Open Access
ARTICLE
Starch Orodispersible Film Loaded with Melatonin for Human Supplementation
1 Department of Health and Biological Sciences, University of Araraquara (UNIARA), São Paulo, 14801-340, Brazil
2 Department of Materials Engineering, São Carlos School of Engineering, University of São Paulo, São Paulo, 13563-120, Brazil
* Corresponding Author: Eliane Trovatti. Email:
Journal of Renewable Materials 2024, 12(7), 1343-1354. https://doi.org/10.32604/jrm.2024.052288
Received 29 March 2024; Accepted 25 June 2024; Issue published 21 August 2024
Abstract
An innovative pharmaceutical form for administering melatonin, based on starch orodispersible film (ODF), was designed and prepared. The composition of the ODF included starch as the polymer matrix, the active drug melatonin, and a plasticizer. Melatonin, a natural hormone produced by the pineal gland in the brain, can be absorbed by passive diffusion across the mucous membrane, resulting in improved bioavailability when compared to conventional oral administration. This study shows a simple and efficient method for preparing melatonin-loaded orodispersible films with a physically stable and commercially viable matrix, suitable for use in the pharmaceutical industry. The films were prepared by treating the starch with microwave irradiation, followed by plasticization with glycerol, melatonin loading and drying by solvent casting. Mechanical tests showed the films’ robustness, with a modulus of approximately 97 MPa, indicating good handling properties. Moisture uptake analysis showed fast water absorption, reaching about 150% within 2 min, indicating its fast oral disintegration potential. Disintegration tests in artificial saliva and dissolution studies indicated the release of the drug in minutes, indicating the potential properties of the film for oral drug delivery. To the best of our knowledge, there is no melatonin ODF based on starch described in the literature.Keywords
Orodispersible films (ODF) are pharmaceutical forms based on a matrix that can dissolve in the oral mucosa without the need for water. They are often used to incorporate drugs for administration to patients who have difficulty swallowing pills or capsules. The preparation of ODFs and their use in the pharmaceutical industry is very new, with few approaches described in the literature. ODFs are usually composed of polymers and plasticizers and may also be flavored to improve acceptance by the patient [1–3]. The composition of an ODF varies according to the drug to be administered, as interactions can occur among the components, but generally include the polymeric matrix, the drug, and the plasticizer. The function of the polymer (e.g., maize starch) is to maintain the shape of the ODF and primarily serve as the matrix for the drug loading. The plasticizer is added to improve flexibility and ensure that the ODF does not become brittle or develop cracks during the manufacturing and handling process. The drug provides the desired medicinal therapeutic action [4–6].
Starch is a complex carbohydrate found in many vegetables, including potatoes, maize, rice, wheat, and cassava. It is composed of two main molecules: amylose (a linear molecule) and amylopectin (a branched molecule), both formed by glucose chains linked by glycosidic bonds. Starch is an important energy source for humans and is used in the manufacture of food products such as bread, pasta, cereals, and cakes. Starch is also used industrially in the manufacture of paper, fabrics, and adhesives [7–9]. Glycerol (C3H8O3) is a colorless, odorless, viscous liquid [10] widely used as an ODF plasticizer. This alcohol possesses three hydroxyl groups (−OH) that enable the molecule to interact, usually via hydrogen bonds, with natural polymer chains, such as the hydroxyl groups of starch. These weak bonds keep the glycerol within the polymer chains, plasticizing it. Hence, when added to the polymer, glycerol can improve flexibility, impact resistance, and durability. Furthermore, glycerol is a humectant that can attract and retain moisture, further helping to maintain the flexibility of the polymer.
The model drug selected in this work was melatonin, a molecule that occurs naturally in the human body and is produced by the pineal gland in the brain. Melatonin is synthesized from the amino acid tryptophan, with the neurotransmitter serotonin as an intermediate. Melatonin production is regulated by the circadian rhythm that governs waking and sleeping cycles. During the night, melatonin levels increase, leading to sleepiness, and during the day, melatonin levels decrease, so the body remains awake and alert. Melatonin also plays a role in regulating the immune system, cardiovascular, and reproductive functions. Furthermore, it is a powerful antioxidant that helps protect the body’s cells from damage by free radicals [11–13].
