Open Access
ARTICLE
Fabrication of Core-Shell Hydrogel Bead Based on Sodium Alginate and Chitosan for Methylene Blue Adsorption
School of Material Engineering, Jinling Institute of Technology, Nanjing, 211169, China
* Corresponding Author: Xiaoyu Chen. Email:
Journal of Renewable Materials 2024, 12(4), 815-826. https://doi.org/10.32604/jrm.2024.048470
Received 08 December 2023; Accepted 18 February 2024; Issue published 12 June 2024
Abstract
A novel core-shell hydrogel bead was fabricated for effective removal of methylene blue dye from aqueous solutions. The core, made of sodium alginate-g-polyacrylamide and attapulgite nanofibers, was cross-linked by Calcium ions (Ca). The shell, composed of a chitosan/activated carbon mixture, was then coated onto the core. Fourier transform infrared spectroscopy confirmed the grafting polymerization of acrylamide onto sodium alginate. Scanning electron microscopy images showed the core-shell structure. The core exhibited a high water uptake ratio, facilitating the diffusion of methylene blue into the core. During the diffusion process, the methylene blue was first adsorbed by the shell and then further adsorbed by the core. Adsorption tests showed that the core-shell structure had a larger adsorption capacity than the core alone. The shell effectively enhanced the adsorption capacity to methylene blue compared to the single core. Methylene blue was adsorbed by activated carbon and chitosan in the shell, and the residual methylene blue diffused into the core and was further adsorbed.Graphic Abstract
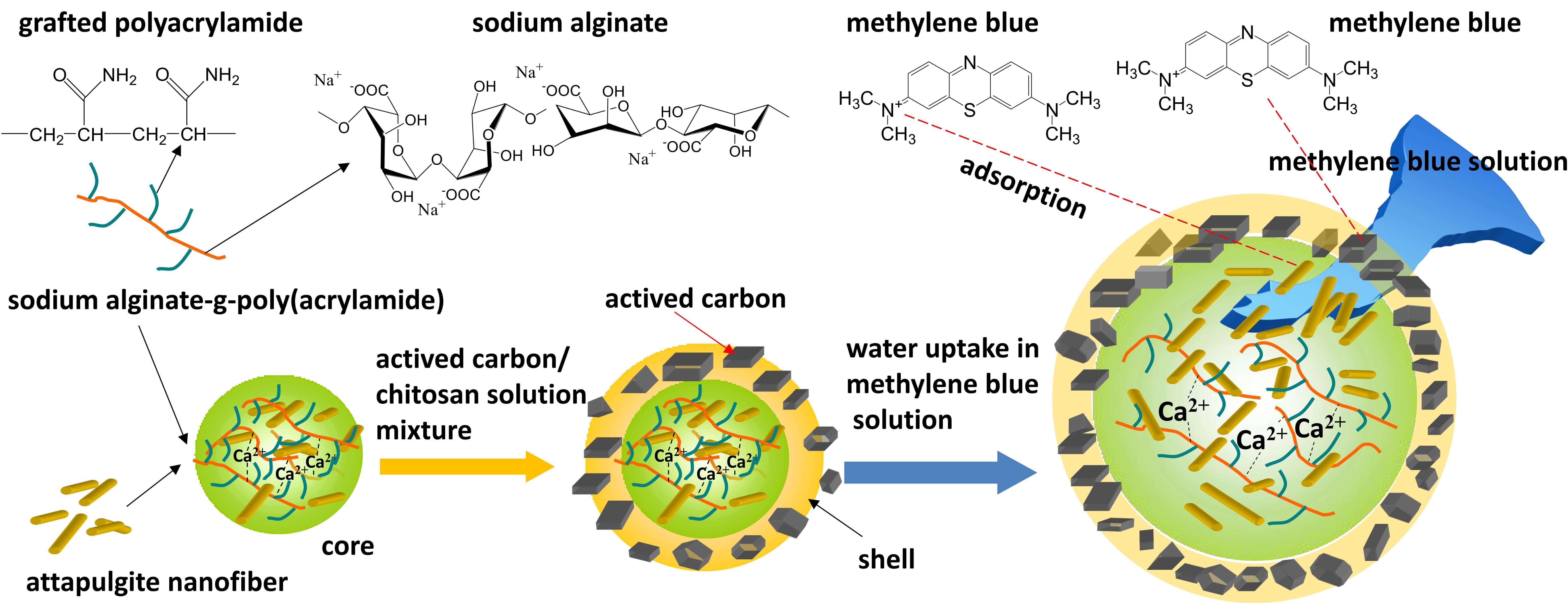
Keywords
The textile industry’s growth has led to a surge in dye-contaminated effluents, posing a significant environmental challenge. Among various water treatment methods, adsorption stands out as a cost-effective and facile approach. Hydrogels, due to their high water uptake ratio in dye solutions, have emerged as an effective adsorbent for dye removal. This property allows for easy diffusion of dye solutions into the hydrogel’s interior, where it is adsorbed by specific adsorption sites within the hydrogel’s structure [1,2].
