Open Access
ARTICLE
Structural Elucidation of the Polymeric Condensed Tannins of Acacia nilotica Subspecies by 13C NMR, MALDI-TOF and TMA as Sources of Bioadhesives
1 Institute of Engineering Research and Materials Technology, National Center for Research, P.O. Box 2404, Khartoum, Sudan
2 ENSTIB-LERMAB, University of Lorraine, 27 rue Philippe Seguin, Epinal, 88000, France
3 Department of Materials Science and Engineering, Institute of Analytical Sciences and Physico-Chemistry for the Environment and Materials-Xylomat (IPREM-UMR5254), University of Pau and the Adour Region, E2S UPPA, CNRS, Mont de Marsan, 40004, France
* Corresponding Authors: Zeinab Osman. Email: ; Antonio Pizzi. Email:
Journal of Renewable Materials 2024, 12(7), 1291-1310. https://doi.org/10.32604/jrm.2024.051619
Received 11 March 2024; Accepted 09 May 2024; Issue published 21 August 2024
Abstract
Tannin was extracted from different subspecies of Acacia nilotica, Acacia nilotica nilotica (Ann), Acacia nilotica tomentosa (Ant) and Acacia nilotica adansonii (Ana). The aim was to elucidate their structure and evaluate their reactivity as bioadhesives in the wood industry. The extracts were prepared by hot water extraction (90°C temperature). Their gel time with paraformaldehyde was used at first to compare their reactivity. The tannin contents and the percentage of total polyphenolic materials in different solutions of the extracts spray dried powder were determined by the hide powder method. Concentrated solutions (47%) were tested by both MALDI ToF, CNMR. The thermomechanical analysis (TMA) was performed to evaluate their modulus of elasticity (MOE) at different pHs. The gel times of all the three tannin extracts showed that their reactivity and it was comparable to other known procyanidin/prodelphinidin tannin extract types. Ana, Ann and Ant showed highest percent of total polyphenolic materials at 70%, 64%, and 57%, respectively. The 13CNMR spectra showed that the three subspecies of condensed tannins were mainly constituted of procyanidins (PC) and prodelphinidins (PD) in slightly different ratios. Ann (56.5% PC and 43.4% PD), Ant (57%PC and 43% PD) and Ana (58% PC and 42% PD). MALDI–TOF spectra showed the presence of flavonoid monomers, and oligomers some of which linked to short carbohydrates monomers or dimers. TMA revealed that the three types of tannins had high MOE at their initial pH (5).Graphic Abstract
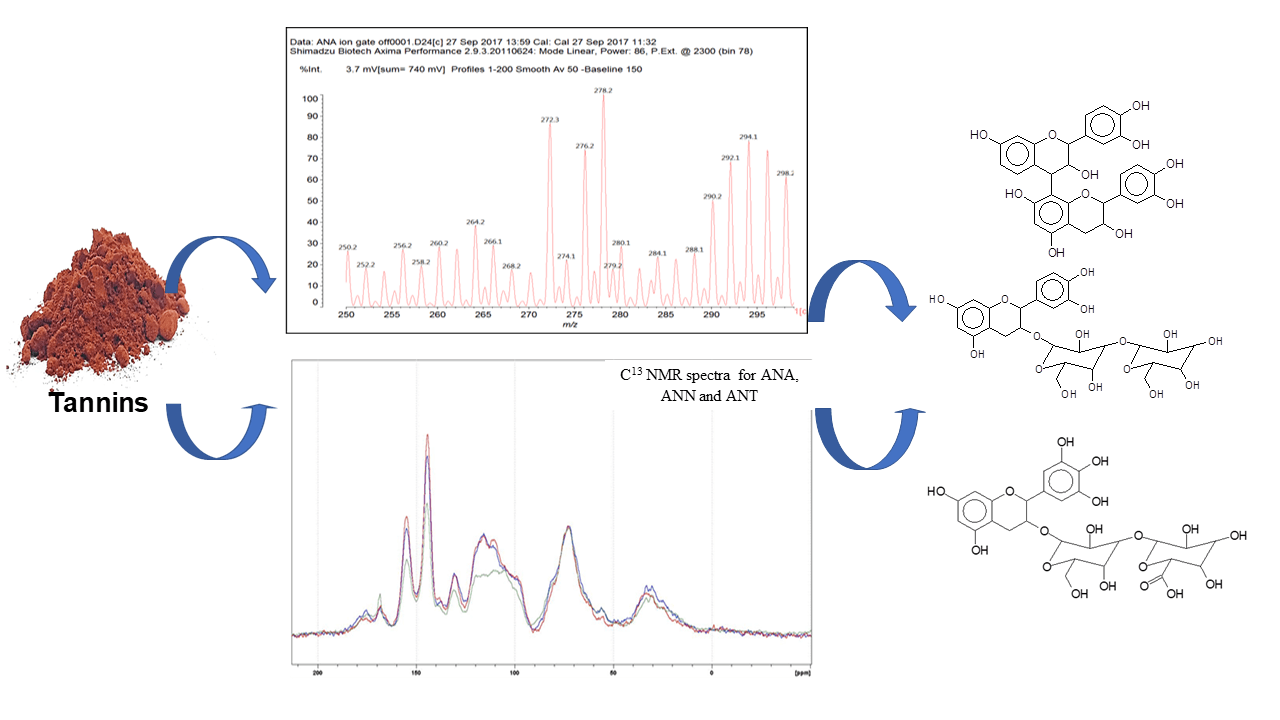
Keywords
Vegetable tannins are traditional materials to tan hides into leather. The most acceptable and simple definition for tannins is that of Bate-Smith et al. [1] “water soluble phenolic compounds having molecular weights between 500–3000”. According to their chemical nature and structural characteristics vegetable tannins are subdivided in two groups: condensed tannins and hydrolysable tannins. Condensed tannins are composed of flavonoids (flavan 3-ol or flavan 3, 4-diol) without a sugar core, however, the hydrolyzable tannins are composed of ellagic and gallic acids with a sugar core mainly glucose [2].