The biosynthesis of melatonin proceeds in two main steps. The first step occurs in the mitochondria, where tryptophan is converted to 5-hydroxytryptophan (5-HTP) by the tryptophan hydroxylase enzyme. In the second step, 5-HTP is converted to serotonin by the aromatic amino acid decarboxylase enzyme. Serotonin is then transported from serotonergic neurons to the pinealocytes of the pineal gland, where it is converted to melatonin by N-acetyltransferase and hydroxyindole-O-methyltransferase enzymes. Melatonin is secreted into the bloodstream and reaches different parts of the body, including the brain, acting to regulate circadian rhythms, sleep, mood, immune function, and other biological processes [14–17].
Melatonin supplementation is used to treat sleep disorders, regulate circadian rhythms in individuals with irregular work schedules or jet lag, and improve recovery in cases of myopathies associated with locomotion difficulty and muscle weakness. Additionally, melatonin has antioxidant, anti-inflammatory, and immunomodulatory effects that can be beneficial in conditions including neurodegenerative disorders, cardiovascular diseases, and diabetes [18–21]. The oral mucosa is one route for the administration of melatonin, where its absorption occurs by passive diffusion across the mucous membrane. Absorption is influenced by factors, including the concentration of melatonin in the product and the pH of the solution, with the compound being more bioavailable compared to conventional oral administration.
Sublingual administration of melatonin results in rapid absorption, with maximum plasma levels reached after 30 min [22–25]. Although oral absorption is very appropriate for melatonin administration, the most common pharmaceutical form is tablets. Few studies have described alternative pharmaceutical forms for melatonin administration, creating a demand in the pharmaceutical industry. Additionally, ODF technology is new, with studies starting to appear in the literature only in recent years. With this in mind, we propose to develop an innovative, simple, and efficient methodology for the production of a melatonin-based supplement suitable for absorption in the oral mucosa. The proposed starch-based ODF technology is not described in the literature, to the best of our knowledge, and represents an alternative pharmaceutical form that can potentially be more attractive for individuals with difficulty swallowing.
2.1 Preparation of the Orodispersible Film
Starch (10 g) was mixed with water (160 g) at room temperature and heated in a microwave oven (700 W) for 1 min. Glycerol (2 g, 20% by weight) was then blended with the heat-treated starch, and stirred for 30 s. The mixture was poured into a Petri dish and dried at 37°C for 48 h, forming the control orodispersible film (COF). For the melatonin-loaded film (MOF), melatonin (0.06 g) was dissolved in glycerol (2 g), and the film was prepared similarly. After drying, samples were cut for testing. Fig. 1 shows a schematic representation of the preparation process. Visual appearances were recorded using an Apple® iPhone 11 ProMAX® camera, and dimensions were measured in triplicate with a micrometer and pachymeter (Mitutoyo).
Figure 1: Schematic representation of the steps of the preparation of the control (COF) and melatonin-loaded (MOF) orodispersible films
2.2 Moisture Content Determination
The films, cut to dimensions of 25 mm × 25 mm × 0.1 mm, were kept at 37°C and 50% relative humidity in a climate-controlled room for 48 h, then weighed. Subsequently, they were dried at 100°C until a constant mass was achieved and weighed again. The measurements were repeated at times of 0, 30, 60, 90, 120, 150, and 180 min. The moisture content was determined as follows:
W0 = initial weight of the specimen; Wt = weight after time t.
