Open Access
ARTICLE
Fish Gelatin-Based Film Containing Maillard Reaction Products: Properties and Its Use as Bag for Packing Chicken Skin Oil
1 International Center of Excellence in Seafood Science and Innovation, Faculty of Agro-Industry, Prince of Songkla University, Songkhla, 90110, Thailand
2 Department of Food Safety and Quality Management, Poznań University of Life Sciences, Poznań, 60-637, Poland
3 Center of Excellence in Bio-Based Materials and Packaging Innovation, Faculty of Agro-Industry, Prince of Songkla University, Hat Yai, Songkhla, 90110, Thailand
4 Department of Food and Nutrition, Kyung Hee University, Seoul, 02447, Republic of Korea
* Corresponding Authors: Krisana Nilsuwan. Email: ; Soottawat Benjakul. Email:
Journal of Renewable Materials 2024, 12(6), 1125-1143. https://doi.org/10.32604/jrm.2024.051361
Received 03 March 2024; Accepted 29 May 2024; Issue published 02 August 2024
Abstract
Maillard reaction is a non-enzymatic browning reaction and its products (MRPs) have been proven to possess antioxidant properties. This research aimed to produce a fish gelatin-based packaging incorporated with MRPs to retard lipid oxidation in chicken skin oil (CSO) during storage at ambient temperature (28°C–30°C). MRPs produced from fish gelatin and fructose (1:1, 90°C, pH 11) showed the highest antioxidant properties compared to those prepared under other conditions. Different glycerol/MRPs ratios (30:0, 25:5, 20:10, 15:15, 10:20, 5:25, 0:30) were incorporated into the film and resulting films were characterized. Glycerol/MRPs at 10:20 ratio was chosen to add into the film prior to bag preparation via heat sealing method. CSO packed in the bag was monitored for lipid hydrolysis and oxidation during 15 days of storage (30°C ± 0.5°C, RH 52% ± 5%). After 15 days, quality deterioration was lower in CSO packed in the prepared gelatin bag as evidenced by lower FFA, TBARS, and volatile compounds in comparison with CSO packed in LDPE bag. Fish gelatin film added with MRPs possessed an excellent water vapor barrier property (WV-BP). This finding indicated that MRPs could be used to substitute glycerol and simultaneously could serve as antioxidants for the developed active bag. The novel packaging can be a potential alternative packaging for retarding lipid oxidation of lipid or fatty foods.Graphic Abstract
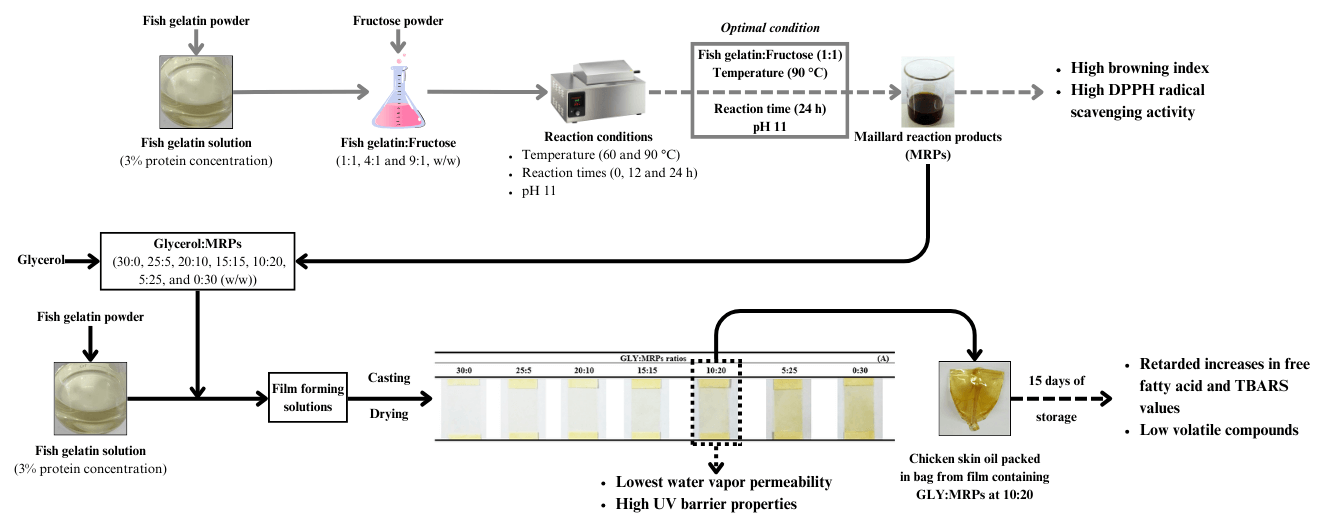
Keywords
Biopolymer has gained an increasing interest as biodegradable food packaging, which is able to replace the use of synthetic polymers. Moreover, sustainable solutions to manage food wastes or by-products via conversion to value-added products can be achieved [1]. Several biopolymers have been produced from food processing by-products, such as chitosan from shrimp processing waste or gelatin from fish processing leftover [2]. Among biopolymers, proteins have the complex compositions and structures, in which the capability of protein molecules to interact with the surrounding molecules allows them to create cohesive and viscoelastic films [3]. Protein-based films, especially based on gelatin, can be obtained from animal processing by-products [1,4]. Gelatin is a fibrous protein obtained from collagen via thermal denaturation or partial hydrolysis. It is applied widely in food and non-food industries [2]. Because of religious prohibitions and outbreaks of bovine spongiform encephalopathy, fish-based gelatin has been considered as a potential alternative [2]. Fish gelatin is an attractive biopolymer for production of film due to its numerous advantages, such as low price, biodegradability, excellent ability to form film, high transparency, and exceptional gas barrier characteristics [1,5]. However, low mechanical and WV-BP of gelatin film limited its applications in food packaging [1]. In order to reduce moisture sensitivity, protein modification through physical and chemical treatments is required to conquer this problem [6].