The condensed tannins are chemically heterogeneous oligomers and polymers. The extractable condensed tannin polymers in the plants may be composed of molecular species with a wide range of 20000 Da. Condensed tannins are often referred to as proanthocyanidins as they can release anthocyanidins upon depolymerization. Catechin and epicatechin are the most representative monomeric units in natural condensed tannins together with epicatechin gallate, gallocatechin, epigallocatechin, afzelechin, and epiafzelechin, Fig. 1 [3–7]. The bioactivity capacity of condensed tannins is generally recognized to be largely dependent on their structure and particularly their degree of polymerization [8].
Figure 1: The structure of the condensed tannins and main monomeric components
The introduction of tannin as a renewable material has provided new opportunities in various applications since the early 1980s [9]. After more extensive studies in recent years, the properties of Radiata pine, Quebracho, Mimosa bark tannins, and others have become better understood, and improved technologies have been developed for their use as wood adhesives [10].
Recently, increasing interest in their usage in the wood industry has attracted attention to their utilization and structural elucidation. However, the great variation in their structure and concentration within plant species makes this difficult because of their heterogeneous character [11]. Procyanidin-type tannins are among the more diffuse flavonoid tannins in nature. Among the more interesting characteristics of tannins that are useful for their adhesive application are the proportion of procyanidin vs. prodelphinidin and other flavonoid units which determines their reactivity towards aldehydes and hence their rate of cross-linking [12].
Furthermore, the diversity of condensed tannins is given by the structural variability of the monomer units which present different hydroxylation patterns of the aromatic rings A and B and different configurations at the chiral centers C2 and C3 (Fig. 1). Due to this complexity and diversity, the characterization of highly polymerized proanthocyanidins thus remains very challenging, and less is known regarding their structure-activity relationships [8]. Work on the application of matrix-assisted laser desorption ionization time of flight mass spectrometry (MALDI-ToF MS) for analysis of oligomeric polyphenols in foods [13–16] and adhesives [17–19] have appeared in the last 20 years. The polymer chain length distribution, frequency of monomer unit as well as degree of polymerization which were difficult to determine by other techniques were more easily determined by MALDI-ToF.
In Sudan, there are many indigenous and exotic plant species that contain tannins in different quantities. Acacias species were found to present high tannin content percentages [20]. The most important of these was Acacia nilotica which dominates in Sudan with three subspecies:
- Sub sp. nilotica (Ann) with glabrous pods, strongly constricted between the seeds.
- Sub sp. adansonii (Ana) with the pods only slightly constricted between the seeds.
- Sub sp. tomentosa (Ant) with the pods necklace-like narrowly and regularly constricted [21] between the seeds.
The bark tannins extracted from these subspecies have not been previously investigated. Nevertheless, the tannins from their pods have been evaluated and their chemical composition has been clarified by thin-layer chromatography (TLC). Previous research work [22] showed that the pods and bark contained good percentages of tannins and that their tannins were of mixed hydrolysable-condensed (gallo-catechol) type. However, their suitability as bioadhesive precursors has not been studied.
Therefore, the aim of this study is to evaluate the tannins extracted from the bark of the three subspecies of Acacia nilotica as a source of bioadhesives. The tannins extracted by hot water were spray dried, their content was evaluated and their structure was elucidated using chemical analysis, 13C Nuclear magnetic resonance (13CNMR), and MALDI-ToF MS. Adhesives prepared from the tannins were evaluated by means of gel time and thermomechanical analysis. The study concluded that the three barks contained high percentages of condensed tannins that their reactivity is comparable to the pine tannins and is therefore suitable for adhesive preparation.
In this study, the condensed tannins from the bark of the three subspecies of Acacia nilotica was extracted by hot water and characterized by using the gel time method, and thermomechanical analysis to evaluate and compare their reactivity as wood adhesives for commercial purposes.
All bark samples were collected from Wad elnayel sawmill at the Blue Nile State, West of Sudan, and Khartoum State, Sudan. They were air-dried and ground using a laboratory star mill. The powdered samples were kept in polyethylene bags and left for overnight in order to homogenize their moisture content.