The mechanical tests were conducted using an Instron 5969 Universal Testing Machine equipped with a 5 kN load cell at a deformation rate of 100 mm/s. The samples, measuring approximately 1 cm × 6 cm and with a thickness of around 100 µm, were conditioned at 25°C and 50% RH for 48 h before testing. Young’s modulus, tensile strength, and elongation were calculated from the curves. Young’s modulus was determined as the slope at low deformation. The tensile strength and elongation at break were calculated using Bluehill 3 software. Six specimens of each film (COF and MOF) were tested.
The COF and MOF samples were observed using optical microscopy to evaluate aspects including uniformity, presence of defects, bubbles or cracks, thickness uniformity, presence of distinct layers, presence of particles or granules, and the presence in the film of specific components (polymers and active agent).
Samples were cut in dimensions of 25 mm × 25 mm × 0.1 mm, weight and immersed into phosphate-buffered saline solution, pH 7.0, at 37°C. At the determined period of time, they were removed from the liquid; their surfaces were gently dried with paper and weighed. After that, each sample was immersed again in a phosphate-buffered saline solution. The measurements were taken at 0, 2, 5, 10, 20, 30, 45, and 60 min. The water absorption, Wabs, was calculated as follows:
where, W0 = initial weight of the material; Wt = weight after immersion for time t.
2.6 Disintegration of the Orodispersible Film in Artificial Saliva
The films (dimensions 25 mm × 25 mm × 0.1 mm) were immersed in 30 mL of artificial saliva at 37°C, without agitation, and weighed at 0, 5, 15, 30, 60, 120, and 240 min. The artificial saliva composition was 0.12% potassium chloride, 0.010% sodium chloride, 0.015% calcium citrate, 0.005% magnesium chloride, 3% sorbitol (corrected), 15% Natrosol gel, 0.1% Nipagin, and distilled water, and 200 mg of amylase. The mass loss was the parameter to determine the disintegration, using the following equation:
where, Wt = weight of the sample after immersion into the solvent; W0 = initial weight.
The melatonin release assay was performed to study the behavior of the orodispersible film, using a MOF sample (dimensions 25 mm × 25 mm × 0.1 mm) immersed in 30 mL of artificial saliva in a capped Becker cup, at 37°C. After immersing the sample, 2 mL of the solution was immediately removed with a micropipette and transferred to a test tube, labeled as time zero. Further 2 mL aliquots were removed at 5, 15, 30, 60, 120, and 240 min. The volume (2 mL) was replaced by artificial saliva after each sampling. The absorbance of the samples was measured by UV-visible spectroscopy, using a V-M5 VIS spectrophotometer (Novatécnica-BelPhotonics), at a wavelength of 250 nm, with the sample in a rectangular 10 mm optical path length quartz cuvette. The accumulated release (%) was calculated using a calibration curve plotted from the data of the measurement of melatonin solutions.
Statistical analysis was performed and the results were reported as the means ± SD. The data were analyzed using ANOVA for repeated measures.
The COF displayed high transparency, and the MOF had a shiny appearance, as shown in Fig. 2. Manipulation of the samples at different angles (Fig. 2B,C, for both COF and MOF) showed that there was no apparent difference between the materials in terms of malleability, which allow to handle them without damaging the structure. The images in Fig. 2D show the thicknesses of the materials.
Figure 2: Macrometric images of the COF (1) and MOF (2) films, showing respectively their surface (A1 and A2), their malleability (B1, B2, C1 and C2), and their thickness (D1 and D2)
3.2 Moisture Content Determination
The behavior of the COF and MOF films related to their loss of water is shown in Fig. 3. At 30 min, the mass loss values of both films could be attributed to starch dehydration. At 60 and 90 min, the mass loss rates decreased for both samples, as shown by the curve. The curves plateaus were reached at 120 and 150 min for the COF and MOF samples respectively with the mass losses maintained until the end of the experiment. The mass losses at the end of the experiment were 11.96% (±0.31%) for COF and 16.21% (±0.32%) for MOF.