Research has focused on natural macromolecules such as cellulose, starch, chitin, and sodium alginate for their non-toxic, water-absorbing, biodegradable, and cost-effective properties in dye adsorption applications [3–5]. The core-shell structure of hydrogel beads, with distinct structures and properties in both the core and shell, has been studied for its versatility in applications like drug delivery, adsorption, and environmental cleanup [6,7].
In drug delivery, sodium alginate is frequently used as a core or shell material due to its gelling ability with multivalent ions. Zhu reported a microsphere with a sodium alginate core incorporated with bioactive glass and a zein protein shell. The zein protein shell can protect bioactive glass passing through an acidic environment in the stomach and finally release it into the intestine [8]. Hydrogel beads were also created with an alginate core and carboxymethylcellulose shell as a drug delivery system. The carboxymethylcellulose shell enhances the mechanical properties of the alginate core and prevents drug leakage from the core [9].
Chitosan hydrogel beads with a uniformly distributed halloysite nanotube core and alginate shell have been developed for drug delivery [10]. The core-shell hydrogel beads were created for the purpose of orally delivering insulin directly to the intestine using a chitosan-coated layered double hydroxides/insulin complex core and alginate shell, produced by a simple dropping method [6]. Core-shell hydrogel capsules were fabricated with a core of diethylenetriamine-β-cyclo-dextrin/ethinylestradiol, aldocellulose, hydroxyethylcellulose, and a shell of sodium alginate for sustained release of ethinylestradiol in the intestine [11].
Hydrogel nanocomposites of β-cyclodextrin loaded with Vitamin E as core and soy soluble polysaccharide polymer network as shell were fabricated for release of Vitamin E [12]. Core-shell hydrogel beads with a mangiferin-loaded κ-carrageenan core and a chitosan shell were prepared using a one-step gelling method for mangiferin delivery [13]. The core-shell hydrogel capsules were produced, featuring a model drug core surrounded by a UV cross-linked poly(N-isopropylacrylamide) shell using a multi-material extrusion-based 4D printing method [14]. Additionally, core-shell hydrogel beads composed of a poly(N-acryloylglycine) core and a co-poly(N-acryloylglycinates) shell were prepared as pH/temperature-sensitive drug delivery vehicles [15].
In adsorption, a series of hydrogel beads with magnetic cores and adsorptive shells were developed. The Fe3O4/poly(acrylamide-co-sodium acrylate) core-shell magnetic nanocomposite hydrogels, measuring 11 nanometers in diameter, demonstrated exceptional sorption capabilities for methylene blue [16]. A magnetite nanoparticles core–crosslinked polyacrylic acid shell was fabricated to facilitate the elimination of methylene blue [17]. Core-shell beads with Fe3O4 nanoparticles cores and carboxymethyl cellulose cross-linked with poly(acrylic acid-co-acrylamide) shells were synthesized for the efficient removal of methylene blue from aqueous solutions through adsorption [18].