2.2.1 Preparation of Tannins Extraction
Preparation of tannin extracts was carried out according to a reported method [23]. The ground bark was then soaked in hot water (1:6w/v) of 90°C initial temperature and left overnight. The solutions were then filtered using a special cloth (50–90 µm). Then, they were further spray dried using a Heidolph YC-500 Laboratory Spray Dryer (Heidolph, Schwabach, Germany) at 175°C. An aqueous solution of 40% concentration was prepared from the spray-dried powder of Ann, Ana, and Ant tannins and used for further testing.
2.2.2 Determination of the Tannins Content and Total Polyphenolics
Chemical analysis was done on the aqueous concentrated solution of the tannins (40%). The official hide powder method [24] was used for tannins percentage determination. Tannins absorbed by the standard hide powder were expressed as a percentage of the original tanning extract. As for the total polyphenolic materials, the Stiasny method [25] was used according to a method revised and reported more recently [26,27].
The gel time for the three subspecies of Acacia nilotica was carried out according to the DIN 16945 standard [28]. An aqueous tannins solution of 40% was prepared from the spray dried of bark of the three subspecies of Acacia nilotica. Their pH was modified (4–9) using 33% solution of NaOH in water. To each, 5% of paraformaldehyde was added. Approximately 10 g of each glue was placed in a test tube, under stirring at 100°C until hardening.
The concentrated tannin extracts (aqueous solution 40%) were analyzed by 13CNMR according to a reported method [29]. The liquid 13CNMR spectrum of the tannin extracts was obtained on a Bruker MSL 300 FT-NMR spectrometer (Bruker, Wissembourg, France). The chemical shifts were calculated with respect to (CH3)3Si(CH2)3SO3Na dissolved in D2O for NMR shift control. The spectra were taken at 62.90 MHz. The spectra (10000 transients) were attained on a Bruker MSL300 FT-NMR spectrometer, at a frequency of 62.9 MHz and with a precision of 1 ppm. The relaxation delay was 5 s.
2.2.5 Matrix Assisted Laser Desorption Ionization Time-of-Flight (MALDI-ToF) Analysis
The samples were treated with a NaCl solution (1.5 µl of a 0.1 M) in a methanol/water mixture (1:1) to increase ion formation, and a drop was placed on the MALDI target (3 mm diameter) steel plate and dried. The samples and the matrix were then mixed in equal amounts, and 1.5 µl of the resulting slurry was placed on the MALDI target and dried at 40°C for 2 h before being analysed. A matrix of 2, 5-dihydroxy benzoic acid was used. Red phosphorous (500–3000 Da) was used as a reference for spectrum calibration. Finally, after evaporation of the solvent, the MALDI target was introduced into the spectrometer.
The spectra were recorded on a KRATOS AXIMA Performance mass spectrometer from Shimadzu Biotech (Kratos Analytical Shimadzu Europe Ltd., Manchester, UK). The irradiation source was a pulsed nitrogen laser with a wavelength of 337 nm. The length of one laser pulse was 3 ns. Measurements were carried out using the following conditions: polarity-positive, flight path-linear, 20 kV acceleration voltages, 100–150 pulses per spectrum. The delayed extraction technique was used applying delay times of 200–800 ns. The software MALDI-MS was used for the data treatment. The oligomers can appear in the spectra either corresponding to their molecular weight or to their molecular weight +23 Da of the Na+ ion derived from the NaCl used as an enhancer. The spectra precision is of +1Da.
2.2.6 Thermomechanical Analysis (TMA)
TMA is used to determine the rate of curing and the prospective mechanical strength performance of tannin and other wood adhesives. The temperature test range is in general from 25°C to 200°C at a 10°C/min heating rate ramp. The maximum MOE value reached and the rate at which it is achieved are the two main values determined [30,31].
30 mg of gluemix composed of a 40% solution of tannin extract and 8% by weight fine paraformaldehyde powder is placed between two thin beech wood plys of 0.5–0.6 mm thickness each, to form a sandwich joint of 20 mm × 6 mm × 1.26 mm and tested in three points bending with a span of 18 mm and subjected to an alternating force of 0.1/0.5 N with a cycle of 6/6 s at a 10°C/min heating rate. The minimum value of the deflection on curing is then measured and converted to the maximum value of MOE.
3.1 Chemical Analysis of the Tannins
Table 1 shows the results of the chemical analysis of the official hide-powder method. It was highest for both, Ant and Ana (54% and 53%) respectively followed by Ann (48%). These percentages are much higher than the level of previous work for commercial interests and higher than the tannins extracted from similar Acacais (10%) [20,32]. It must be pointed out that the hide powder method is focused on leather tanning, thus it detects only flavonoid oligomers from trimers upward while dimers and monomers still very useful in other applications are not detected by this method.