Figure 3: Mass loss of the COF and MOF samples in the moisture content assay
Stress-strain curves, shown in Fig. 4, were used to determine Young’s modulus, tensile strength, and stress-strain data, provided in Table 1. Stress-strain curves displayed a linear elastic behavior at low strains (around 1%), which gradually changed to a plastic profile, as expected for plasticized starch derivatives [26,27]. The Young’s modulus values indicated greater resistance of MOF when compared to COF, resulting in its lower elongation at break. The statistic tests indicated p < 0.02 when comparing the COF and MOF modulus, and p < 0.00 for elongation at break. These low p values indicated a significant difference between these groups for both modulus and elongation at break. The tensile stress was similar for both samples, however, the great reproducibility among the samples of each group resulted in p < 0.3, indicating a statistical difference between the groups COF and MOF. The increase in modulus values can be attributed to the incorporation of melatonin into the starch matrix.
Figure 4: Stress-deformation curves for the COF and MOF films
As shown in Fig. 5A–D, the COF and MOF films showed a homogeneous overall appearance. The starch grains were completely disrupted, then, no whole grains were found in the materials. The homogenous appearance, with no phase separation, indicated the good plasticization of the starch. The surface of COF (Fig. 5A,C) was smoother than that of MOF (Fig. 5B,D), although both materials were free from cracks or fissures. No foreign particles or agglomerates were observed in the images. Both COF and MOF were homogeneous, without distinct layers or areas of different densities.
Figure 5: Morphology of the COF (A and C) and MOF (B and D) films surface, at 100 and 200 magnifications. Scale bar = 100 µm
3.5 Water Absorption Assays and Disintegration of the Orodispersible Film in Artificial Saliva
The behavior of COF and MOF related to their gain of water mass in the absorption tests is shown in Fig. 6. Rapid mass increases occurred within 2 min, as shown in Fig. 6A, which could be explained by the hydrophilicity of the starch, with a deceleration observed in the growth curves between 5 and 10 min. The swelling was maintained at 20 and 30 min, with plateaus reached at 45 min. The final measurements after 60 min showed that the samples presented complete stability in the swelling process, with mass gains of 60.41% (±0.51%) and 64.50% (±0.70%) for COF and MOF, respectively. The swelling of the starch was the result of increased mobility of the amylose and amylopectin molecules, which facilitated the incorporation of water. The water absorption enables film disintegration after swelling, a desired feature for orodispersible films.
Figure 6: Behaviors of the COF and MOF films in the water absorption assay (A) and the results of their disintegration in artificial saliva (B), showing COF and MOF before (b1 and b4) and after disintegration test, in its wet (b2 and b5) and dried (b3 and b6) states, respectively
Fig. 6B shows the images of COF (b1) and MOF (b4) used for the disintegration test in artificial saliva, the residual wet samples after 45 min in contact with water (b2 and b5) and the dried residual samples (b3 and b6), respectively. The samples were weighed before the experiment and after complete drying, showing that there was a mass loss of 92.8% for COF (initial and final masses of 0.30 and 0.02 g, respectively) and a mass loss of 82.3% for MOF (initial and final masses of 0.42 and 0.08 g, respectively).
Fig. 7 shows the concentrations of melatonin measured at 0, 5, 15, 30, 60 and 120 in the release test. The release of the drug started just at the first contact with the solution, reaching about 59% of the drug at 5 min time point. The maximum release was about 95% of the drug, reached at 60 min time point. After 120 min, the film started to disintegrate and release particles into the medium, which abruptly increased the absorbance values and did not allow the measurements after that time point because the high absorbance of the system resulting from the particles in suspension.