Maillard reaction products (MRPs) have been gained interest for many decades. MRPs have been known to improve the bioactivity of food protein or peptides as well as sensory characteristics without using any chemical reagents [7]. The condensation of carbonyl and amines is the core of Maillard reaction [8,9]. MRPs had the ability to donate electrons, break radical chains, and chelate the metals [7]. Some factors including pH, type and concentration of saccharides or protein, as well as the heating condition, were reported to affect the formation of MRPs [6,7]. High pH is able to increase the proportion of deprotonated amino acids, leading to color development associated with antioxidant activity [10]. The rate of the browning reaction is also affected by the sugar types [10]. Reducing sugar’s reactivity was in descending order: aldopentoses > aldohexoses > ketohexoses > disaccharides [11]. Aldehyde group of acyclic aldoses showed higher electrophilicity than keto group of acyclic ketoses [12]. Since fructose possessed a higher fraction of open chain than glucose, the amino acid-fructose complex could be developed easily [13]. Recently, the MRPs have been implemented to increase the protein’s functionality for food packaging purposes [5]. Apart from the improvement of physical properties of film by chemical cross-linking, MRPs also serve as antioxidants, which are able to maintain the quality of foods [14,15]. Active films containing MRPs were used as packaging for food product. Kchaou et al. [16] used fish gelatin films comprising glucose to induce the MRPs formation at 120°C for packaging flaxseed oil for 21 days at 50°C, and Zheng et al. [17] applied fish skin gelatin/L-arabinose (FG-Ara) films comprising MRPs for packaging soybean oil at different storage temperatures (−17°C, 4°C, and 25°C). Furthermore, chicken skin is the by-product of the chicken processing industry that has nutritional value, and the dominant fatty acids in chicken skin oil (CSO) were oleic acid (43%), palmitic acid (27%), and linoleic acid (14%) [18]. Fatty acids, especially unsaturated fatty acids, in chicken skin oil are susceptible to lipid oxidation or rancidity. Thus, the application of antioxidative fish gelatin film containing MRPs as bag for packing chicken skin oil could maintain its quality during storage. However, no information on the use of MRPs based on fish gelatin-fructose as antioxidant and for substitution of glycerol in fish gelatin film exists. No application of developed films as bags for shelf-life extension of CSO have been documented. Therefore, the present study aimed to produce MRPs from the mixture of fish gelatin and fructose at different ratios to obtain high antioxidant activity and to monitor the quality changes of CSO packed in bag made from fish gelatin film containing MRPs during the storage at room temperature.
D-fructose was procured from Fluka (Buchs, Switzerland). All chemicals were acquired from Sigma-Aldrich Chemical Co. (St. Louis, MO, USA). Tilapia skin gelatin (~240 bloom) was purchased from Lapi Gelatine S.p.A (Empoli, Italy).
2.2 Preparation and Characterization of Maillard Reaction Products (MRPs)
Fish gelatin solution (3% protein concentration, w/v) was prepared by heating (70°C, 30 min) in a water bath. Fructose was added to gelatin solution at different protein/fructose ratios (1:1, 4:1 and 9:1, w/w). The mixture adjusted to pH 11 was transferred into a tightly capped bottle and kept in a shaking water bath (Memmert, Bavaria, Germany) at 60°C and 90°C with gentle agitation for 0, 12 and 24 h. Thereafter, the resulting MRPs solutions were cooled to room temperature (RT) and analyzed.
Measurement of pH of solutions was done with the aid of a pH meter (Sartorius PB-10, Göttingen, Germany).
2.2.2 DPPH Radical Scavenging Activity (DPPH-RS-A)
DPPH-RS-A was examined [10]. A standard curve of Trolox (10–60 µM) was prepared. The activity was computed and reported as µmol Trolox equivalents (TE)/mL sample.
2.2.3 UV-Absorbance and Browning Index
UV-absorbance and browning index of MRPs were measured at 294 and 420 nm, respectively [13].
2.3 Preparation of Fish Gelatin Film Containing MRPs
First, fish gelatin powder was dissolved in distilled water (70°C, 30 min) to obtain 3% protein (w/v). MRPs solution was produced with fish gelatin: fructose ratio of 1:1 (w/w) at 90°C and pH 11 for 24 h. Glycerol and MRPs solution mixtures at various ratios (30:0, 25:5, 20:10, 15:15, 10:20, 5:25, and 0:30 (w/w)) were incorporated into the gelatin solution at 30% (w/w, based on protein content). After stirring for 1 h at RT, film forming solutions (FFS) (4.00 ± 0.01 g) were poured onto a silicone resin plate (50 mm × 50 mm) and air-blown (12 h, RT). All samples were dried in an climate-controlled atmosphere at 25°C ± 0.5°C with relative humidity (RH) of 50% ± 5%. Dried films were peeled off manually and analyzed.
A micrometer (Mitutoyo, Model ID-C112PM, Mitutoyo Corp., Kawasaki-shi, Japan) was employed to determine the thickness of film. The thickness of 12 randomly chosen areas in film samples was obtained to calculate the average thickness.
Before measuring mechanical properties, the films were conditioned (48 h, 25°C, 50% ± 5% RH). Tensile strength (TS) and elongation at break (EAB) were assessed [18] using the Universal Testing Machine (Lloyd Instrument, Hampshire, UK) equipped with 100 N tensile load cell using cross-head speed of 30 mm/min. Ten samples (20 mm × 50 mm) with 30 mm of initial length were used for testing.
2.3.3 Water Vapor Permeability (WVP)
WVP of films was measured [18]. The films were placed to cover the aluminium cup, and a rubber gasket was used to fix the films tightly. The aluminium cups were then transferred into an environmental chamber (model H110K-30DM; Seiwa Riko Co., Tokyo, Japan) (25°C ± 0.5°C, 50% ± 5% RH). Over 10 h period, the cups were weighed every 1 h. WVP was determined by the following equation:
where w is the weight gain of the cup (g); l is the film thickness (m); A is the exposed area of film (m2); t is the time of gain (s); P2−P1 is the vapor pressure difference across the film (Pa).
A CIE colorimeter (Hunter associates Laboratory, Inc., Reston, VA, USA) was used to measure the color of the films using D65 (day light). A measuring cell having 1.25 inch opening was used. L* (Lightness/darkness), a* (redness/greenness) and b* (yellowness/blueness) were recorded. The total difference in color (ΔE*) was computed with the equation shown below [5]:
where ΔL*, Δa* and Δb* are the differences between the color parameter of the samples and those of the white standard (L* = 92.82, a* = −1.24, b* = 0.46).
2.3.5 Light Transmission and Opaqueness Value
Light transmission of films was determined at UV and visible range (from 200–800 nm) using UV–vis spectrophotometer (Shimadzu, Kyoto, Japan) [5]. Opaqueness value of film was computed:
where T600 is fractional transmission at 600 nm, and x is the thickness of film (mm).
2.4 Characterization of the Selected Films
Film containing glycerol/MRPs (10:20) showing the lowest WVP and high UV-light barrier properties and film containing glycerol/MRPs (0:30) showing the highest tensile strength were selected for characterization in comparison with the control film containing glycerol/MRPs (30:0).
Protein patterns of solubilized films were determined using sodium dodecyl sulphate–polyacrylamide gel electrophoresis. The prepared samples (15 mg protein) were loaded onto the gel (4% stacking gel and 10% separating gel). Constant current (15 mA/gel) was applied using Mini Protean Tetra Cell unit (Bio-Rad Laboratories, Richmond, CA, USA). Thereafter, the proteins separated in gel were stained and destained [7].