This method has been used to determine the percentages of the tannins available in these barks and extracts. Nevertheless, as it is focused on leather tanning, the main drawback of this method is its inability to give information on the nature of monoflavonoid or biflavonoid, in leather tannins being defined as molecules from trimers upwards. Thus, important flavonoid fractions of the extract useful for other applications are not detected [27,33]. All the flavonoid contributions can instead be determined by measuring the tannins reactivity towards formaldehyde. Therefore, the determination of the percentages of polyphenolic materials (catechin number), the part rich in condensed tannins as suggested by other authors [34] was carried out. The results showed that Ana has the highest percentage (70%), which implies high reactivity with the hardeners such formaldehyde and hexamethylenetetramine (hexamine), in comparison to Ann and Ant for which the percentages were slightly low (64% and 57%), respectively.
The amount of water-soluble materials (sugars, gum, and tannins) is almost 80% for the three tannins. The high percentage of these materials indicates indirectly the presence of tannins as they are soluble in hot water [35]. The tannin purity or the ratio of tannin/soluble material was good, ≥0.6, for all subspecies of Acacia nilotica and comparable to previous studies [20].
The results obtained indicate that Ana is more reactive as it contains the highest percentages of condensed tannin. However, it could be concluded that all three bark of Acacia nilotica contain high tannin percentages and it is expected to have desirable properties for bioadhesives and biopolymers application.
The gel time results are shown in Fig. 2. It has been observed that the pH has a pronounced influence on the gel time which became shorter at alkaline pH. It indicates that the characteristics of Ana show a different constitution to Ann and Ant. While from pH 6 upward the curves of gel time of the three subspecies are indeed very similar, indicating their high reactivity as a function of pH characteristic of procyanidin/prodelphinidin type tannins, the somewhat slightly slower gel time. The upward curve of Ana at pHs 4 and 5 indicates that Ana is likely to have a higher proportion of procyanidin type oligomers than Ann and Ant while to similar extents these latter have a predominance of prodelphiniding type oligomers. This fact is supported by the results obtained by 13C NMR spectroscopy. All three species then should be well suited to use as wood adhesives.
Figure 2: Gel times as a function of pH of the Ann, Ana and Ant tannin extracts
For further investigations on the structure of the condensed tannins, 13CNMR analysis was employed. The 13CNMR spectra (Figs. A1–A4) and Table 2 for the three subspecies of Acacia nilotica present information on the nature of their tannin extracts. The signals were assigned according to Czochanska et al. [36]. The three spectra show typical signals due to the presence of condensed tannins (Fig. A4), containing PD and PC units. The signals at 116 ppm (C2′, C5′), 120 ppm (C6′), and 145 ppm (C3′, C4′) show the presence of PC units (catechin/epicatechin). The sharp and high signal at 145–147 ppm is typical of the presence of PD units (gallocatechin/epigallocatechin) as not only represents the –OH carrying carbons in C3′ and C4′ but superimposed also a C5′ carrying an –OH group, this being confirmed from the intensity decrease of the peak at 116 ppm where the main contribution is mainly limited to the C2′ and much less C5′ without an –OH group linked. The PC/PD ratio of condensed tannins is usually and approximately determined from the relative ratio of these two peaks. According to the intensity of these peaks presented in Table 2, the PC/PD ratios for each tannin were 30%/70% for Ann, 40%/60% for Ant and 50%/50% for Ana. In Ant, is also evident a decrease in intensity of the 110 ppm peak that pertains to the interflavonoid bond C4–C8 indicating that Ana and Ann present a higher percentage of a higher degree of polymerization oligomers than Ant (Figs. A2 and A3).
The region between 30 and 90 ppm is due to the signals of C2, C3, and C4 in flavan-3-ol units. The split peak and the two signals at 76–72 ppm although partially masked by the intense peak at 72 ppm of the carbohydrate oligomers of the extract indicate the presence of 2,3-cis and at 85–84 ppm to 2,3-trans isomers The spectra demonstrate that both stereoisomers co-exist in all the three tannins. However, the intensity of the shifts of these stereoisomers was equal in both Ann and Ana while Ant has a majority of the trans form (Table 2). It has also been noticed that the Ana tannins have a majority of both forms among the three tannins types.
Thus, it can be concluded from these results that the condensed tannins of Ann and Ant extracts are predominantly constituted of prodelfinidins while Ana is constituted of equal proportions of procyanidins and prodelphinidins, a result confirming the indications obtained by the gel time test. Hence, all three are expected to be excellent precursors for wood adhesive application [37].
MALDI-ToF analyses has been employed in order to obtain more detailed information on the chemical composition of the three tannins extracts, which are particularly well suited for large and small molecules from a variety of different vegetable sources [38] both for food grade materials [39,40] and commercial tannins [41,42].
In this study, MALDI-TOF with deionization and selection of Na+ as the cationization reagent was used according to previously codified and accepted procedures [17,18,43,44] to enhance the flight of oligomers to the target of the spectrometer.
Fig. B1 shows the MALDI-ToF mass spectra of the Ana tannins extracts as this is the richest in polyphenolics. In general, the spectra of the three tannin extracts are almost similar, having the same series of peaks but with somewhat slightly different intensities, hence, Ana spectra was provided as a model of these tannin. The structural assignment for the Ana and Ann extracts and the extra peaks present in the Ann extract are shown in Table 3.
