Open Access
ARTICLE
Evaluation of Mechanical Properties and Surface Quality of Wood from Bosnia and Herzegovina Exposed to Outdoor Conditions
1 Department of Wood Science and Technology, Faculty of Technical Engineering, University of Bihać, Bihać, 77000, Bosnia and Herzegovina
2 Department of Wood and Furniture Industry Engineering, Faculty of Resources and Earth Science, Shahrekord University, Shahrekord, 64165478, Iran
3 Department of Botany, Institute of Science, Banaras Hindu University, Varanasi, Uttar Pradesh, 221005, India
4 Biotechnical Faculty, Department of Wood Science, University of Ljubljana, Ljubljana, 1501, Slovenia
* Corresponding Authors: Redžo Hasanagić. Email: ; Mohsen Bahmani. Email:
Journal of Renewable Materials 2024, 12(8), 1417-1431. https://doi.org/10.32604/jrm.2024.052826
Received 16 April 2024; Accepted 19 June 2024; Issue published 06 September 2024
Abstract
This study investigated the mechanical properties of beech (Fagus sylvatica L.) and fir (Abies alba) wood from Bosnia and Herzegovina under outdoor exposure. Samples were exposed for 3-month exposure to assess bending strength, color changes, and surface quality. Results showed outdoor exposure negatively affected mechanical properties, particularly in samples with extended finger joints, causing significant surface cracks in uncoated samples. Beech wood exhibited notable color changes under exposure, with approximately 50% darkening without coating compared to 25% under covered conditions. Coated samples displayed minimal color changes, affirming the efficacy of surface treatment. Fir wood exhibited a roughness of 8.264 μm, while beechwood average roughness increased from 6.767 to 13.916 μm after exposure, with micro-pore development affecting water performance. Microscopic analysis identified prevalent fungal colonies, including Penicillium, Aureobasidium, Sclerophoma, and Chaetomium, underscoring their role in organic matter decomposition. This study highlights the importance of wood exposure and treatment selection for various applications.Graphic Abstract
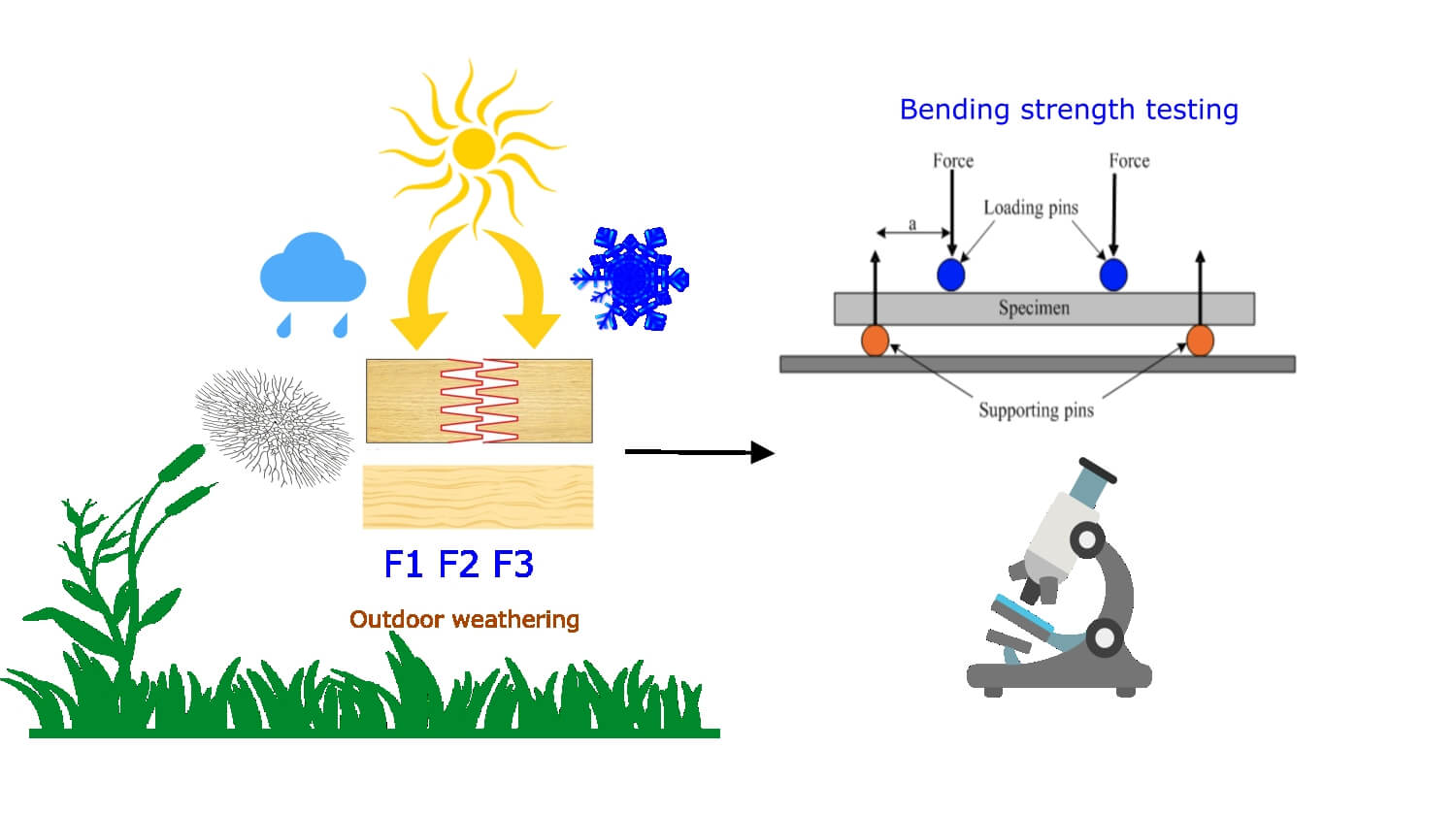
Keywords
Wood, a timeless and renewable material used by humans for centuries, exhibits exceptional adaptability across various applications, from furniture to construction. Despite this, its susceptibility to organisms that degrade wood, such as fungi and insects, has traditionally limited the outdoor applicability of wood with insufficient durability [1]. The integration of wood composites is indispensable in modern construction and building methods, presenting a sustainable alternative to traditional materials like steel and concrete in various construction applications [2].