Figure 7: Accumulated release of melatonin (%) in solution
The burst release of the drug at the first minutes of the experiments is the result of the diffusion of the soluble fraction of melatonin in the medium, as soon as it contacts the artificial saliva. The release profile follows the exponential growth kinetics (fit curve), which confirms that the drug release is governed by diffusion, according to a Fickian model [28]. Fickian diffusion describes the high velocity of solvent diffusion to the interior of the matrix, leading to high drug dissolution and diffusion. Melatonin is very low soluble in water, the main component of saliva and, this feature, in the in vitro release test, leads to the profile of the curve shown in Fig. 7, resulting from the equilibrium of the soluble drug in the medium with the insoluble drug in the matrix. These in vitro test results could be associated with a fast release of melatonin when it contacts saliva, which, in an in vivo condition, under dynamic leaching and absorption of the drug by the oral mucosa in vitro, could increase the melatonin release kinetics, because the equilibrium shift from the high concentration (film) to the concentration (oral mucosa) compartments.
COF and MOF (Fig. 2) films were translucent, flexible, and can be handled easily without breaking. The visual appearance of MOF suggested its high homogeneity, indicating good drug dispersion into the matrix. The lower moisture content of the MOF film (Fig. 3) when compared to COF film can be attributed to the hydrophobic nature of melatonin. Similar results were reported for the orodispersible film loaded with diclofenac, which displayed a water content below 20%, in accordance with the criteria to minimize the water content, aiming to avoid microbial contamination [5].
The results of mechanical tests (Fig. 4) indicated that COF and MOF exhibited typical behavior of starch derivatives, as expected. MOF samples displayed about three times higher Young’s modulus than COF. This behavior results from the melatonin incorporation into the starch matrix, which, even at very low concentrations, has increased the elastic modulus at the low deformation region.
Orodispersible films based on solid lipid particles for the release of melatonin were also described in the literature [29], and showed a negative effect of the melatonin on the mechanical properties, generating a fragile film. This effect was also shown in films based on poly(methyl methacrylate) for the delivery of paracetamol [30]. In both cases, the film fragility increased, increasing the powder concentration. When compared to the literature [29,30], the mechanical performance of COF and MOF were higher, indicating that the method, the matrix-plasticizer system and the melatonin concentration led to the preparation of an improved ODF. Good mechanical performance is a crucial essential requirement for the development of orodispersible films for drug administration, since to be used in the mouth they must not cause discomfort or break when handled. Thus, these results indicated the potential of the films for use in the administration of melatonin. Thus, these results indicate the potential of MOF for use in melatonin delivery.
The results shown in Fig. 6A indicated rapid water absorption by COF and MOF films during the first 2 min of the experiment, and can be attributed to the starch’s nature, rich in hydroxyl groups, with high affinity for water. Water absorption was lower after around 5–10 min and stabilized after 20 min, with values of 60.41 and 64.4 wt% for COF and MOF, respectively. The water absorption of the starch film can be seen as the initial stage that leads it to disintegrate, an essential characteristic required for orodispersible films. Fig. 6B shows the COF and MOF films before and after the disintegration experiment. The contact with the artificial saliva initiated a process of swelling and expansion, resulting in the film becoming more flexible and fragile. The saliva then dissolved the polymers of the film matrix, resulting in the disintegration of the film into small pieces. In the body, this process of dissolution and disintegration would enable the release of the drug and its mixing with the saliva, facilitating its absorption and subsequent action.
The release assays showed the fast release of melatonin, about 60% released in the first 5 min. This result is in total agreement with the literature [28]. It should be noted that the use of artificial saliva in disintegration testing of orodispersible films is common in quality control laboratories, since the solution composition mimics the physical and chemical characteristics of human saliva. Hence, the results obtained in these tests are considered a good approximation of the processes that occur in the mouth of the patient during drug administration.
The advantages of ODF have been described in the literature in the last few years and show that it can be a promising pharmaceutical form to benefit the neonatal and pediatric populations with respect to the development of suitable oral dosage forms [31]. This work can contribute to the development of this research field aiming to decrease the time to reach the industry.
In general, it could be concluded from the results that degradation of the orodispersible film matrix led to total release of the melatonin. The results were considered satisfactory, since in addition to the enzymes present in saliva, administration of the formulation in humans would result in faster disintegration of the film, due to the mechanical forces applied within the oral structure.