Film solubility was examined with a slight modification [18]. Conditioned films (width: 20 mm; length: 50 mm) were weighed and transferred into Erlenmeyer flask containing 10 mL of distilled water and 0.1% (w/v) sodium azide. The mixture was then agitated using a shaker (Heidolth Inkubator 10000, Schwabach, Germany) (250 rpm, 30°C, 24 h). After 24 h, the mixture was filtered using Whatman filter paper No. 1 to obtain the retentate. The retentate was dried (105°C, 24 h) and the dried insolubilized matter was weighed. Film solubility value was computed and reported as a percentage of total soluble matter weight relative to the weight of initial film.
2.4.3 Scanning Electron Microscopic (SEM) Images
SEM (Quanta 400, FEI, Eindhoven, the Netherlands) was used to visualize the surface and cross-section of the selected films [18]. Film samples were placed on bronze stubs and sputtered with gold (Sputter coater SPI-Module, PA, USA) to make them conductive before the morphological visualization. An acceleration voltage of 20 kV was employed. The magnification of 500× and 1000× were used for visualization of film surface and cross-section, respectively.
2.5 Quality Assessment of Chicken Skin Oil Packed in the Developed Bags Based on Fish Gelatin Containing MRPs During Storage
The selected films containing glycerol and MRPs at a ratio of 10:20 as well as LDPE film were used to prepared 3-side sealed bags. An impulse sealer with a magnet (Model ME-300HIM, S.N.MARK Ltd., Park, Nonthaburi, Thailand) was used to seal the films (90 mm × 90 mm) at 150°C ± 0.5°C for 2.5 s. The sealed films were then cooled for 3 s.
2.5.2 Preparation of Chicken Skin Oil
Chicken skin containing depot fat was used [19]. Chicken skins (20 mm × 30 mm) were rendered using a pan at 130°C–150°C for 10 min. The obtained oil namely CSO was filtered through two layers of cheesecloth and centrifuged at 3000 × g for 20 min.
2.5.3 Packing and Storage of CSO
CSO (4 g) was filled into the prepared bags from different films and headspace air was removed. Finally, the bags were heat-sealed. All samples were kept at 30°C ± 0.5°C with 52% ± 5% RH mediated by Mg(NO3)2 saturated salt [20] and kept away from direct light exposure. CSO samples were collected every 3 days for totally 15 days for quality assessment.
FFA content in CSO samples was measured [18]. Standard curve of palmitic acid in isooctane (10–50 mM) was prepared. FFA content was expressed as g FFA/100 g lipid.
PV in the CSO samples was determined using the method of Nilsuwan et al. [18]. Cumene hydroperoxide (0.5–2 ppm) was used to prepare a standard curve. PV was computed and reported as mg cumene hydroperoxide equivalent/kg CSO.
Thiobarbituric Acid-Reactive Substances (TBARS)
TBARS in CSO samples were examined using the procedure as mentioned by Manzoor et al. [21]. A standard curve encompassing 1,1,3,3-tetramethoxypropane (MDA) (0–10 ppm) was used. TBARS were computed and expressed as mg MDA equivalent/kg CSO.
Volatile compounds in CSO collected at day 0 and 15 of storage were analyzed by solid-phase microextraction gas chromatography mass spectrometry (SPME-GC-MS) [18]. The abundance of each individual compound was reported.
All experiments were run in triplicate using three different lots of samples. Analysis of variance (ANOVA) was employed to analyze the variance of data. The Duncan’s multiple range test [22] was used to compare the means. The Statistical Package for Social Science (SPSS 20.0 for windows, SPSS Inc., Chicago, IL, USA) was employed for statistical analysis.
3.1 Characteristics of Maillard Reaction Products (MRPs)
The pHs of MRPs solution produced under varying conditions are presented in Table 1. The pH of MRPs solution decreased as the Maillard reaction took place [7]. As reaction time was augmented, the continuous decrease of pH values was found for all MRPs solutions, regardless of temperature and gelatin-fructose (GE/FR) ratio used. The drastic decreases in pH were more pronounced for MRPs solutions after 12 h of reaction (p < 0.05). The lowest pH of MRPs solution was obviously obtained in all MRPs solutions after 24 h of reaction, compared to those of MRPs solution at 0 h. This result indicated that the Maillard reaction more proceeded with a rising reaction time [7]. Despite different GE/FR ratios, pH values between all MRPs solutions at the initial reaction time (0 h) were similar (p > 0.05). Differences in pH were attained after the heating was applied. At the same temperature and reaction time (except at 0 h), the lower pH (p < 0.05) was observed for MRPs solutions with GE/FR ratio of 1:1 (w/w), compared to those of MRPs solutions with other GE/FR ratios (4:1 and 9:1) (p < 0.05). The equal ratio between gelatin and fructose could provide the appropriate number of functional groups, which were mainly involved in the Maillard reaction as witnessed by the lowering of pH in resulting MRPs solution. Nevertheless, lower pH value was obtained for MRPs solutions prepared at 90°C, compared to those of MRPs solutions heated at 60°C, irrespective of GE/FR ratio and reaction time used (except at 0 h). This was suggested that a higher temperature used could accelerate Maillard reaction [10], which might be associated with high energy for glycation between fructose and α- or ε-NH2 of amino acids in gelatin. Lowering of pH of MRPs might be associated with the formation of organic acids such as formic acid, acetic acid, etc., mainly degraded from reducing sugars during Maillard reaction [23]. Additionally, the amine-amine reaction could be another important mechanism to form acidic products, resulting in the decreased pH of MRPs [24]. Therefore, the temperature, GE/FR ratio and reaction time had the profound impact on pH of MRPs solutions.