Adhesive bonding, a technique employed for centuries, underwent significant advancement in the 20th century, becoming pivotal in nearly all branches of production [3]. The bonding process, involving surface preparation and adhesive application, facilitates the creation of strong joints between different materials [4]. Furthermore, research into novel ways of utilizing waste and recovered wood and optimizing resources is crucial for sustainable construction, with innovations like glued laminated timber gaining popularity in structural applications [5–9]. Numerous authors have investigated various aspects of finger joint strength through experimental research, mathematical modeling, and numerical simulation to enhance process efficiency and predictability. The strength of finger joint bonding depends on critical parameters, including height, length of the tooth, tip depth, and slope, as elucidated in research works [10].
Researchers discovered that 10 mm-long teeth and class D3 PVAc adhesives showed the highest strength when assessing bonding effects on resistance classes D1, D2, and D3 according to standard EN 204:2001 [11–14]. Engineered wood products (EWPs) have gained prominence over traditional timber due to their superior span capabilities and performance consistency. The finger joint method, a crucial step in EWP production, enhances structural integrity by interlocking timber boards. Flexural tests determine the load-bearing capacity of finger joints, ensuring quality in EWP manufacturing [15–18]. Investigations into the impact of ambient climates on beech glued laminated timber (glulam) revealed variations in moisture content and finger-joint delamination. Additionally, research explored cyclic temperature effects on the bending properties of glued laminated timber from different wood species. Studies also evaluated methanol’s influence on the stability and adhesion performance of PRF resin adhesives [19–23]. Other studies analyzed fingertip surface impact on bending strength and finger parameters on tensile strength [24–27], examined various wooden joints for mechanical, physical, or chemical properties, and compared experimental findings with ANSYS software outcomes [28]. Further research investigated lap joint strength in oriental beech samples bonded with different adhesives [29] and utilized laminated pine wood elements to assess strength under specific conditions. In research [30], laminated elements of pine wood with an average density of 575 kg/m3 and a moisture content of 8.9% were used, and the results showed that samples with reinforced edge inserts had improved bending strength of 90.3 N/mm2.
An investigation [31] into the influence of geometric parameters of the toothed joint profile on the tensile strength of individual bonded wooden panels demonstrated that the group of samples with the shortest teeth had the highest tensile strength. In contrast, the group with the longest teeth resulted in the lowest tensile strength. The study [32] of angular joints with beech wedge teeth in chair products showed that teeth measuring 7.5 mm exhibited greater durability under dynamic loads and greater stiffness at certain moments compared to 10 mm teeth. Research [33] on the strength of bonded toothed joints on fir wood samples demonstrated compliance with quality standards.
This research aims to present the results of experimental testing conducted on solid wood and wood joined by finger joints manufactured from various species, including fir and common beech. Special emphasis is placed on the mechanical properties of polyvinyl acetate (D4) adhesive joints, commonly used for connections exposed to outdoor conditions susceptible to the influence of biological and abiotic degradation factors, particularly fungi, color alteration, and surface roughness.
This research involves experimentally examining the bending strength of wooden samples, color, surface change, and fungi colonies after exploitation. These samples were prepared by cutting them in the longitudinal direction to enable a detailed study of their mechanical properties. The experimental segment encompasses several steps, including sample preparation, conducting the tests, analyzing the results, and drawing conclusions. Twenty samples without visible defects, a full cross-section and white finger joints, and free from knots and resin pockets were prepared for each test. The primary aim of this research lies in investigating the mechanical behavior of solid wood and wood joined by a finger joint under conditions of external exposure, partial external (covered) exposure, and laboratory conditions. Such an analysis allows for a detailed understanding of the behavior of these materials under various conditions, providing valuable insights into their stability and durability in different environments.
In this study, Rectangular defect-free samples of dimensions (length, width, height) 380 × 20 × 20 (mm3) as Fig. 1, made according to the BAS EN 408 + A1 standard [34] for bending strength testing in four-point bending were used. For bonding along the length of the samples, a finger joint with a mini finger length of 9 mm was used, with an average moisture content ranging from 7%–8%. Bostik polyvinyl acetate (PVAC) glue of D4 category, certified for water resistance as D4 BS-EN-204-D4 [35], with a bonding time of 7 min, was used as the adhesive. For the bonding of finger-jointed wooden specimens, a press manufactured by WEINIG, model ProfiPress L II, was used at standard room conditions (temperature of 20°C–25°C and relative humidity of 40%–60%), a pressure of 0.7 MPa, and a pressing time of 20 min to achieve optimal bond strength.
Figure 1: Wooden samples joined by a 9 mm-long mini-finger joint
The wooden samples used in the experiment were prepared by the company Malak Janj from Donji Vakuf, a specialized company in manufacturing solid panels made from segments connected by a longitudinal and transverse finger joints.