On top of flavonoid momomers, dimers, and trimers of mixed composition that are logically expected in MALDI of flavonoid tannins [17,18], there are a few species that merit attention. Thus at 365 Da a glucose dimer and the species at 697 Da is a tetramer of glucuronic acid and glucose, both residues of hydrolyzed hemicelluloses, a type of residual species always present in condensed tannin raw extracts. The presence of this confirms the presence of carbohydrate monomer and dimer chains linked to the flavonoid units, a fact that has already been noticed in other tannin extracts from African hardwood species [45,46]. In this respect also of interest is the presence of the species at 667 Da, in which a glucuronic acid is present in a carbohydrate dimer side chain linked to a flavonoid unit. This could have been generated during the extraction process or in the MALDI equipment. It is also not possible to ascertain to which site of the flavonoid units the carbohydrate side chains are linked to. They can equally be on the phenolic OH groups or on the C3 –alcoholic –OH group on the flavonoid units heterocyclic ring. Equally degradation sub-products are present as the species at 346, 368, 380 and 398 Da, the mechanism of formation being exemplified in Fig. 3.
Figure 3: An example of the passage of dimers to fragmented substructures with the Da values for each species
The Data obtained by MALDI support what gleaned on these tannins by 13CNMR.
A comparison of the experimental intensities of the PD and PC characteristic peaks shown in Table 4 indicates that Ana is a tannin with approximately equal contribution of procyanidin and prodelphinidin structures while Ant is a predominantly procyanidin type of tannin and Ann a predominantly prodelphinidin one.
Thus, the results of MALDI-ToF appear to support the results of 13CNMR, and showed to be an appropriate and convenient technique to examine the distribution of condensed tannin oligomers. It also allows the determination of the structure and characteristics of tannins which would be difficult to characterize by other analytical tools.
3.5 Thermomechanical Analysis (TMA)
To confirm the suitability of the three tannins extracted from the subspecies of Acacia nilotica for use as tannin-based adhesives and to compare their relative reactivities, TMA analysis was performed. The data provided here were deducted from a previous study [47,48]. The evolution of the Modulus of Elasticity (MOE) of the adhesives as a function of temperature and time was measured for the three tannins subspecies The results are shown in Fig. 4.
Figure 4: TMA curves of the increase in MOE on hardening with paraformaldehyde of Ana (a), Ann (b), And (c) tannin extracts at three different conditions of pH4, pH 5 and pH 7
Fig. 4a–c shows that Ana and Ant achieve the higher MOE with a faster increase in temperature. The slope starts at a lower temperature at pH 7 than that of Ann. Ana and Ant have the second highest MOE and the second fast slope of MOE increase as a function of temperature. It shows that as the proportion of the more reactive prodelphinidin in the tannin increases the performance of the glue mix is worse both as regards maximum MOE value as well as in fastness of slope increase. This indicates that the more reactive prodelphinidins may cross-link so fast to immobilize the cross-linked network at an earlier, less cross-linked level the reaction not be able to proceed further. The Ant and Ana tannins richer in procyanidins can instead proceed to a more complete cross-linking with a lower level of steric hindrance. In general, the maximum value of the MOE obtained at pH 4, the natural pH of the three tannin extracts, is the lowest indicating undercuring under such a pH condition. It has been reported that the three tannins autocondensed at their initial pH at room temperature [48]. As the pH reaches 5 and particularly 7 the MOE reaches its maximum value. The maximum MOE values are similar for the three tannin extracts, namely 2750 MPa for Ana and Ant, and 2650 MPa for Ann.
Among the techniques that have been used to characterize the tannin extracts of the three Acacia nilotica subspecies, the chemical analysis has allowed to distinguish the percentage, the type and quantity of the tannins in the studied subspecies. It can also give an idea about the reactivity of the tannins and their final use in the adhesives’ formulations.
The results obtained from the chemical analysis demonstrated that Ana has the highest percentage (70%), which implies high reactivity with the hardeners such formaldehyde and hexamethylenetetramine (hexamine), in comparison to Ann and Ant for which the percentages were slightly low (64% and 57%), respectively.
The results deducted from the 13CNMR showed that the condensed tannins of Ann and Ant extracts are predominantly constituted of prodelfinidins while Ana is constituted of equal proportions of procyanidins and prodelphinidins, a result confirming the indications obtained by the gel time test.
The data on the chemical structure of these tannins obtained from MALDI were in agreement with what had been achieved by 13CNMR analysis; Ana is a tannin with approximately equal contribution of procyanidin and prodelphinidin structures while Ant is a predominantly procyanidin type of tannin and Ann a predominantly prodelphinidin one. The three tannins studied are mainly gallocatechin (delphinidin), catechin and epicatechin and some fisetinidin.
The thermomechanical analysis which was performed in order to evaluate the strength of adhesives prepared from these tannins showed that the maximum MOE values are similar for the three tannin extracts, namely 2750 MPa for Ana and Ant, and 2650 MPa for Ann. The results confirmed the high reactivity of these tannins which produced high MOE values that could be suitable for application in the wood industry.
The results obtained by the different techniques were consistent, and alone or all together could be used to study the structural characterization of tropical woods polyflavonoid tannins and they can be viable tools for the valorization of their suitability as tannins-based adhesives.