3.1.2 UV-Absorbance and Browning Index
The impact of heating temperature, GE/FR ratio and reaction time on the changes of UV-absorbance (A294) and browning index (A420) of MRPs solutions is shown in Table 1. Typically, A294 has been used for the measurement of the production of uncolored intermediate MRPs [10], whereas A420 represents the formation of the final browning product [13]. At the same temperature and GE/FR ratio, higher A294 were observed for MRPs solutions after 12 h of reaction. This result suggested that the formation of uncolored intermediate compounds was increased as reaction time upsurged, in which those intermediate compounds could serve as Maillard reaction’s precursor [10]. Regardless of GE/FR ratio and reaction time used, no difference in A294 (p > 0.05) between MRPs solutions produced at 60°C and 90°C was detected, except MRPs solutions with GE/FR ratio of 9:1 after 12 and 24 h of reaction. Lower A294 was found for those aforementioned MRPs solutions prepared at 60°C, compared to that of MRPs solutions prepared at 90°C, suggesting that the Maillard reaction occurred at a lower extent when lower level of sugar and temperature were used. Additionally, at the same temperature and reaction time used, no differences in A294 between MRPs solutions with GE/FR ratios of 1:1, 4:1 and 9:1 were observed, except A294 of MRPs solutions with GE/FR ratio of 9:1 after heating for 12 and 24 h. For the MRPs solutions after 12 h of reaction, higher values of A420 were obviously observed for MRPs solution heated at 90°C, compared to those of MRPs solutions prepared at 60°C (p < 0.05). Higher A420 values were also obtained when MRPs solutions were heated for longer time. A420 of MRPs solutions heated for 24 h were higher than those of MRPs solutions prepared for 0 and 12 h, irrespective of temperature and GE/FR ratio used, except A420 of MRPs solutions with GE/FR ratio of 1:1 heated for 12 h. In particular, the highest A420 value was observed for MRPs solution with GE/FR ratio of 1:1 heated at 90°C for 12 and 24 h. Therefore, temperature, GE/FR ratio, and reaction time had a direct influence on the formation of intermediate and final browning products in Maillard reaction.
3.1.3 DPPH Radical Scavenging Activity (DPPH-RS-A)
DPPH-RS-A of MRPs solution produced at various temperatures, GE/FR ratios and times is displayed in Table 1. In general, the highest DPPH-RS-A was found for MRPs solution heated at 90°C using GE/FR ratio of 1:1 for 24 h (p < 0.05). Xu et al. [25] documented that the higher pH of the reaction system provided a higher antioxidant activity of MRPs than the lower pHs used in amino acids-reducing sugars system. In addition, Chen et al. [26] found that higher temperature also increased antioxidant activity of MRP in gelatin hydrolysate-sugars model systems. DPPH-RS-A increased along with the rises of A294 and browning intensity (Table 1). This results were also documented by Benjakul et al. [10] and Karnjanapratum et al. [7]. In general, DPPH-RS-A of MRPs was related with the formation of intermediate and final products or browning intensity. According to Chen et al. [26], MRPs can act as hydrogen donors in antiradical activity. Apart from melanoidin, different MRPs such as furans, pyrroles, pyridines, and imidazoles might contribute to antioxidant potential during extended heating [27]. Therefore, DPPH-RS-A of MRPs produced from gelatin-fructose systems could be improved under the optimal MRP production condition.
3.2 Effect of MRPs on Properties and Characteristics of Fish Gelatin-Based Film
Film Appearance, Thickness, and Mechanical Properties
Photographs of films containing glycerol (GLY)/MRPs mixtures at varying ratios are depicted in Fig. 1A. Gelatin film without MRPs (30:0) was colorless and transparent. Brownish color was noticeable for all films incorporated with MRPs. Increasing brownish color was visually observed for the resulting films as higher level of MRPs was incorporated. The highest brownish color was obtained for the film containing GLY/MRPs at a ratio of 0:30. Film thickness increased with the addition of MRPs (Fig. 1B). Film with GLY/MRPs ratio of 0:30 showed the highest thickness (p < 0.05). Etxabide et al. [6] documented the increment of thickness in heat-treated fish gelatin-based film containing lactose. TS increased along with augmenting MRPs portion (p < 0.05) (Fig. 1C). Film with GLY/MRPs ratio of 0:30 showed the highest TS (p < 0.05). Tahsiri et al. [28] also found that tensile strength was improved with the addition of gum arabic in heat treated almond protein isolate-gum arabic based edible film. According to Zhang et al. [29], MRPs might increase interaction between polymer chains and result in denser film with higher tensile strength. Conversely, glycerol molecules with small size could weaken protein-protein interaction, resulting in lower TS of films [30]. According to the results, TS and EAB were inversely correlated (Fig. 1C,D). The rigid and strong film matrix was associated with the loss of flexibility or elasticity of film. Thus, higher proportion of MRPs could enhance TS but lowered EAB of resulting films.
Figure 1: Appearance (A), thickness (B), tensile strength (C), elongation at break (D) and water vapor permeability (E) of fish gelatin films incorporated with glycerol (GLY) and Maillard reaction products (MRPs) at different ratios. Different lowercase letters on the bars denote significant difference (p < 0.05). Bars represent standard deviation (n = 3)
Water Vapor Permeability (WVP)
WVP values were affected by GLY/MRPs ratios used in the film (Fig. 1E). The lowest WVP was obtained for film containing GLY/MRPs at a ratio of 10:20 (p < 0.05), while the highest WVP was attained as GLY/MRPs mixture (30:0) was added (p < 0.05). Generally, glycerol, which is a hydrophilic plasticizer that consists of three positions of hydroxyl groups (−OH), could bind with water in the plasticized protein [31]. Glycerol generally enhanced the hydrophilicity of films [30,31]. Also, the free volume in film matrix is enlarged in the presence of glycerol, resulting in the increased water diffusion in film matrix [31]. Film added with GLY/MRPs mixture (10:20) had the lowest WVP value. This might be due to the higher cross-linking between polymers when MRPs at the optimum concentration was added, resulting in the reduced free volume matrix in the film. In addition, Etxabide et al. [6] reported that MRPs formed in heat-treated gelatin-lactose-based edible films could decrease the polar group, thus increasing the hydrophobic character of films. When the excessive concentration of MRPs was added, the number of polar compounds in MRPs might contribute to high hydrophilicity of films. Consequently, diffusion of water vapor in film matrix at higher extent occurred. Therefore, mechanical properties and WV-BP of resulting films were influenced by levels of both glycerol and MRPs.
Color, Light Transmission and Film Opaqueness
Color parameters of gelatin films containing various GLY/MRPs ratios are shown in Fig. 2A–D. In general, when the ratio of MRP in GLY/MRP mixture increased, L*-value decreased along with upsurges in b* and ΔE* values (p < 0.05). For a*-value, the lowest value was attained in film added with GLY/MRPs mixture (15:15) (p < 0.05). Films having higher MRPs ratios turned to be more yellowish as noted by the augmented b*-value and darker color as witnessed by lower L*. It was suggested that the coloring pigments in MRPs plausibly contributed to the color of resulting film. The brown pigment from non-enzymatic browning reactions, such as melanoidin is formed during the interaction between amino group of protein chains and carbonyl group of reducing sugar [26]. Therefore, MRPs ratio noticeably affected the color of resulting gelatin films. Light transmission and opaqueness values of films with various GLY/MRPs ratios are depicted in Fig. 2E,F. Light transmission values decreased when the higher proportion of MRPs in the GLY/MRPs mixtures was added. In contrast, the increment of opaqueness values was observed, indicating that film became less transparent. At 200, 280 and 350 nm wavelengths, film added with GLY/MRPs ratio of 10:20, 5:25 and 0:30 showed excellent UV barrier properties toward light transmission. Films containing higher MRPs proportion were more capable of lowering light transmission, compared to film with a higher proportion of glycerol. The capability to prevent light transmission was associated with high film opaqueness (Fig. 2F). High film opaqueness was caused by the presence of brown pigments from MRPs [29]. Film with the greater glycerol proportion had the highest light transmission at all wavelengths measured. The light transmission in film was facilitated by glycerol that reduced the compactness of the film matrix [32]. Thus, levels of glycerol and MRPs played a significant role in color, light transmission and opaqueness of the resulting gelatin-based films.