The adhesive utilized was Bostik Polyvinyl Acetate (PVAC) D4 grade, certified for water resistance according to BS-EN-204-D4 [35] standards. Polyvinyl Acetate (PVAC) cold wood glue of D4 grade is a dispersion adhesive based on polyvinyl acetate, designed for bonding various types of wood. Applying PVAC D4 wood adhesive was performed bilaterally and mechanically using standard tools within an optimal processing temperature range of 18°C to 20°C and an ideal wood moisture content for bonding of 7% to 8%. The adhesive was evenly applied to contact surfaces at a rate of 150–200 g/m2. Joined samples were then subjected to pressure in a parts bonding press for a duration of 7 min. Typical application areas for Bostik PVAC D4 adhesive include kitchen, bathroom, and garden furniture, windows, doors, fences, fiberboards, plywood, and similar products.
In our research, we utilized the “Jubin” varnish with wax formulated on the basis of water dispersion to protect wooden samples in outdoor conditions. This coating was chosen for its exceptional effectiveness and durability, proving to be a reliable option for safeguarding wood from adverse weather conditions. The application quantity amounted to 145 g/m2 of the wooden sample’s surface area.
Our investigation entailed a three-month exposure of wood specimens in Cazin, Bosnia and Herzegovina (44.966°N 15.942°E, altitude ranging from 200 to 400 m), commencing on 01 March, 2023. Samples were tested under laboratory conditions (exposure factor-F1) and outdoor conditions (exposure factors F2 and F3), where a portion of the samples underwent treatment for outdoor use and were exposed to outdoor influences (exposure factor 2, samples exposed outdoors but sheltered-F2, and exposure factor F3, samples exposed outdoors without shelter and above ground level-F3). Samples exposed to outdoor conditions F2 and F3 were placed on a metal structure 0.5 m above the ground, as defined by EN 335 (CEN, 2013) [36]. The primary objective of employing these exposed specimens was to conduct a comprehensive evaluation of the mechanical properties of wood subsequent to a three-month exposure to outdoor conditions. This encompassed the identification of surface irregularities (e.g., cracks, discoloration) and fungal infestation attributable to atmospheric influences.
The density of wood samples was determined by precisely measuring their mass and volume following the EN 384 [37] standard. Samples, sized consistently at 50 × 20 × 20 millimeters, were prepared and weighed using a digital scale with a minimum accuracy of 0.1 grams. Volume was determined using a micrometer to measure sample dimensions. Density was calculated by dividing mass by volume, yielding results in grams per cubic centimeter (g/cm3), providing quantitative insight into this vital wood parameter. This method ensures a comprehensive analysis of wood density, crucial for understanding its structural properties and diverse applications. The volume of the samples is determined by measuring their dimensions using a micrometer and applying the following equation:
where:
m = Sample mass in grams (g)
V = The sample volume in cubic centimeters (g/cm3)
2.6 Method for Determining the Moisture Content in Wood Samples
The wood samples, measuring 50 × 20 × 20 mm3, were meticulously prepared and weighed on a digital scale with an accuracy of 0.1 grams, recording the precise mass of each sample. Following this initial measurement, the samples were subjected to drying at a temperature of 103°C ± 2°C following ASTM D2016 [38] standards for a period of 24 h. Upon completion of the drying process, the samples were re-weighed to record their final mass. To calculate the moisture content in the wood, the difference between the initial and final mass of the wood sample was used, applying the following Eq. (2):
where:
MC = Moisture Content (%)
W1 = Initial sample mass (g)
W2 = Final sample mass (g)
To assess the mechanical properties of the samples pre and post-exposure, bending strength was determined in accordance with BAS EN 408 + A1 (CEN 2010) [34]. Prior to testing, the samples were conditioned under standard laboratory climate conditions: temperature (T) of 20°C and relative humidity of 65%. The samples, with a minimum length of approximately 19 times the cross-sectional thickness, were subjected to simple support and symmetric loading through 2-point bending, spanning about 18 times the thickness. Four-point bending samples measured 380 × 20 × 20 mm3 in dimension. Mechanical testing was conducted using a SHIMADZU (Kyoto, Japan) shredder type SIL-50KNAG apparatus at the Faculty of Technical Engineering in Bihać. Each material underwent twenty replicate tests. The samples were subjected to incremental bending under a pressure rate of 2 mm/min until the point of fracture.
The test samples underwent scanning and processing utilizing Corel Photo-Paint 8 software. Corel Photo-Paint was employed for color analysis, chosen for its ability to provide color data for the entire surface rather than isolated spots, thereby ensuring more comprehensive measurements. This method has demonstrated reliability, as corroborated by comparative analysis [39].
Color measurements were conducted on samples exposed to external weather conditions over a three-month period using the CIE Lab system, developed by the International Commission on Illumination (CIE) for colorimetry [40]. This system comprises three parameters: L*, a*, and b*. The L* axis signifies lightness, ranging from zero (black) to one hundred (white), serving as the achromatic axis for gray tones, while a* and b* denote the chromaticity coordinates.
The color difference (∆E) from a reference color (L0, a0, b0) to a target color (L1, a1, b1) in CIE Lab space is determined by calculating the Euclidean distance between the two colors. This computation is expressed by the following equation (Eq. (3)):
By summing (Σ ΔE) the obtained results of the analysed points (Eq. (4)), the course of the colour changes can be determined:
2.9 Advanced Surface Morphology Analysis of Wood Samples Utilizing Laser Scanning Confocal Microscopy
The surface morphology of the samples under investigation was meticulously characterized by utilizing an Olympus LEXT OLS5000 laser scanning confocal micro-scope, which is headquartered in Tokyo, Japan. The microscope offered precise insights into surface features by capturing 3D images of the longitudinal surfaces at a magnification of 50× over an area measuring 0.26 × 0.26 mm2 [41]. Measurement locations were systematically chosen at random to ensure comprehensive coverage. For each material combination, five measurements were recorded across two randomly selected samples, ensuring robustness in data collection.