Acknowledgement: Z. Osman would like to aknowledge the financial support for a research visit provided by PAUSE program, College de France, and Pau university.
Funding Statement: The authors would like to acknowledge the fund provided by NAPATA program, jointly funded by France campus and the Ministry of Higher Education and Scientific research, Sudan. Lab facilities provided by LERMAB which is supported by a grant of the French Agence Nationale de la Recherche (ANR) in the ambit of the laboratory of excellence (Labex) ARBRE is also aknowledged.
Author Contributions: The authors confirm contribution to the paper as follows: study conception and design: Zeinab Osman, Antonio Pizzi; data collection: Zeinab Osman; analysis and interpretation of results: Zeinab Osman, Antonio Pizzi; draft manuscript preparation: Zeinab Osman, Antonio Pizzi, Bertrand Charrier. All authors reviewed the results and approved the final version of the manuscript.
Availability of Data and Materials: Data will be available upon request.
Conflicts of Interest: The authors declare that they have no conflicts of interest to report regarding the present study.
References
1. Bate-Smith EC, Swain T. Flavonoid compounds. In: Mason HS, Florkin AM, editors. Comparative biochemistry. New York: Academic Press; 1962. vol. 3, p. 764. [Google Scholar]
2. Sharma KP. Tannin degradation by phytopathogen’s tannase: a Plant’s defense perspective Biocatal. Agric Biotechnol. 2019;21:101342. doi:10.1016/j.bcab.2019.101342. [Google Scholar] [CrossRef]
3. Tanner GJ, Francki KT, Abrahams S, Watson JM, Larkin PJ, Ashton AR. Proanthocyanidin biosynthesis in plants: purification of legume leucoanthocyanidin reductase and molecular cloning of its cDNA. J Biol Chem. 2003;278:31647–56. doi:10.1074/jbc.M302783200. [Google Scholar] [PubMed] [CrossRef]
4. Shahidi F, Varatharajan V, Oh WY, Peng H. Phenolic compounds in agri-food by-products, their bioavailability and healtheffects. J Food Bioact. 2019;5:57–119. [Google Scholar]
5. Fraga-Corral M, García-Oliveira P, Pereira AG, Lourenço LC, Jimenez-Lopez C, Prieto MA, et al. Technological application of tannin-based extracts. Molecules. 2020;25:614. [Google Scholar]
6. Molino S, Casanova NA, Rufián Henares JA, Fernandez ME. Natural tannin wood extracts as a potential food ingredient in the food industry. J Agric Food Chem. 2020;68:2836–48. doi:10.1021/acs.jafc.9b00590. [Google Scholar] [PubMed] [CrossRef]
7. Zeller WE. Activity, purification, and analysis of condensed tannins: current state of affairs and future endeavors. Crop Sci. 2019;59:886–904. doi:10.2135/cropsci2018.05.0323. [Google Scholar] [CrossRef]
8. Rue EA, Glinski JA, Glinski VB, van Breemen RB. Ion mobility-mass spectrometry for the separation and analysis of procyanidins. J Mass Spectrom. 2020;55(2):e4377. doi:10.1002/jms.v55.2. [Google Scholar] [CrossRef]
9. Dhawale PV, Vineeth SK, Gadhave RV, Fatima MJ, Supekar MV, Thakur VK, et al. Tannin as a renewable raw material for adhesive applications: A review. Journal Mater. Adv. 2022;3(8):3365–88. doi:10.1039/D1MA00841B. [Google Scholar] [CrossRef]
10. Pizzi A. Tannins: prospectives and actual industrial applications. Biomolecules. 2019;9(8):344. doi:10.3390/biom9080344. [Google Scholar] [PubMed] [CrossRef]
11. Molino S, Francino MP, Henares JR. Why is it important to understand the nature and chemistry of tannins to exploit their potential as nutraceuticals? Food Re Int. 2023;173(2):113329. [Google Scholar]
12. Thompson D, Pizzi A. Simple 13C NMR methods for quantitative determination of polyflavonoid tannin characteristics. J Appl Polym Sci. 1995;55:107–12. doi:10.1002/app.07.v55:1. [Google Scholar] [CrossRef]
13. Low JH, Rahman WAWA, Jamaluddin J. Structural elucidation of tannins of spent coffee grounds by CP-MAS 13C NMR and MALDI-TOF MS. Ind Crops Prod. 2015;69:456–61. doi:10.1016/j.indcrop.2015.03.001. [Google Scholar] [CrossRef]
14. Pinaffi ACDC, Sampaio GR, Soares MJ, Shahidi F, de Camargo AC, Torres EAFS. Insoluble-bound polyphenols released from guarana powder: inhibition of alpha-glucosidase and proanthocyanidin profile. Molecules. 2020;25(3):679. doi:10.3390/molecules25030679. [Google Scholar] [PubMed] [CrossRef]