Figure 2: Color parameters (A–D), light transmission (E) and opaqueness value (F) of fish gelatin films incorporated with glycerol (GLY) and Maillard reaction products (MRPs) at different ratios. Different lowercase letters on the bars denote significant difference (p < 0.05). Bars represent standard deviation (n = 3)
3.2.2 Characteristics of Selected Films
Film with GLY/MRPs ratio of 10:20 was chosen due to the excellent film properties, including lowest WVP (Fig. 1D) and high UV barrier properties (Fig. 2E). Properties of films with GLY/MRPs ratios of 30:0 and 0:30 were also examined.
The solubility of film containing the mixture of GLY/MRPs at ratios of 30:0, 10:20, and 0:30 are shown in Fig. 3A. Film with GLY/MRPs of 30:0 showed an excellent solubility in water than other films (p < 0.05). Film solubility in both films with GLY/MRP ratios of 10:20 and 0:30 became lower (p < 0.05). It might be associated with the presence of melanoidin, the insoluble polymeric compound formed at the final stage of Maillard reaction [5]. Additionally, the reduced solubility indicated the higher number of protein cross-links, which also reduced the moisture sorption ability in the resulting film [33]. The results suggested that MRPs potentially acted as a cross-linker in fish gelatin-based film system. Therefore, the ratio of GLY/MRPs affected film solubility.
Figure 3: Film solubility (A), protein patterns (B) and SEM micrographs (C) at the magnification of 500× and 1000× for surface and cross-section, respectively, of the selected fish gelatin films incorporated with glycerol (GLY) and Maillard reaction products (MRPs) at ratios of 30:0, 10:20, and 0:30. HM: High molecular weight marker. Different lowercase letters on the bars denote significant difference (p < 0.05). Bars represent standard deviation (n = 3)
Protein patterns of MRP solution as well as fish gelatin film added with GLY/MRPs at ratios of 0:30, 10:20, and 30:0 are presented in Fig. 3B. All fish gelatin films contained α1 and α2 [34]. For MRPs produced from gelatin and fructose, no α1 or α2 chains were retained. The results indicated that gelatin underwent degradation under alkaline condition at high temperature and it might be polymerized during Maillard reaction. Disappearance of α1 and α2 chains were noted in films added with only MRPs without glycerol (0:30). Additionally, the films containing only MRP (0:30), glycerol (30:0), and added with GLY/MRPs at 10:20 showed slight differences in the protein patterns. It indicated that bonds between MRPs, glycerol, and gelatin are easily interrupted by denaturing agent used for electrophoresis. Wang et al. [35] documented the similar protein patterns of fish gelatin glycosylated without and with galacto-oligogalactose with heating time less than 4 h. Thus, film properties could be governed by the interaction between MRPs, glycerol, and gelatin in film matrix.
Surface and cross-sectional images of film with GLY/MRPs ratios of 30:0, 10:20, and 0:30 are depicted in Fig. 3C. Film containing only MRPs (0:30) had the roughest surface and crack-free cross-section, compared to another two films. The intermolecular reaction plausibly occurred between high molecular MRPs and gelatin film, resulting in the denser and rougher surface in film containing high amount of MRPs. The dense structure of films incorporated with MRPs also indicated that MRPs had a potential to act as a cross-linker as proved by the increase of tensile strength with the addition of MRPs at higher level (Fig. 1C). Higher water vapor and UV-light barrier properties were also observed in films containing higher levels of MRPs, compared to that of control film (Figs. 1E and 2E). Film containing only glycerol (30:0) had higher crack, compared with other films. It might be related with the lower tensile strength of film without MRPs. Thus, the addition of glycerol produced porous structure and cracks, while the addition MRPs yielded a rough and dense structure of resulting films.
3.3 Quality Changes of Chicken Skin Oil Packed in Developed Bags during Storage
The efficacy of bag prepared from fish gelatin film incorporated with GLY/MRPs mixture having three different ratios to retard lipid deterioration in chicken skin oil (CSO) during 15 days of storage were investigated in comparison with LDPE bag.
3.3.1 Free Fatty Acid (FFA) Content
The changes in FFA content of CSO as affected by different types of bag are depicted in Fig. 4A. FFA content increased with the upsurge in storage time. FFAs were formed by hydrolysis of triacylglycerol or phospholipid, due to the presence of lipase, water, and excessive heating process [18]. The main factor affecting FFA increment in CSO packed in bags during storage was the water that possibly passed through the film or water present in the CSO during dry rendering. CSO packed in bag from gelatin containing glycerol alone (30:0) generally had higher FFA content compared to other samples (p < 0.05). Poor WV-BP of film was generally obtained when added with glycerol. Nilsuwan et al. [36] also reported the high water absorptivity of gelatin film added with glycerol compared to nylon/LLDPE film. In contrast, the changes in FFA contents between CSO packed in bag from gelatin incorporated with GLY/MRPs ratio of 10:20 and 0:30 as well as LDPE bag were not noticeably different from each other, especially from day 9 to day 15 of storage (p > 0.05). Therefore, packaging material with lower water vapor migration could be achieved when gelatin film was added with MRPs to slow down the hydrolysis of CSO during storage.