Subsequent analysis of surface roughness was facilitated through the sophisticated microscope software included in the LEXT OLS5000 package. While several parameters were computed, particular emphasis was placed on Sa, acknowledged as the most representative and widely utilized metric for assessing surface roughness. Sa quantifies the deviation in point height from the arithmetic mean of the surface, providing a concise representation of surface texture.
Statistical scrutiny of the gathered data was conducted using industry-standard software suites, including Microsoft Excel (Microsoft, 2019, Redmond, Washington, USA) and GraphPad Prism (GraphPad Software, 9.0, San Diego, CA, USA), ensuring rigorous analysis and interpretation of results.
3.1 Mechanical Properties Analysis
As previously mentioned, the research conducted in this study provides detailed insights into the changes in the mechanical properties of wood under various meteorological conditions. The results clearly indicate that the resistance of different wood species to external factors depends on their material resistance to fungal degradation and their ability to absorb moisture. Over the course of several months of exposure, wood samples subjected to bending tests exhibited maximum force and fracture point. This study analysed the impact of wood joined with dovetail joints compared to solid fir and beech wood. These samples were exposed to the influence of three factors (F1, F2, and F3). The results of these investigations for exposure factor F1 are systematically presented in Table 1. This analysis meticulously documents the test results at four key points for exposure factors F1–F3, providing a basis for a deeper understanding of the influence of external factors on the mechanical properties of the tested wood species.
Analyzing the data for firewood, the mean maximum force was notably higher in the sample group (F1) exposed to outdoor conditions, as anticipated, registering at 1783.39 N. Conversely, the mean maximum force values in sample groups (F2) at 1043.56 N and (F3) at 1054.27 N showed more consistency but lower values than group (F1), Fig. 2. These findings imply that external factors diminish the maximum force values in groups (F2 and F3) compared to group (F1), despite only a 3-month exposure period. As expected, the highest mean values of the maximum force were recorded in samples of solid beech (B), with a mean value of 2479.36 N. The mean value for beech samples with surface protection (B-W) is slightly lower, at 2258.50 N.
Figure 2: The results of maximum bending force under various exposure factors
Fir wood (J) achieved slightly lower values of maximum force, with an average of 1186.82 N, while samples of fir with surface protection (J-W) yielded better results with an average of 1156.95 N. This is noteworthy because samples (J-W) were exposed to external conditions (F2 and F3).
The samples of beech with extended toothed joints (B-FJ) exhibited an average maximum force of 670.19 N. In comparison, samples with surface protection (BFJ-W) had a slightly lower average maximum force of 420.87 N. Fir samples with extended toothed joints achieved a mean maximum force of 655.84 N. In contrast, fir samples with surface protection achieved an average of 453.19 N.
The highest mean values of the maximum force were observed in samples of solid beech (B) and beech with surface protection (B-W) under all exposure conditions (F1, F2, and F3), attributed to the undamaged wood structure (fibers), resulting in lower mean maximum force values for samples (B-FJ and BFJ-W). Improvements in the finger joint could lead to better results.
Comparing fir (J) samples with beech (B) samples, lower values of maximum force were recorded, which can be attributed to the lower density of fir wood. Solid fir samples (J) showed similar results to fir samples with surface protection (J-W), with minor differences, especially under exposure conditions (F2 and F3). Fir samples with extended finger joints (J-FJ) and samples with surface protection (JFJ-W) had lower maximum force values attributed to the interrupted wood structure.
Solid wood samples (B, J, BW, and JW) demonstrate superior performance compared to finger joint samples under various external exposure conditions (F1, F2, and F3). Solid wood samples exhibited higher modulus of elasticity values, indicating better resistance to environmental changes, particularly moisture content variations (1,2). In contrast, finger joint samples consistently showed lower modulus of elasticity values across all exposure conditions. The elongation caused by finger joints significantly diminishes their resistance, leading to reduced displacement and weaker stability under load (1). These findings align with previous studies that have investigated the impact of moisture content on the mechanical properties of wood. Wood density, which is directly related to the modulus of elasticity, is known to decrease with increasing moisture content (2). The presence of finger joints in wooden structures can exacerbate this effect, as the joints are more susceptible to moisture-induced changes (3). Solid wood structures, like beech and fir, exhibit more consistent resistance to environmental changes compared to extended finger joints (4).
3.2 Colour Changes of the Weathered Samples
The conducted color measurements on larger-sized samples that were exposed to external influences. It is well-known that wood color changes due to various factors. Wood exposed to light and ultraviolet rays darkens or yellows, which is particularly noticeable in light-colored wood species. Weather conditions such as rain and snow also have a significant impact. Over time, wooden structures, fences, facades, playgrounds, and other outdoor wooden products acquire a characteristic grey color. Knowing that wood changes color outdoors, some samples were previously coated with a coating in the test. The question is how the added pigments affect color changes due to weather influences. By employing the CIE Lab* method, the color change (∆E) was determined after a specific time interval of exposure.