15. Esquivel AD, Alfaro VE, Krueger CG, Vestling MM, Reed JD. Identification of A-type proanthocyanidins in cranberry-based foods and dietary supplements by matrix-assisted laser desorption/ionization time-of-flight mass spectrometry. J AOAC Int. 2021;104(1):223–31. doi:10.1093/jaoacint/qsaa106. [Google Scholar] [PubMed] [CrossRef]
16. Sei-ichi K, Toda K, Matsumoto K, Chisato I, Shuhei N, Chisato M, et al. Isolation and characterization of a novel oligomeric proanthocyanidin with significant anti-cancer activities from grape stems (Vitis vinifera). Sci Rep. 2019;9:12046. doi:10.1038/s41598-019-48603-5. [Google Scholar] [PubMed] [CrossRef]
17. Pasch H, Pizzi A, Rode K. MALDI-TOF mass spectrometry of polyflavonoid tannins. Polymers. 2001;42(18):7531–9. doi:10.1016/S0032-3861(01)00216-6. [Google Scholar] [CrossRef]
18. Pasch H, Pizzi A. On the macromolecular structure of chestnut ellagitannins by MALDI-TOF mass spectrometry. J Appl Polym Sci. 2002;85(2):429–37. doi:10.1002/app.v85:2. [Google Scholar] [CrossRef]
19. Pizzi A, Pasch H, Kassim MJ. Study on the structure of mangrove polyflavonoid tannins with MALDI-TOF mass spectrometry. J Appl Polym Sci. 2008;109:963–7. doi:10.1002/app.v109:2. [Google Scholar] [CrossRef]
20. Ahmed M, Khirstova P, Ich G. Comparative study of tannins of Acacia nilotica, an indigenous tanning material in Sudan with Acacia mearnsii. Suranaree J Sci Technol. 2005;12(4):259–65. [Google Scholar]
21. Badi KH, El Houri A, Bayoumi AMS. The forests of the Sudan. Khartoum: Forests National Corporation and National Council for Research; 1989. [Google Scholar]
22. Ahmed M, Khirstova P, Ich G. Characterization of Acacia nilotica as an indigenous tanning material of Sudan. J Trop For Sci. 2006;18(3):181–7. [Google Scholar]
23. Osman Z, Eltayeb F, Albadawi M, Khalied MA. Evaluation of the antioxidant activities of water extracted polyphenolics contents of some Acacias. Species J for prod ind. 2014;3(2):89–92. [Google Scholar]
24. Society of Leather Trade Chemists. Official methods of analysis. Redbourne, UK: International Society of Leather Trade Chemists; 1965. [Google Scholar]
25. Stiasny E. Die einwiirkung von formaldehyde auf gerbstoffe. Der Gerber. 1905;740:186–8. [Google Scholar]
26. Yazaki Y, Zheng G, Searle S. Extractive yields and polyphenolic contents from Acacia mearnsii barks in Australia. Aust For. 1990;53(3):148–53. doi:10.1080/00049158.1990.10676071. [Google Scholar] [CrossRef]
27. Pizzi A, Laborie MP, Candan Z. A review on sources, extractions and analysis methods of a sustainable biomaterial: tannins. J Renew Mater. 2024;12(3):397–425. doi:10.32604/jrm.2023.046074. [Google Scholar] [CrossRef]
28. German Standard DIN 16945. Testing of resins, hardeners and accelerators, and catalyzed resins. 1989. [Google Scholar]
29. Breitmaier EW, Carbon-13 NMR spectroscopy. 13th ed Weinheim, Germany: VCH; 1987. [Google Scholar]
30. Ghahri S, Chen X, Pizzi A, Hajihassani R, Papadopoulos AN. Natural tannins as new cross-linking materials for soy-based adhesives. Polymers. 2021;13(4):595. doi:10.3390/polym13040595. [Google Scholar] [PubMed] [CrossRef]
31. Ghahri S, Pizzi A, Mohebby B, Mirshokraie A, Mansouri HM. Soy-based, Tannin-modified plywood adhesives. J Adhes. 2018;94:218–37. doi:10.1080/00218464.2016.1258310. [Google Scholar] [CrossRef]
32. Lionel KT, Danwe R, Konai N, Pizzi A, Meva’a L. Thermomechanical analysis of African tannins resins and biocomposite characterization. J Adhes Sci Technol. 2020;35(14):1492–9. [Google Scholar]
33. Garro Galvez JM, Riedl BB, Conner AH. Analytical studies on tara tannins. Holzforschung. 1997;53:235–43. [Google Scholar]
34. Fechtal M, Reidl BB, Calve L. Modeling of tannins as adhesives. I. Condensation of the (+)-catechin with formaldehyde. Holzforschung. 1993;47:419–24. doi:10.1515/hfsg.1993.47.5.419. [Google Scholar] [CrossRef]
35. Stevanovic T, Perrin D. Les extractibles du bois. Available from: https://www.eyrolles.com/Sciences/Livre/chimie-du-bois-9782880747992/. [Accessed 2009]. [Google Scholar]
36. Czochanska Z, Foo LY, Newman RH, Porter LJ. Polymeric proanthocyanidins. Stereochemistry, structural units, and molecular weight. J Chem Soc. 1980;1:2278–86. [Google Scholar]