Figure 4: Changes in free fatty acid content (A), peroxide value (B) and thiobarbituric acid reactive substance value (C) of chicken skin oil packed in bags from gelatin films incorporated with GLY and MRPs at ratios of 30:0, 10:20, and 0:30 and LDPE during storage of 15 days at 30°C ± 0.5°C and 52% ± 5% RH. Bars represent the standard deviation (n = 3). Different lowercase letters on the bars in the same storage time denote significant difference (p < 0.05). Different uppercase letters on the bars in the same sample denote significant difference (p < 0.05)
Changes in PV of CSO packed in various bags during 15 days of storage are depicted in Fig. 4B. Although PV increased during storage times, the differences between samples were not obvious (p > 0.05), except for CSO packed in gelatin bag containing only glycerol (30:0), which had higher PV than others, especially within the initial 6 days of storage (p < 0.05). The gradual upsurge in PV might be caused by the presence of some oxygen in CSO that could be involved in the oxidation reaction in CSO to form the primary oxidation products, e.g., hydroperoxide. This finding is supported by Nilsuwan et al. [36], who documented that similar PV in shrimp crackers packed with glycerol-plasticized gelatin film and nylon/LLDPE were obtained after storage. For day 3 and 6, the CSO packed in the bag produced from film with GLY/MRPs at 10:20 had the lower PV than other samples packed in bag produced from film containing only glycerol. Furthermore, the presence of natural antioxidant from MRPs added into gelatin films regardless of its proportion in GLY/MRPs mixture did not significantly affect PV of CSO during storage. The water-soluble polymeric fractions of MRPs that have antioxidant potential could not be released effectively in CSO, a hydrophobic system [37]. Additionally, a slight decrease of PV was observed on day 12 of storage. As the temperature and light were controlled during storage, PV still increased in the presence of oxygen. Hydroperoxide formed at the earlier stages of oxidation underwent decomposition at the advanced stages of oxidation [38]. Minor reduction of PV on day 12 indicated the generation of secondary oxidation products, which negatively affected the quality of CSO.
3.3.3 Thiobarbituric Acid Reactive Substance (TBARS)
TBARS values of CSO were changed as affected by various types of bag during storage as depicted in Fig. 4C. TBARS value represents the amount of secondary oxidation products in CSO. Secondary oxidation products occurred even in the presence of oxygen at a low extent. TBARS value increased in all samples on day 3, suggesting secondary oxidation products were produced. Thereafter, TBARS values were fluctuated in the narrow range up to 15 days of storage. The highest TBARS value was attained in sample packed in LDPE bag. Higher lipid oxidation therefore occurred in the sample packed in LDPE bag. In contrast, lowest TBARS value was observed in CSO packed in gelatin bag containing GLY/MRPs of 10:20 (p < 0.05), especially during 9–15 days of storage. TBARS value of CSO packed in bag added with only MRPs was similar to that found in CSO packed in LDPE bag. Protein-based films are recognized to possess better O-BP than synthetic films [39,40]. The result indicated the necessity to develop a film with proper ratio of additives to improve the film properties. Thus, packaging material or bag that possessed an excellent oxygen barrier and antioxidative properties was important to retard lipid oxidation in CSO samples.
The volatile compounds of CSO packed in various types of bag after 15 days of storage in comparison with fresh CSO (day 0) are presented in Table 2. The fatty acid content in CSO is mainly dominated by unsaturated fatty acid, especially oleic (c18:1 n-9) and linoleic acid (c18:2 n-6) [18]. The unsaturated fatty acid content in CSO accounted up to 70% in 100 g of oil, which influenced the oxidative stability during storage [18]. Autoxidation occurred in the presence of oxygen, leading to the formation of hydroperoxides and subsequent decomposition to the secondary oxidation products such as aldehydes, ketones, and alcohol [41]. The presence of secondary oxidation products are often linked to off-odors and rancidity in oil [42]. Major compounds generated from the oxidation of oleic and linoleic acids, such as pentanal and hexanal were detected at day 0. Hexanal content raised up to 8 times after 15 days of storage in samples packed in all bags. During rendering in air and at high temperature, oxidation could be enhanced. Apart from hexanal, significant increment was also observed for other volatile aldehydes (pentanal, heptenal, 3-methylbutanal, 2,4-heptadienal) after 15 days. The linearity between PV and the amount of 2,4-heptadienal was previously reported [43]. In the present study, similar levels of 2,4-heptadienal in all samples were obtained. Relationship between PV and 2,4-heptadienal was also observed (Fig. 4B). Additionally, the least abundance of aldehydic volatile compounds was mostly observed in CSO packed in gelatin bag added with GLY/MRPs at 10:20 ratio. These results were coincidental with the lowest content of TBARS value in CSO packed in the bag containing GLY/MRPs ratio of 10:20 (Fig. 4C). For alcoholic volatile compounds, significant increases of 1-penten-3-ol and 3-methyl-1-butanol were observed after 15 days of storage. 1-octen-3-ol and 1-pentanol were also detected at low abundance. It demonstrated the oxidation of linoleic acid in the presence of single oxygen [18,43]. Among all samples, CSO packed in the gelatin bag containing only glycerol (30:0) had the highest alcoholic volatiles compounds. Ketonic volatile compounds that increased in most samples after storage were 3-pentanone, 3-hydroxy-2-butanone, and dihydro-2(3H)-furanone. CSO packed in gelatin bag containing the GLY/MRPs at a ratio of 10:20 contained the least abundance of ketonic volatile compounds compared to other samples. Overall, CSO packed in gelatin bag incorporated with GLY/MRPs at 10:20 possessed the lowest abundance of volatile compounds, indicating better oxidative stability compared to other samples after storage. The results were in tandem with the changes in FFA (Fig. 4A) and TBARS values (Fig. 4C). Thus, the gelatin bag with GLY/MRPs ratio of 10:20 was capable of retardation of the formation of secondary oxidation products and maintained the quality of CSO during storage.
MRPs derived from fish skin gelatin and fructose (1:1) at 90°C under alkaline condition (pH 11) had the highest DPPH-RS-A. The incorporation of glycerol and MRPs had an impact on the properties of fish skin gelatin film, depending on the ratio of GLY:MRPs used. Gelatin film added with GLY/MRPs at 10:20 ratio possessed the best mechanical properties in terms of tensile strength, water vapor barrier property, light transmission, as well as antioxidative activity. When the aforementioned film was used to make the bag, chicken skin oil (CSO) packed in the bag had the retarded quality loss as indicated by lower FFA, TBARS values, and volatile compounds after 15 days of storage. Therefore, fish gelatin film containing MRPs could be utilized as active packaging for edible oil susceptible to lipid oxidation. However, further improvement of film containing MRPs should be continued to improve the properties of film or bag for real application in lipids or fatty foods.
Acknowledgement: The authors would like to thank the International Center of Excellence in Seafood Science and Innovation, Faculty of Agro-Industry, Prince of Songkla University for the use of all facilities.
Funding Statement: The authors received no specific funding for this study.
Author Contributions: The authors confirm contribution to the paper as follows: study conception and design: Soottawat Benjakul, Thummanoon Prodpran; data collection: Krisana Nilsuwan, Yolanda Victoria Rajagukguk; analysis and interpretation of results: Krisana Nilsuwan, Yolanda Victoria Rajagukguk, Umesh Patil; draft manuscript preparation: Krisana Nilsuwan, Yolanda Victoria Rajagukguk. All authors reviewed the results and approved the final version of the manuscript.