In Table 2, it is noted that the average (∆E) value decreased during the measurements. The most significant color changes are observed in beech wood, where the beech samples were exposed without coating to external conditions F2 and F3. Additionally, noticeable changes in values can be observed in the samples of fir and beech wood treated with wood stain. Samples treated with the coating exhibit the least color changes. Thus, the desired effect of the prior surface treatment with coating has been achieved. Fir wood samples record minimal differences in ∆E values according to Table 2. Although both control samples and those treated with coating achieved the lowest average values for ∆E, significant differences among the coating-treated samples were not observed. Table 2 shows that samples untreated with the coating but exposed to above-ground external conditions (F3) showed darkening by approximately 50% after 3 months of exposure compared to samples not exposed. Conversely, significant changes were not noticed in samples exposed to external conditions but under covered circumstances (F2), where the changes amounted to approximately 25%. Our results are in line with previous studies that investigated the color change of wood. Hrčková et al. [42] examined the functionality of the wood color measurement method, where assessments were conducted in the CIE Lab* color space. The measured values confirmed a decrease in the lightness of the wood.
3.3 Characteristics of Wood Surface Roughness
Roughness denotes the presence of fine irregularities on surfaces treated by machinery. These irregularities, arising from wood processing and anatomical structural features, can be quantified by measuring the height, width, and shape of peaks and valleys. Nonetheless, since all samples were processed using identical technical equipment, disparities observed in these tests are likely attributable to the inherent characteristics of the wood. Comparison of roughness across various control surfaces (Table 3) reveals a value of 6.767 μm for beech. In contrast, a higher roughness of 8.264 μm was noted for fir wood, indicative of the anatomical distinctions between the two wood species. Beech, characterized by a highly anatomically homogeneous structure, belongs to the category of diffuse-porous hardwoods, while fir represents a typical coniferous species [43].
The parameter Sa is influenced by cell size, highlighting the complexities of wood anatomy in contributing to surface roughness. Notably, the roughness of beechwood samples surged from 6.767 to 13.916 μm after three months of exposure in the second exposure factor (F2) without protective wood stain. Similarly, Sa in beech samples subjected to exposure factor (F3) escalated to 14.666 μm. These observations align with prior findings documented in the study [44]. Importantly, naturally exposed samples encountered a spectrum of biotic and abiotic factors, fostering the development of micropores that impact roughness and water performance [44,45].
The coating, characterized by its formation of a thin protective layer on material surfaces, offers resistance against abrasion, UV radiation, moisture, and other external influences. Typically formulated with transparent pigments and binders, the coating penetrates the wood, preserving its natural appearance while safeguarding it from environmental stressors. Given its protective attributes, wood stain serves to impede moisture infiltration, thus mitigating roughness fluctuations induced by climatic variability. Moreover, wood stain contributes to surface preservation, shielding against damage inflicted by sunlight, UV radiation, and other external factors. Consequently, the presence and properties of wood stain play a pivotal role in shielding surfaces from external influences, thereby maintaining relatively stable roughness levels during exposure to environmental conditions. The darkening of wood color results from two processes. Firstly, weather conditions cause the degradation of lignin and the subsequent leaching of degradation products from the wood, resulting in a silvery hue. Secondly, darker coloration is also associated with melanin pigments (as seen in CLSM Roughness images) secreted by blue stain and sapstain fungi. However, less noticeable changes were observed in wood protected by wood stain.
The main reason for this is that coated wood is already darker, so the blue staining does not cause as significant a change in the L* component as noted for unmodified species. After twelve months of exposure, one of the main changes observed is the degradation of lignin on the surface and the leaching of quinones after UV radiation. Visual changes, such as color alteration and cracking, of samples before and after weathering can be seen in Fig. 3.
Figure 3: Surfaces of samples, dimensions (2000 μm × 2000 μm) generated by LEXT 3D measurement laser microscope OLS5000 before exposure and after 3 months of exposure outdoors
The results indicate that exposure to sunlight and precipitation leads to reltively rapid color change towards silverygray. It has also been stated that color change is a function of two processes: lignin degradation and associated leaching of degradation products from wood, as well as melanin pigments secreted by blue stain and sapstain fungi. Additionally, colonies of blue stain fungi or weathering conditions, which contribute to increased roughness due to the appearance of loose fibers, also play a role. However, as the wood was analyzed at higher magnifications, larger cracks did not affect roughness parameters.
Based on microscopic analysis (Table 4), the predominant fungal colonies identified on the wood samples belong to the genus Penicillium, Aureobasicium, Sclerophoma and Chaetomium. This genus, comprising ascomycetous fungi, holds widespread distribution in natural habitats and plays a pivotal role in organic matter decomposition. Typically thriving in environments characterized by elevated relative humidity, these fungi indicate moisture-rich conditions conducive to their proliferation.
The wood in the analyzed samples retained its structure, as confirmed by detailed microscopic and mechanical analysis. Despite exposure to external conditions over three months, degradation primarily manifested only on the surface layer of the wood, evident through color changes on the exterior of samples exposed to atmospheric conditions. Lignin, responsible for the darker color of wood, degrades under the influence of UV radiation, while cellulose fibers remain on the surface, imparting a silvergray hue to the wood [46]. Analysis of samples that were coated did not show the presence of blue coloration, implying that the presence of the coating prevents the growth of blue spots caused by fungi on the wood surface. Coatings, acting as protective layers, provide resistance to moisture, UV radiation, and other atmospheric factors that could lead to wood deterioration [47].