The novelty of this work lies in the innovative, simple and efficient method of preparing melatonin-loaded orodispersible films. Control films (COF), consisting of starch plasticized with glycerol, were compared with films loaded with melatonin (MOF). The results showed high hydrophilicity, with around 70 wt% water absorption in 2.5 min, in line with the disintegration results in artificial saliva. The melatonin release profile showed potential for the intended application. The melatonin films exhibited improved mechanical properties, with a modulus of around 97 MPa, compared to 34 MPa for the control films, indicating suitability for handling and orodispersible administration. These findings suggest that orodispersible film is physically stable and commercially viable, improving drug development technologies and enhancing pharmaceutical quality, particularly for individuals unable to use conventional drug delivery methods.
Acknowledgement: The authors thanks Tatiane Araújo Soares for her contribution, namely the measurement of the absorbance of the samples in the drug release tests and for plotting the correspondent graphic.
Funding Statement: This research was funded by São Paulo Research Foundation, FAPESP (Research Project Funding 2017-18782-6).
Author Contributions: Conceptualization of research and supervision: Eliane Trovatti and Creusa Sayuri Tahara Amaral; Experimental development: Fabio Tamanini; Methodology verification: Antônio José Felix Carvalho; Writing—original draft preparation: Fabio Tamanini, Writing—original draft preparation: Tatiane Zucchini de Souza, Writing—review and editing: Eliane Trovatti and Antônio José Felix Carvalho. All authors participated in the discussion of the results. All authors reviewed the results and approved the final version of the manuscript.
Availability of Data and Materials: Not applicable.
Conflicts of Interest: The authors declare that they have no conflicts of interest to report regarding the present study.
References
1. Morath B, Sauer S, Zaradzki M, Wagner AH. Orodispersible films-recent developments and new applications in drug delivery and therapy. Biochem Pharmacol. 2022;200(3):115036. doi:10.1016/j.bcp.2022.115036. [Google Scholar] [PubMed] [CrossRef]
2. Ferlak J, Guzenda W, Osmałek T. Orodispersible films-current state of the art, limitations, advances and future perspectives. Pharmaceutics. 2023;15(2):361. doi:10.3390/pharmaceutics15020361. [Google Scholar] [PubMed] [CrossRef]
3. Nguyen KV, Dang TK, Vu LTD, Ha NT, Truong HD, Tran TH. Orodispersible film incorporating nanoparticulate loratadine for an enhanced oral bioavailability. J Pharm Invest. 2023;53(3):417–26. doi:10.1007/s40005-023-00613-2. [Google Scholar] [CrossRef]
4. Thongwong P, Wattanathorn J, Thukhammee W, Tiamkao S. The potential role of the novel orodispersible film from rice polymer loaded with silkworm pupae hydrolysate and the combined extract of holy basil and ginger for the management of stroke with stress. Biomaterials. 2023;299(1):122175. doi:10.1016/j.biomaterials.2023.122175. [Google Scholar] [PubMed] [CrossRef]
5. Khadra I, Obeid MA, Dunn C, Watts S, Halbert GW, Ford S, et al. Characterisation and optimisation of diclofenac sodium orodispersible thin film formulation. Int J Pharm. 2019;561(1):43–6. doi:10.1016/j.ijpharm.2019.01.064. [Google Scholar] [PubMed] [CrossRef]