Availability of Data and Materials: Data is contained within the article.
Conflicts of Interest: The authors declare that they have no conflicts of interest to report regarding the present study.
References
1. Lu Y, Luo Q, Chu Y, Tao N, Deng S, Wang L, et al. Application of gelatin in food packaging: a review. Polymers. 2022;14(3):436. doi:10.3390/polym14030436. [Google Scholar] [PubMed] [CrossRef]
2. Asgher M, Qamar SA, Bilal M, Iqbal HMN. Bio-based active food packaging materials: sustainable alternative to conventional petrochemical-based packaging materials. Food Res Intern. 2020;137:109625. doi:10.1016/j.foodres.2020.109625. [Google Scholar] [PubMed] [CrossRef]
3. Atta OM, Manan S, Shahzad A, Ul-Islam M, Ullah MW, Yang G. Biobased materials for active food packaging: a review. Food Hydrocoll. 2022;125:107419. doi:10.1016/j.foodhyd.2021.107419. [Google Scholar] [CrossRef]
4. Etxabide A, Kilmartin PA, Maté JI, Gómez-Estaca J. Characterization of glucose-crosslinked gelatin films reinforced with chitin nanowhiskers for active packaging development. LWT. 2022;154:112833. doi:10.1016/j.lwt.2021.112833. [Google Scholar] [CrossRef]
5. Etxabide A, Kilmartin PA, Maté JI, Prabakar S, Brimble M, Naffa R. Analysis of advanced glycation end products in ribose-, glucose- and lactose-crosslinked gelatin to correlate the physical changes induced by maillard reaction in films. Food Hydrocoll. 2021;117:106736. doi:10.1016/j.foodhyd.2021.106736. [Google Scholar] [CrossRef]
6. Etxabide A, Uranga J, Guerrero P, de la Caba K. Improvement of barrier properties of fish gelatin films promoted by gelatin glycation with lactose at high temperatures. LWT–Food Sci Technol. 2015;63(1):315–21. doi:10.1016/j.lwt.2015.03.079. [Google Scholar] [CrossRef]
7. Karnjanapratum S, Benjakul S, O’Brien N. Production of antioxidative Maillard reaction products from gelatin hydrolysate of unicorn leatherjacket skin. J Aquat Food Prod Technol. 2017;26(2):148–62. doi:10.1080/10498850.2015.1113221. [Google Scholar] [CrossRef]
8. Quan W, Li Y, Jiao Y, Xue C, Liu G, Wang Z, et al. Simultaneous generation of acrylamide, β-carboline heterocyclic amines and advanced glycation ends products in an aqueous Maillard reaction model system. Food Chem. 2020;332(26):127387. doi:10.1016/j.foodchem.2020.127387. [Google Scholar] [PubMed] [CrossRef]
9. Starowicz M, Zieliński H. How Maillard reaction influences sensorial properties (color, flavor and texture) of food products? Food Rev Int. 2019;35(8):707–25. doi:10.1080/87559129.2019.1600538. [Google Scholar] [CrossRef]
10. Benjakul S, Lertittikul W, Bauer F. Antioxidant activity of Maillard reaction products from a porcine plasma protein-sugar model system. Food Chem. 2005;93(2):189–96. doi:10.1016/j.foodchem.2004.10.019. [Google Scholar] [CrossRef]
11. Laroque D, Inisan C, Berger C, Vouland É, Dufossé L, Guérard F. Consideration of sugar reactivity. Food Chem. 2008;111(4):1032–42. doi:10.1016/j.foodchem.2008.05.033. [Google Scholar] [CrossRef]
12. Naranjo GB, Malec LS, Vigo M. Reducing sugars effect on available lysine loss of casein by moderate heat treatment. Food Chem. 1998;62(3):309–13. doi:10.1016/S0308-8146(97)00176-3. [Google Scholar] [CrossRef]
13. Ajandouz EH, Tchiakpe LS, Ore FD, Benajiba A, Puigserver A. Effects of pH on caramelization and Maillard reaction kinetics in fructose-lysine model systems. J Food Sci. 2001;66(7):926–31. doi:10.1111/j.1365-2621.2001.tb08213.x. [Google Scholar] [CrossRef]
14. Zhang Q, Li L, Lan Q, Li M, Wu D, Chen H, et al. Protein glycosylation: a promising way to modify the functional properties and extend the application in food system. Crit Rev Food Sci Nutr. 2019;59(15):2506–33. doi:10.1080/10408398.2018.1507995. [Google Scholar] [PubMed] [CrossRef]
15. Kchaou H, Benbettaieb N, Jridi M, Nasri M, Debeaufort F. Influence of Maillard reaction and temperature on functional, structure and bioactive properties of fish gelatin films. Food Hydrocoll. 2019;97(Supplement C):105196. doi:10.1016/j.foodhyd.2019.105196. [Google Scholar] [CrossRef]
16. Kchaou H, Jridi M, Nasri M, Debeaufort F. Design of gelatin pouches for the preservation of flaxseed oil during storage. Coatings. 2020;10(2):150. doi:10.3390/coatings10020150. [Google Scholar] [CrossRef]
17. Zheng Q, Zhang Q, Chen F, Yin L. Effect of heat sealing and storage on mechanical and barrier properties of Maillard modified fish skin gelatin/L-arabinose composite films. Coatings. 2022;12(12):1929. doi:10.3390/coatings12121929. [Google Scholar] [CrossRef]
18. Nilsuwan K, Arnold M, Benjakul S, Prodpran T, de la Caba K. Properties of chicken protein isolate/fish gelatin blend film incorporated with phenolic compounds and its application as pouch for packing chicken skin oil. Food Packag Shelf Life. 2021;30:100761. doi:10.1016/j.fpsl.2021.100761. [Google Scholar] [CrossRef]
19. Sheu KS, Chen TC. Yield and quality characteristics of edible broiler skin fat as obtained from five rendering methods. J Food Eng. 2002;55(3):263–9. doi:10.1016/S0260-8774(02)00100-0. [Google Scholar] [CrossRef]
20. Cho SY, Lee SY, Rhee C. Edible oxygen barrier bilayer film pouches from corn zein and soy protein isolate for olive oil packaging. LWT—Food Sci Technol. 2010;43(8):1234–9. doi:10.1016/j.lwt.2010.03.014. [Google Scholar] [CrossRef]
21. Manzoor S, Masoodi FA, Rashid R. Influence of food type, oil type and frying frequency on the formation of trans-fatty acids during repetitive deep-frying. Food Control. 2023;147:109557. doi:10.1016/j.foodcont.2022.109557. [Google Scholar] [CrossRef]