The study emphasizes the critical importance of joint quality through evaluations of the bending strength of beech and fir wood, with and without finger joints, under various exposure factors. Compliance with BAS EN 408 standards facilitated the assessment of crucial physical and mechanical properties for joint quality determination. Key conclusions from the findings include the variation in bending strength and modulus of elasticity observed in samples of beech and fir wood, both with and without finger joints. Laboratory conditions (F1) positively impacted bending strength, while the modulus of elasticity experienced a reduction compared to exploitation conditions due to moisture content alterations. External conditions (F2 and F3) can influence the durability of adhesive joints and the structural stability of solid wood. It is crucial to emphasize the importance of using protective coatings to preserve the color and surface quality of wooden samples, recommending their application as a preventive measure against adverse external influences. Furthermore, careful selection of wood species according to intended use can be pivotal, while regular maintenance and inspection are essential for prolonging the lifespan of wooden materials and preventing more serious damage. Detailed analysis reveals the intricate interactions between external factors and material characteristics, highlighting the need for further research to achieve a comprehensive understanding. Continuous testing beyond adhesive certificates is necessary for accurately evaluating solid wood bonding strength. Coatings applied to wood surfaces form surface films, affecting liquid absorption without diminishing wood hygroscopicity. Color changes observed in untreated samples suggest inherent wood sensitivity to environmental factors such as UV radiation. Future research endeavors should prioritize expanding treatment analysis and exploring different adhesive types for finger joint bonding.
Acknowledgement: The authors acknowledge the financial support of the Slovenian Research Agency (ARRS) within Research Program P4-0015 (Wood and Lignocellulosic Composites), Ministry of Education, Science, Culture, and Sports of the Una-Sana Canton, Co-Financing of Scientific Research and Research and Development Projects of Special Interest to the Una-Sana Canton (03-02-2190-647/2023) Assessment of the Structural Integrity of Cultural Buildings in Bosnia and Herzegovina (Una-Sana Canton) Using Non-Destructive Testing Methods.
Funding Statement: The authors received no specific funding for this study.
Author Contributions: Conceptualization, Redžo Hasanagić; methodology, Miha Humar, Redžo Hasanagić; validation, Redžo Hasanagić, Mohsen Bahmani; formal analysis, Miha Humar, Redžo Hasanagić, Leila Fathi, Mohsen Bahmani, Pallavi Gautam; investigation, Redžo Hasanagić, Miha Humar, Leila Fathi, Pallavi Gautam; resources, Redžo Hasanagić, Umejr Šljivo, Miha Humar; data curation, Redžo Hasanagić, Mohsen Bahmani, Miha Humar; writing–original draft preparation, Redžo Hasanagić, Miha Humar, Mohsen Bahmani; writing–review and editing, Redžo Hasanagić, Miha Humar, Pallavi Gautam, Mohsen Bahmani, Leila Fathi, Umejr Šljivo; visualization, Redžo Hasanagić; su-pervision, Miha Humar; project administration, Redžo Hasanagić, Mohsen Bahmani; funding acquisition, Redžo Hasanagić. All authors reviewed the results and approved the final version of the manuscript.
Availability of Data and Materials: Data is available on request from the authors.
Conflicts of Interest: The authors declare that they have no conflicts of interest to report regarding the present study.
References
1. Ahmad Z, Lum WC, Lee SH, Razlan MA, Wan Mohamad WH. Mechanical properties of finger jointed beams fabricated from eight Malaysian hardwood species. Con Buil Mat. 2017;145(9):464–73. doi:10.1016/j.conbuildmat.2017.04.016. [Google Scholar] [CrossRef]
2. Morin-Bernard A, Blanchet P, Dagenais C, Achim A. Glued-laminated timber from northern hardwoods: effect of finger-joint profile on lamellae tensile strength. Con Buil Mat. 2021;271(1):121591. doi:10.1016/j.conbuildmat.2020.121591. [Google Scholar] [CrossRef]
3. De Silva S, Liyanage V. Suitability of finger jointed structural timber for construction. J Str Eng App Mech. 2019;2(3):131–42. doi:10.31462/jseam.2019.03131142. [Google Scholar] [CrossRef]
4. Hasanagić R, Hodžić A, Jurković M. Modelling and optimization of tensile break force of solid wood elements lengthened by finger joint. J Adhe Sci Tech. 2019;2019(1):1–15. doi:10.1080/01694243.2019.1690266. [Google Scholar] [CrossRef]
5. Faircloth A, Kumar C, McGavin R, Gilbert B, Leggate W. Mechanical performance and bond integrity of finger jointed high-density sub-tropical hardwoods for residential decking. Forests. 2023;14(5):956. doi:10.3390/f14050956. [Google Scholar] [CrossRef]
6. Abdul Hamid NH, Ahmad M, Suratman MN, Abu F. Bending Strength of finger jointed kelat wood (Syzygium spp.) as affected by finger length and orientation. Ad Mat Res. 2015;1134:138–42. doi:10.4028/www.scientific.net/AMR.1134.138. [Google Scholar] [CrossRef]