6. Zhao ZL, Peng D, Liu X, Li XC, Lan LJ, Wu XH, et al. Formulation and characterization of tadalafil-loaded orodispersible films with enhanced dissolution rate and membrane permeability. J Drug Deliv Sci Tech. 2023;84(6):104535. doi:10.1016/j.jddst.2023.104535. [Google Scholar] [CrossRef]
7. Silva MJB, Alves FS, Queiroz RN, Queiroz NIF, Lago GVP, Pereira GVS, et al. Starch–a review of biopolymeric products and their derivations. Res Soc Develop. 2022;11(12):e280111234470. doi:10.33448/rsd-v11i12.34470. [Google Scholar] [CrossRef]
8. Malik MK, Bhatt P, Kumar T, Singh J, Kumar V, Faruk A, et al. Significance of chemically derivatized starch as drug carrier in developing novel drug delivery devices. Nat Prod J. 2023;13(6):40–53. doi:10.2174/2210315512666220819112334. [Google Scholar] [CrossRef]
9. Matheus JRV, Dalsasso RR, Rebelatto EA, Andrade KS, Andrade LM, Andrade CJ, et al. Biopolymers as green-based food packaging materials: a focus on modified and unmodified starch-based films. Compr Rev Food Sci Food Saf. 2023;22(2):1148–83. doi:10.1111/1541-4337.13107. [Google Scholar] [PubMed] [CrossRef]
10. Callam CS, Singer SJ, Lowary TL, Hadad CM. Computational analysis of the potential energy surfaces of glycerol in the gas and aqueous phases: effects of level of theory, basis set, and solvation on strongly intramolecularly hydrogen-bonded systems. J Am Chem Soc. 2001;123(47):11743–54. doi:10.1021/ja011785r. [Google Scholar] [PubMed] [CrossRef]
11. Santos IMP, Silva IZ, Toledo AJF, Marciano BR, Duarte GGM, Soares EA, et al. Insomnia: use of exogenous melatonina and its implications. Res Soc Develop. 2023;12(3):e19212339010. doi:10.33448/rsd-v12i3.39010. [Google Scholar] [CrossRef]
12. Reiter RJ, Sharma R, Cucielo MS, Tan DX, Rosales-Corral SA, Gancitano G, et al. Brain washing and neural health: role of age, sleep, and the cerebrospinal fluid melatonin rhythm. Cell Mol Life Sci. 2023;80(4):516. doi:10.1007/s00018-023-04736-5. [Google Scholar] [PubMed] [CrossRef]
13. Yoo YM, Joo SS. Melatonin can modulate neurodegenerative diseases by regulating endoplasmic reticulum stress. Int J Mol Sci. 2023;24(3):2381. doi:10.3390/ijms24032381. [Google Scholar] [PubMed] [CrossRef]
14. Reiter RJ, Sharma R, Rosales-Corral S, Zuccari DAPC, Almeida Chuffa LG. Melatonin: a mitochondrial resident with a diverse skill set. Life Sci. 2022;301(6):120612. doi:10.1016/j.lfs.2022.120612. [Google Scholar] [PubMed] [CrossRef]
15. Feng L, Jiang X, Kitazawa H, Wang X, Guo Y, Li L, et al. Characterization of bioactive films loaded with melatonin and regulation of postharvest ROS scavenging and ascorbate-glutathione cycle in Agaricus bisporus. Postharvest Biol Technol. 2022;194(2):112107. doi:10.1016/j.postharvbio.2022.112107. [Google Scholar] [CrossRef]
16. Mohammed SH, Karatepe M. The new melatonin derivative: synthesis, characterization, biological properties, and serum determination by HPLC-UV. Appl Nanosci. 2022;13(3):2611–23. doi:10.1007/s13204-021-02195-7. [Google Scholar] [CrossRef]
17. Ahmad SB, Ali A, Bilal M, Rashid SM, Wani AB, Bhat RR, et al. Melatonin and health: insights of melatonin action, biological functions, and associated disorders. Cell Mol Neurobiol. 2023;43(6):1–22. doi:10.1007/s10571-023-01324-w. [Google Scholar] [PubMed] [CrossRef]