Graphene oxide core-polyethersulfone shell particles exhibit a high adsorption capacity as high as 352.11 mg·g−1 for methylene blue dye [19]. Chitosan hydrogel beads with a core-sodium dodecyl sulfate(SDS) shell show an enhanced malachite green removal due to “adsolubilization” of malachite green on SDS bilayer [20]. Polyvinyl alcohol nanoparticle core and poly(acrylamide-co-N-isopropylacrylamide) hydrogel shell nanogels were produced employing surfactant-free emulsion polymerization to efficiently eliminate Cu2+ from aqueous solutions [21]. Core-shell nanocomposite structures with metal oxide cores(TiO2, ZnO, TiO2–ZnO) and polyacrylamide shells showed good photodecolorization of organic dyes [22]. A polyetherimide core with a chitosan shell was fabricated for platinum metal ion recovery [23]. Core-shell bead with micrometer-sized sodium alginate beads and polyetherimide shell was used for Cr(VI) removal [24].
The beads, composed of a poly(acrylic acid) microgel core encapsulated by a polyethersulfone shell, exhibited exceptional adsorption capacity for methylene blue, reaching up to 84.82 mg·g−1 [25]. The water-soluble chitosan-poly(vinyl alcohol) core with ionic cross-linked hydrogel sodium alginate shell particles can adsorb carbon dioxide, lead ion, and copper ion [26]. The core-shell particles, consisting of a Cu2+ crosslinked carboxylated cellulose nanocrystals-carboxymethyl chitosan hydrogel sphere aerogel core and an MOF-199 crystal shell, demonstrate remarkable adsorption capacity towards methylene blue [27]. Wheat straw waste hydrogel sphere core with polypyrrole nanotubes shell shows high removal efficiency for water-soluble model anionic dye eosin Y [28]. A mechanically stable core-shell hydrogel bead has a Cu(II) removal capacity of 221 mg·g−1. Carboxymethylated cellulose nanofibril (shell) was immobilized on the surface of the sodium alginate hydrogel bead (core) via electrostatic attractions and hydrogen bonding [7].
Few reports exist on core-shell hydrogel beads with a high water uptake core and a high adsorption shell. The high water uptake core absorbs more dye solution, which diffuses into the core. During this process, the dye must pass through the shell and be adsorbed by the adsorption sites in the shell. The remaining dye solution then diffuses further into the core and is adsorbed by adsorption sites there.
Sodium alginate, a natural polymer extracted from seaweed, can be crosslinked by Ca2+ to form hydrogels. Grafting polymerization of acrylamide or acrylic acid to sodium alginate boosts the hydrogel’s water absorption capacity, facilitating the diffusion of more solution into the hydrogel and subsequent adsorption.
To enhance the adsorption capacity, various inorganic adsorbents, such as attapulgite nanofiber, sepolite, montalite, and rectorite, are integrated into the hydrogel. Attapulgite nanofiber is a magnesium aluminum silicate nanofiber with a high specific surface area and strong adsorption ability [29]. Hydrogels incorporating attapulgite nanofiber have been developed for dye removal from solutions [30].
Chitosan, a polysaccharide derived from chitin [31], has excellent film-forming properties and can form complexes with sodium alginate due to the opposite charges of the amine groups in chitosan and the carboxylate groups in alginate. Activated carbon is a commonly used high-performance adsorbent for dyes or heavy metal ions.
In this study, a novel core-shell hydrogel bead was fabricated using sodium alginate grafted with poly(acrylamide), attapulgite nanofiber, chitosan, and activated carbon. The hydrogel bead’s adsorption capacity and kinetic behavior were assessed for the common textile dye, methylene blue.
Attapulgite (Jiangsu Dianjinshi Au soil Mining Industry Co., Ltd., Xuyi, China). Sodium alginate (Shanghai Qingxi Chemical Technology Co., Ltd., Shanghai, China). Chitosan (degree of deacetylation >90%. Shanghai Lanji Science and Technology Company, Shanghai, China). Actived carbon (Liyang Zhuxi Actived Carbon Company, Liyang, China). N,N,N′,N′-Tetraethylethylenediamine (Sinopharm Chemical Regent Co., Ltd., Shanghai, China). CaCl2 (A.R. grade. Sinopharm Chemical Regent Co., Ltd., Shanghai, China). Methylene blue (methylthioninium chloride C16H18N3SCl. Tianjin Chemical Reagent Research Institute, Tianjin, China). Distilled water.