7. Pěnčík J. Tests of wooden specimens from scots pine (Pinus sylvestris) with the help of anisotropic plasticity material model. Drv Ind. 2015;66(1):27–33. doi:10.5552/drind.2015.1362. [Google Scholar] [CrossRef]
8. Šljivo U, Hasanagić R, Fathi L, Bahmani M. Comparative analysis of bending strength properties between glued and un-glued finger joints in beech and fir wood species. IOP Conf Series: Mater Sci Eng. 2023;1298(1):012011. doi:10.1088/1757-899X/1298/1/012011. [Google Scholar] [CrossRef]
9. Gomon SS, Gomon P, Homon S, Polishchuk M, Dovbenko T, Kulakovskyi L. Improving the strength of bending elements of glued wood. Pro Struc Int. 2022;36(1):217–22. doi:10.1016/j.prostr.2022.01.027. [Google Scholar] [CrossRef]
10. Vassiliou V, Barboutis I, Karastergiou S. Effect of PVAc bonding on finger-joint strength of steamed and unsteamed beech wood (Fagus sylvatica). J App Polyr Sci. 2006;103(3):1664–9. doi:10.1002/app.25079. [Google Scholar] [CrossRef]
11. Bašić N, Kulenović E, Hozdić E. Glue laminated elements for Wood joinery (In Hrvatski). Available from: https://hrcak.srce.hr/clanak/184409. [Accessed 2014]. [Google Scholar]
12. Klippel M, Frangi A, Hugi E. Experimental analysis of the fire behavior of finger-jointed timber members. J Str Eng. 2014;140(3):435. doi:10.1061/(ASCE)ST.1943-541X.0000851. [Google Scholar] [CrossRef]
13. Konnerth J, Valla A, Gindl W, Müller U. Measurement of strain distribution in timber finger joints. W Sci Tech. 2006;40(8):631–6. doi:10.1007/s00226-006-0090-9. [Google Scholar] [CrossRef]
14. Kohan N, Via BK, Taylor S. A comparison of geometry effect on tensile testing of wood strands. F Pro J. 2012;62(3):167–70. doi:10.13073/0015-7473-62.3.167. [Google Scholar] [CrossRef]
15. Tang Z, Shan B, Li WG, Peng Q, Xiao Y. Structural behavior of glubam I-joists. Con Buil Mat. 2019;224(9):292–305. doi:10.1016/j.conbuildmat.2019.07.082. [Google Scholar] [CrossRef]
16. Vega A, Baño V, Cardoso A, Moya L. Experimental and numerical evaluation of the structural performance of Uruguayan Eucalyptus grandis finger-joint. H A Roh-Und Werkstoff. 2020;78(5):923–32. doi:10.1007/s00107-020-01570-5. [Google Scholar] [CrossRef]
17. Rescalvo FJ, Portela M, Cruz C, Bravo R, Lorenzana JA. Relationship between the acoustic emission and the strain field in finger joints of engineered wood products for construction. E Fail Analy. 2024;162(1):108411. doi:10.1016/j.engfailanal.2024.108411. [Google Scholar] [CrossRef]
18. Navaratnam S, Christopher PB, Ngo T, Le TV. Bending and shear performance of Australian Radiata pine cross-laminated timber. Con Buil Mat. 2020;232(4):117215. doi:10.1016/j.conbuildmat.2019.117215. [Google Scholar] [CrossRef]
19. Wibowo ES, Park BD. Controlling the stability and adhesion performance of cold-setting phenol-resorcinol–formaldehyde resin adhesives through methanol addition. I J Adh Adh. 2024;132:103717. doi:10.1016/j.ijadhadh.2024.103717. [Google Scholar] [CrossRef]
20. Kytka T, Gašparík M, Sahula L, Karami E, Teterin D, Das S, et al. Bending characteristics of glued laminated timber depending on the alternating effects of freezing and heating. Con Buil Mat. 2022;350(5):128916. doi:10.1016/j.conbuildmat.2022.128916. [Google Scholar] [CrossRef]
21. Stolze H, Schuh M, Kegel S, Fürkötter-Ziegenbein C, Brischke C, Militz H. Monitoring of beech glued laminated timber and delamination resistance of beech finger-joints in varying ambient climates. Forests. 2021;12(12):1672. doi:10.3390/f12121672. [Google Scholar] [CrossRef]
22. Ibrisevic A, Obucina M, Hajdarevic S, Mihulja G. Influence of moisture content on the strength of finger joints bonded beech wood. In: International DAAAM Symposium; 2021; Vienna, Austria. p. 270–4. doi:10.2507/32nd.daaam.proceedings.038. [Google Scholar] [CrossRef]
23. Liu J, Yue K, Wang F, Wu J, Tang Z, Chen Z, et al. Effects of moisture content on lap-shear, bending, and tensile strength of lap-jointed and finger-jointed southern pine using phenol resorcinol formaldehyde and melamine urea formaldehyde. BioResources. 2020;15(2):3534–44. doi:10.15376/biores.15.2.3534-3544. [Google Scholar] [CrossRef]
24. Lara-Bocanegra AJ, Majano-Majano A, Crespo J, Guaita M. Finger-jointed Eucalyptus globulus with 1C-PUR adhesive for high performance engineered laminated products. Con Buil Mat. 2017;135(5):529–37. doi:10.1016/j.conbuildmat.2017.01.004. [Google Scholar] [CrossRef]
25. Hemmasi AH, Khademi-Eslam H, Roohnia M, Bazyar B, Yavari A. Elastic properties of oak wood finger joints with polyvinyl acetate and isocyanate adhesives. BioResources. 2013;9(1). doi:10.15376/biores.9.1.849-860. [Google Scholar] [CrossRef]
26. Amoah M, Kwarteng FO, Dadzie PK. Bending properties and joint efficiency of some tropical hardwoods finger-jointed in green and dry states. J Ind Aca W Sci. 2014;11(1):57–64. doi:10.1007/s13196-014-0118-y. [Google Scholar] [CrossRef]
27. Lovrić Vranković J, Boko I, Divić V. Experimental testing and numerical modelling of glued laminated timber. In: Common Foundations 2018-uniSTem: 6th Congress of Young Researchers in the Field of Civil Engineering and Related Sciences; 2018; Split: University in Split. doi:10.31534/co/zt.2018.19. [Google Scholar] [CrossRef]