18. Chivchibashi-Pavlova D, Stoyanov GS, Bratoeva K. Effects of melatonin supplementation on the aortic wall in a diet-induced obesity rat model. Cureus. 2023;15(1):e33333. doi:10.7759/cureus.33333. [Google Scholar] [PubMed] [CrossRef]
19. Li J, Somers VK, Xu H, Lopez-Jimenez F, Covassin N. Trends in use of melatonin supplements among us adults, 1999–2018. JAMA. 2022;327(5):483–5. doi:10.1001/jama.2021.23652. [Google Scholar] [PubMed] [CrossRef]
20. Liu Y, Wang D, Li T, Xu L, Li Z, Bai X, et al. Melatonin: a potential adjuvant therapy for septic myopathy. Biomed Pharmacother. 2023;158(117):114209. doi:10.1016/j.biopha.2022.114209. [Google Scholar] [PubMed] [CrossRef]
21. Thomas M, Setty S, Kaveeshwar V, Puranik SN. Efficacy of melatonin supplementation as an adjunct to periodontal therapy. Int J Drug Res Dent Sci. 2023;5(1):1–7. doi:10.36437/ijdrd.2023.5.1.A. [Google Scholar] [CrossRef]
22. Waldhauser F, Saletu B, Trinchard-Lugan I. Sleep laboratory investigations on hypnotic properties of melatonin. Psychopharmacology. 1990;100(2):222–6. doi:10.1007/BF02244410. [Google Scholar] [PubMed] [CrossRef]
23. Zisapel N. New perspectives on the role of melatonin in human sleep, circadian rhythms and their regulation. Br J Pharmacol. 2018;175(16):3190–9. doi:10.1111/bph.14116. [Google Scholar] [PubMed] [CrossRef]
24. Iwamoto BK, Decker KM, Byars KC, Van Dyk TR. Impact of exogenous melatonin on sleep and daytime functioning in healthy, typically developing adolescents. Current Sleep Med Rep. 2022;8(5):1–12. doi:10.1007/s40675-022-00235-4. [Google Scholar] [CrossRef]
25. Lamolha MA, Senna LA, Oliveira DA. Development and evaluation of melatonin orodispersible films obtained through magistral practice. Braz J Develop. 2023;9(2):8696–711. doi:10.34117/bjdv9n2-163. [Google Scholar] [CrossRef]
26. Trovatti E, Carvalho AJF, Gandini A. A new approach to blending starch with natural rubber. Polym Int. 2014;64(5):605–10. doi:10.1002/pi.4808. [Google Scholar] [CrossRef]
27. Tamanini F, Moraes BS, Amaral CST, Carvalho AJF, Trovatti E. Starch-based orodispersible film for diclofenac release. Braz J Pharm Sci. 2023;59(1):e211019. [Google Scholar]
28. Bruschi ML. Mathematical models of drug release. In: Strategies to modify the drug release from pharmaceutical systems. Cambridge, England: Woodhead Publishing; 2015. p. 63–86. doi 10.1016/B978-0-08-100092-2.00005-9. [Google Scholar] [CrossRef]
29. Musazzi UM, Dolci LS, Albertini B, Passerini N, Cilurzo F. A new melatonin oral delivery platform based on orodispersible films containing solid lipid microparticles. Int J Pharm. 2019 Jan 25;25(559):280–8. doi:10.1016/j.ijpharm.2019.01.046.Epub. [Google Scholar] [CrossRef]
30. Musazzi UM, Selmin F, Franzé S, Gennari CGM, Rocco P, Minghetti P, et al. Poly(methyl methacrylate) salt as film forming material to design orodispersible films. Eur J Pharm Sci. 2018;115:37–42. [Google Scholar] [PubMed]
31. Mfoafo KA, Omidian M, Bertol CD, Omidi Y, Omidian H. Neonatal and pediatric oral drug delivery: hopes and hurdles. Int J Pharm. 2021;597:120296. [Google Scholar] [PubMed]
Cite This Article
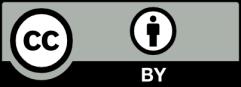
This work is licensed under a Creative Commons Attribution 4.0 International License , which permits unrestricted use, distribution, and reproduction in any medium, provided the original work is properly cited.