22. Steel R, Torrie J, Dicky D. Principles and procedures of statistics: a biometrical approach. New York, NY, USA: McGraw-Hill; 1986. [Google Scholar]
23. Liu S, Sun H, Ma G, Zhang T, Wang L, Pei H, et al. Insights into flavor and key influencing factors of Maillard reaction products: a recent update. Front Nutr. 2022;9:973677. doi:10.3389/fnut.2022.973677. [Google Scholar] [PubMed] [CrossRef]
24. Li X, Liu SQ. Effect of pH, xylose content and heating temperature on colour and flavour compound formation of enzymatically hydrolysed pork trimmings. LWT. 2021;150:112017. doi:10.1016/j.lwt.2021.112017. [Google Scholar] [CrossRef]
25. Xu H, Zhang X, Karangwa E, Xia S. Correlating enzymatic browning inhibition and antioxidant ability of Maillard reaction products derived from different amino acids. J Sci Food Agric. 2017;97(12):4210–8. doi:10.1002/jsfa.2017.97.issue-12. [Google Scholar] [CrossRef]
26. Chen K, Yang X, Huang Z, Jia S, Zhang Y, Shi J, et al. Modification of gelatin hydrolysates from grass carp (Ctenopharyngodon idellus) scales by Maillard reaction: antioxidant activity and volatile compounds. Food Chem. 2019;295:569–78. doi:10.1016/j.foodchem.2019.05.156. [Google Scholar] [PubMed] [CrossRef]
27. Kitts DD, Chen XM, Jing H. Demonstration of antioxidant and anti-inflammatory bioactivities from sugar-amino acid Maillard Reaction products. J Agric Food Chem. 2012;60(27):6718–27. doi:10.1021/jf2044636. [Google Scholar] [PubMed] [CrossRef]
28. Tahsiri Z, Mirzaei H, Hosseini SMH, Khalesi M. Gum arabic improves the mechanical properties of wild almond protein film. Carbohydr Polym. 2019;222:114994. doi:10.1016/j.carbpol.2019.114994. [Google Scholar] [PubMed] [CrossRef]
29. Zhang W, Azizi-Lalabadi M, Roy S, Salim SA, Castro-Muñoz R, Jafari SM. Maillard-reaction (glycation) of biopolymeric packaging films; principles, mechanisms, food applications. Trends Food Sci Technol. 2023;138:523–38. doi:10.1016/j.tifs.2023.06.026. [Google Scholar] [CrossRef]
30. Tarique J, Sapuan SM, Khalina A. Effect of glycerol plasticizer loading on the physical, mechanical, thermal, and barrier properties of arrowroot (Maranta arundinacea) starch biopolymers. Sci Rep. 2021;11(1):13900. doi:10.1038/s41598-021-93094-y. [Google Scholar] [PubMed] [CrossRef]
31. de Souza Silva R, Santos BMM, Fonseca GG, Prentice C, Cortez-Vega WR. Analysis of hybrid sorubim protein films incorporated with glycerol and clove essential oil for packaging applications. J Polym Environ. 2020;28(2):421–32. doi:10.1007/s10924-019-01608-7. [Google Scholar] [CrossRef]
32. Lim WS, Ock SY, Park GD, Lee IW, Lee MH, Park HJ. Heat-sealing property of cassava starch film plasticized with glycerol and sorbitol. Food Packag Shelf Life. 2020;26(1):100556. doi:10.1016/j.fpsl.2020.100556. [Google Scholar] [CrossRef]
33. Hager AS, Vallons KJR, Arendt EK. Influence of gallic acid and tannic acid on the mechanical and barrier properties of wheat gluten films. J Agric Food Chem. 2012;60(24):6157–63. doi:10.1021/jf300983m. [Google Scholar] [PubMed] [CrossRef]
34. Fan HY, Dumont MJ, Simpson BK. Preparation and physicochemical characterization of films prepared with salmon skin gelatin extracted by a trypsin-aided process. Curr Res Food Sci. 2020;3(1):146–57. doi:10.1016/j.crfs.2020.04.002. [Google Scholar] [PubMed] [CrossRef]
35. Wang Y, Wu C, Jia H, Mráz J, Zhao R, Li S, et al. Modified structural and functional properties of fish gelatin by glycosylation with galacto-oligosaccharides. Foods. 2023;12(15):2828. doi:10.3390/foods12152828. [Google Scholar] [PubMed] [CrossRef]
36. Nilsuwan K, Benjakul S, Prodpran T. Quality changes of shrimp cracker covered with fish gelatin film without and with palm oil incorporated during storage. Int Aquat Res. 2016;8(3):227–38. doi:10.1007/s40071-016-0138-x. [Google Scholar] [CrossRef]
37. McClements DJ. Emulsion ingredients. In: McClements DJ, editor. Food emulsions: principles, practices, and techniques. Boca Raton: CRC Press; 2004. p. 632. [Google Scholar]
38. Frankel EN. Lipid oxidation. Cambridge, UK: Woodhead Publishing; 2012. p. 488. [Google Scholar]
39. Sahraee S, Milani JM, Regenstein JM, Kafil HS. Protection of foods against oxidative deterioration using edible films and coatings: a review. Food Biosci. 2019;32:100451. doi:10.1016/j.fbio.2019.100451. [Google Scholar] [CrossRef]
40. Tyuftin AA, Kerry JP. Gelatin films: study review of barrier properties and implications for future studies employing biopolymer films. Food Packag Shelf Life. 2021;29:100688. doi:10.1016/j.fpsl.2021.100688. [Google Scholar] [CrossRef]
41. Kmiecik D, Fedko M, Siger A, Kulczyński B. Degradation of tocopherol molecules and its impact on the polymerization of triacylglycerols during heat treatment of oil. Molecules. 2019;24(24):4555. [Google Scholar] [PubMed]
42. Sharma A, Bhardwaj A, Khanduja G, Kumar S, Bagchi S, Kaur R, et al. Determination of hexanal using static headspace GC-FID method and its correlation with oxidative rancidity in edible oils. Food Anal Methods. 2022;15(10):2652–63. doi:10.1007/s12161-022-02320-4. [Google Scholar] [CrossRef]
43. Xu L, Yu X, Li M, Chen J, Wang X. Monitoring oxidative stability and changes in key volatile compounds in edible oils during ambient storage through HS-SPME/GC-MS. Int J Food Prop. 2017;20(sup3):S2926–38. doi:10.1080/10942912.2017.1382510. [Google Scholar] [CrossRef]
Cite This Article
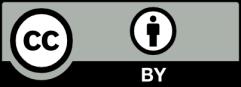
This work is licensed under a Creative Commons Attribution 4.0 International License , which permits unrestricted use, distribution, and reproduction in any medium, provided the original work is properly cited.