medicine, 17(2), 330–338. [Google Scholar]
32. Fu, F., Zhang, Y., Shao, S., Ren, J., Lake, M. et al. (2016). Comparison of center of pressure trajectory characteristics in table tennis during topspin forehand loop between superior and intermediate players. International Journal of Sports Science & Coaching, 11(4), 559–565. DOI 10.1177/1747954116654778. [Google Scholar] [CrossRef]
33. Wang, M., Fu, L., Gu, Y., Mei, Q., Fu, F. et al. (2018). Comparative study of kinematics and muscle activity between elite and amateur table tennis players during topspin loop against backspin movements. Journal of Human Kinetics, 64(1), 25–33. DOI 10.1515/hukin-2017-0182. [Google Scholar] [CrossRef]
34. Le Mansec, Y., Dorel, S., Hug, F., Jubeau, M. (2018). Lower limb muscle activity during table tennis strokes. Sports Biomechanics, 17(4), 442–452. [Google Scholar]
35. Ireland, A., Maden-Wilkinson, T., McPhee, J., Cooke, K., Narici, M. et al. (2013). Upper limb muscle bone asymmetries and bone adaptation in elite youth tennis players. Medicine & Science in Sports & Exercise, 45(9), 1749–1758. DOI 10.1249/MSS.0b013e31828f882f. [Google Scholar] [CrossRef]
36. Seeley, M. K., Funk, M. D., Denning, W. M., Hager, R. L., Hopkins, J. T. (2011). Tennis forehand kinematics change as post-impact ball speed is altered. Sports Biomechanics, 10(4), 415–426. DOI 10.1080/14763141.2011.629305. [Google Scholar] [CrossRef]
37. Rajabi, R., Johnson, G. M., Alizadeh, M. H., Meghdadi, N. (2012). Radiographic knee osteoarthritis in ex-elite table tennis players. BMC Musculoskeletal Disorders, 13(1), 1–16. DOI 10.1186/1471-2474-13-12. [Google Scholar] [CrossRef]
38. Fong, D. T., Hong, Y., Shima, Y., Krosshaug, T., Yung, P. S. et al. (2009). Biomechanics of supination ankle sprain: A case report of an accidental injury event in the laboratory. American Journal of Sports Medicine, 37(4), 822–827. DOI 10.1177/0363546508328102. [Google Scholar] [CrossRef]
39. Xu, D., Quan, W., Zhou, H., Sun, D., Baker, J. S. et al. (2022). Explaining the differences of gait patterns between high and low-mileage runners with machine learning. Scientific Reports, 12(1), 2981. DOI 10.1038/s41598-022-07054-1. [Google Scholar] [CrossRef]
40. Lin, H. T., Kuo, W. C., Chen, Y., Lo, T. Y., Li, Y. I. et al. (2022). Effects of fatigue in lower back muscles on basketball jump shots and landings. Physical Activity and Health, 6(1), 273–286. DOI 10.5334/paah.199. [Google Scholar] [CrossRef]
Cite This Article
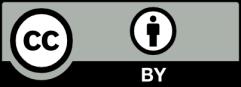