37. Vivas N, Nonier MF, Gaulejac NV, Bertrand CA, Mirabel M. Differentiation of proanthocyanidin tannins from seeds, skins and stems of grapes (Vitis vinifera) and heartwood of Quebracho (Schinopsis balansae) by matrix –assisted laser desorption/ionization time of flight mass spectrometry and thioacidolysis/liquid chromatography/electrospray ionization mass spectrometry. Anal Chim Acta. 2004;513:247–56. [Google Scholar]
38. Haq N, Muhammad S, Rehman N, Andaleeb H, Ullah N. Effect of solvent polarity on extraction yield and antioxidant properties of phytochemicals from bean (Phaseolus vulgaris) seeds. Braz J Pharm Sci. 2020;56:1–9. [Google Scholar]
39. Šebela M. Biomolecular profiling by MALDI-TOF mass spectrometry in food and beverage analyses. Int J Mol Sci. 2022;23(21):13631. doi:10.3390/ijms232113631. [Google Scholar] [PubMed] [CrossRef]
40. Daiane GR, Lílian STC, Ivonaldo RS, Raphael FA, Luciano PS, Osmundo BON, et al. Applications for bacterial and plant sample differentiation and biological variability assessment. J Proteomics. 2020;213:103619–6. doi:10.1016/j.jprot.2019.103619. [Google Scholar] [PubMed] [CrossRef]
41. Zhen L, Lange H, Crestini C. An analytical toolbox for fast and straightforward structural characterisation of commercially available tannins. Molecules. 2021;26:2532. doi:10.3390/molecules26092532. [Google Scholar] [PubMed] [CrossRef]
42. Engozogho ASP, Bi AAB, Safou TR, Rodrigue ST, Francisco JM, Thomas C, et al. The condensed tannins of Okoume (Aucoumea klaineana Pierrea molecular structure and thermal stability study. Sci Rep. 2020;10:1773. doi:10.1038/s41598-020-58431-7. [Google Scholar] [PubMed] [CrossRef]
43. Zhang LL, Lin YM. HPLC, NMR and MALDI-TOF MS analysis of condensed tannins from lithocarpus glaber leaves with potent free radical scavenging activity. Molecules. 2008;13(12):2986–97. doi:10.3390/molecules13122986. [Google Scholar] [PubMed] [CrossRef]
44. Xiang P, Lin YM, Lin P, Xiang C, Yang ZW, Lu ZM. Effect of cationization reagents on the matrix-assisted laser desorption/ionization time-of-flight mass spectrum of Chinese gallotannins. J Appl Polym Sci. 2007;105:859–64. doi:10.1002/app.v105:2. [Google Scholar] [CrossRef]
45. Drovou S, Pizzi A, Lacoste C, Zhang J, Abdalla SS, El-Marzouki FM. Flavonoid tannins linked to long carbohydrate chains–MALDI ToF analysis of the tannin extract of the african locust bean. Ind Crops Prod. 2015;67:25–32. doi:10.1016/j.indcrop.2015.01.004. [Google Scholar] [CrossRef]
46. Konai N, Pizzi A, Raidandi D, Lagel MC, L’Hostis CC, Saidou AH, et al. Aningre (Aningeria spp.) tannin extract characterization and performance as an adhesive resin. Ind Crops Prod. 2015;77:225–31. doi:10.1016/j.indcrop.2015.08.053. [Google Scholar] [CrossRef]
47. Osman Z. Comparative thermodynamic study on the contribution of the autocondensation and copolymerization reactions for the tannins of the subspecies of Acacia nilotica. J Polym Environ. 2013;21:1100–8. doi:10.1007/s10924-013-0611-1. [Google Scholar] [CrossRef]
48. Osman Z. Thermomechanical analysis of the tannins of Acacia Nilotica spp. Nilotica as a rapid tool for the evaluation of wood-based adhesives. J Therm Anal Calorim. 2012;107:709–16. doi:10.1007/s10973-011-1721-4. [Google Scholar] [CrossRef]
Appendix A
Figure A1: 13C NMR of the Acacia nilotica nilotica (Ann) subspecies tannin extract
Figure A2: 13C NMR of the Acacia nilotica adansonii (Ana) subspecies tannin extract
Figure A3: 13C NMR of the Acacia nilotica tomentosa (Ant) subspecies tannin extract
Figure A4: Comparison of the 13C NMR of the three subspecies Acacia nilotica adansonii (Ana) (red trace), Acacia nilotica nilotica (Ann) (blue trace) and Acacia nilotica tomentosa (Ant) (green trace) tannin extracts
Appendix B
Figure B1: MALDI ToF spectra of Acacia nilotica adansonii (Ana): (a) 260–300 Da range; (b) 260–300 range; (c) 300–400 Da range; (d) 400–600 Da range; (e) 600–800 Da range; (f) 800–1000 Da range
Cite This Article
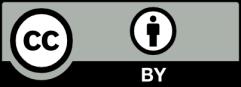
This work is licensed under a Creative Commons Attribution 4.0 International License , which permits unrestricted use, distribution, and reproduction in any medium, provided the original work is properly cited.