28. Gao Y, Wu Y, Zhu X, Zhu L, Yu Z, Wu Y. Numerical analysis of the bending properties of cathay poplar glulam. Materials. 2015;8(10):7059–73. doi:10.3390/ma8105362. [Google Scholar] [PubMed] [CrossRef]
29. Derkowski A, Kuliński M, Trociński A, Krzosek S, Mirski R. Selected mechanical properties of glue-laminated timber produced from locally repaired timber. Materials. 2022;15(22):8112. doi:10.3390/ma15228112. [Google Scholar] [PubMed] [CrossRef]
30. Rao S, Gong M, Chui YH, Mohammad M. Effect of end pressure on performance of structural finger-joined lumber fabricated using a short joint profile. Eu J W W Prod. 2013;72(1):143–5. doi:10.1007/s00107-013-0756-6. [Google Scholar] [CrossRef]
31. Hou J, Taoum A, Kotlarewski N, Nolan G. Study on the effect of finger joints on the stiffness of fibre-managed E. nitens sawn boards. Buildings. 2022;12(12):2078. doi:10.3390/buildings12122078. [Google Scholar] [CrossRef]
32. Fulton L, Beauvais B, Brooks M, Kruse CS, Lee K. A publicly available cost simulation of sustainable construction options for residential houses. Sustainability. 2020;12(7):2873. doi:10.3390/su12072873. [Google Scholar] [CrossRef]
33. González-Prieto O, Casas Mirás JM, Torres LO. Finger-jointing of green Eucalyptus globulus L. wood with one-component polyurethane adhesives. Eu J W W Prod. 2021;80(2):429–37. doi:10.1007/s00107-021-01770-7. [Google Scholar] [CrossRef]
34. Bosnian Adaptation of the European Standard, BAS EN 408: 2011 (CEN 2010). Timber structures-structural timber and glued laminated timber-determination of some physical and mechanical properties; 2011. Available from: https://isbih.gov.ba/en/standard/240541. [Accessed 2024]. [Google Scholar]
35. British Adaptation of the European Standard. BS EN 204:2016. Classification of thermoplastic wood adhesives for non-structural applications; 2016. Available from: https://www.en-standard.eu/bs-en-204-2016-classification-of-thermoplastic-wood-adhesives-for-non-structural-applications/. [Accessed 2024]. [Google Scholar]
36. European Committee for Standardization (CEN, 2013EN 335. Durability of wood and wood-based products-use classes: definitions, application to solid wood and wood-based products; 2013. Available from: https://standards.iteh.ai/catalog/standards/cen/e5d368b1-2232-47e2-8349-ee85cb6c895b/en-335-2013. [Accessed 2023]. [Google Scholar]
37. European Committee for Standardization (CENEN 384. Structural timber-determination of characteristic values of mechanical properties and density for determining the density of wood; 2016. [Google Scholar]
38. American Standard Developed by ASTM International, Formerly Known as the American Society for Testing and Materials (ASTMASTM D2016. Standard test methods for moisture content of wood; 2013. Available from: https://global.ihs.com/doc_detail.cfm?document_name=ASTM%20D2016&item_s_key=00016225. [Accessed 2023]. [Google Scholar]
39. European Committee for Standardization (CEN, 2016EN 350:2016. Durability of wood and wood-based products-testing and classification of the durability to biological agents of wood and wood-based materials; 2016. Available from: https://standards.iteh.ai/catalog/standards/cen/b02d18a7-87ce-4a20-84c7-c0de641a2780/en-350-2016. [Accessed 2023]. [Google Scholar]
40. EN ISO 11664-4. Colorimetry–part 4: CIE 1976 L*a*b* colour space. Brussels: CEN; 2008. [Google Scholar]
41. Geometrical Product Specifications (GPSISO 25178. Geometrical product specifications (GPS)–surface texture: Area–part 2: terms, definitions and surface texture parameters. Geneva: International Organisation for Standardization; 2021. [Google Scholar]
42. Hrčková M, Koleda P, Koleda P. Measuring and analysis colour of birch wood in digital picture. Manag Syst Prod Eng. 2020;28(3):162–7. doi:10.2478/mspe-2020-0024. [Google Scholar] [CrossRef]
43. Csanády E, Magoss E, Tolvaj L. Surface roughness of wood. In: Quality of machined wood surfaces. Berlin/Heidelberg: Springer; 2015. p. 183–236. [Google Scholar]
44. Jirouš-Rajković V, Miklečić J. Enhancing weathering resistance of wood—a review. Poly. 2021;13(12):1980. doi:10.3390/polym13121980. [Google Scholar] [PubMed] [CrossRef]
45. Dong H, Hasanagić R, Fathi L, Bahmani M, Kržišnik D, Keržič E, et al. Selected mechanical and physical properties of thermally modified wood after field exposure tests. Forests. 2023;14(5):1006. doi:10.3390/f14051006. [Google Scholar] [CrossRef]
46. Brischke C, Thelandersson S. Modelling the outdoor performance of wood products—a review on existing approaches. Con Buil Mat. 2014;66(3):384–97. doi:10.1016/j.conbuildmat.2014.05.087. [Google Scholar] [CrossRef]
47. William S. ThermoWood® handbook. Available from: https://thermalwoodcanada.com/images/PDF/Thermowood_handbook.pdf. [Accessed 2023]. [Google Scholar]
Cite This Article
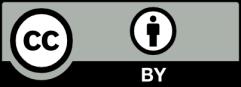
This work is licensed under a Creative Commons Attribution 4.0 International License , which permits unrestricted use, distribution, and reproduction in any medium, provided the original work is properly cited.