2.2 Fabrication of Core-Shell Hydrogel Bead
1.4 grams of sodium alginate were dissolved in 70 grams of distilled water, and 3.0 grams of acrylamide were added to form a mixed solution. Adding 0.08 grams of K2S2O4 and 100 μL of N,N,N′,N′-Tetraethylethylenediamine into above solution to initiate the grafting polymerization of acrylamide. The solution was stirred for 1 h at 25°C. Next, a certain amount of attapulgite powder was dispersed into the solution. The hydrogel beads were formed by dropping the resulting mixture into a 5% CaCl2 solution. The obtained hydrogel beads were kept in CaCl2 solution for 24 h. The beads were rinsed multiple times with distilled water to obtain the final product.
The mass ratios of (sodium alginate + acrylamide) to attapulgite were 1:0.5, 1:1, 1:1.5, 1:2, 1:2.5, and 1:3. Coordinate beads were labeled as C1, C2, C3, C4, C5, and C6, respectively. C designed the core. The sodium alginate-g-ploy(acrylamide) bead was coded as C0.
2.2.2 Fabrication of Core-Shell Hydrogel Bead
2 grams chitosan, 5 grams CH3COOH, and 95 grams H2O were mixed together and agitated until chitosan dissolve. 8 grams actived carbon powder was dispersed in the above chitosan solution. sodium alginate-g-ploy(acrylamide) beads were immersed into the above chitosan/actived carbon mixture and then were removed and placed in a 0.5M NaOH solution to cause chitosan to gel. This process created a chitosan/activated carbon layer on the surface of the sodium alginate-g-ploy(acrylamide) core. Finally, the prepared core-shell beads were freeze-dried. Core-shell beads with core of C1, C2, C3, C4, C5, and C6 were labeled as CS1, CS2, CS3, CS4, CS5, and CS6, respectively.
The structure of sodium alginate-g-ploy(acrylamide) was detected by Fourier transform infrared spectroscopy (FTIR) spectrometer (Thermo Fisher Nicolet iS10). The surface morphology of the core-shell hydrogel bead was observed by scanning electron microscopy (Apreo 2C Thermo Scientific, Shanghai, China).
2.4 Water Uptake Ratio Measurement
The freeze-dried core or core-shell beads were immersed in distilled water. After a certain period of time, the beads were taken out and weighed. The water uptake ratio, designated as W(t) at a given time t, was determined through the application of Eq. (1). In this equation, mi and mt correspond to the masses measured at the initial time and at time t, respectively.
2.5 Adsorption Capacity Measurement
0.1 grams of freeze-dried core or core-shell beads were submerged in 50 mL of a methylene blue solution (250 mg·L−1) at 25°C. After 72 h of adsorption, the absorbance of methylene blue solution was measured using a UV-Vis spectrophotometer (VARIAN Cary 50). The concentration of methylene blue was determined by absorbance using a standard curve of methylene blue. The adsorption capacity, designated as Q, was determined using Eq. (2).
where 250 is the initial concentration of methylene blue of the solution (mg·L−1), while the final concentration after adsorption was Ce (mg·L−1). The volume of the methylene blue solution was 0.05 (L), and the original weight of the beads was 0.1 grams.
0.1 grams CS1 were submerged in 100 mL of a methylene blue solution (250 mg·L−1) at 25°C. At certain time intervals, 0.5 mL solution was removed to measure its absorbance and determine the adsorption capacity of the CS1 beads, as previously described in Section 2.5. This process continued until the absorbance of the remaining solution reached a consistent value.
Fig. 1 shows the photos of prepared hydrogel beads. Sodium alginate is a biodegradable, non-toxic, and water-soluble linear polysaccharide extracted from brown seaweed. It consists of (1-4)-β-D-mannuronic acid (M) and (1-4)-α-L-guluronic acid (G) units [32]. The G units of sodium alginate can be easily cross-linked by divalent ions such as Ca2+, Ba2+ to form an egg-box structure [33]. This process creates a hydrogel in a short time. Sodium alginate hydrogel has found use in the adsorption of dyes, serving as an effective method for treating dye-contaminated wastewater [34].
Figure 1: Photos of prepared hydrogel beads. A: C0, B: C1, C: C2, D: C3, E: C4, F: C5, G: C6, and H: CS1
To achieve a core with a high water uptake ratio, hydrophilic -NH2 groups of acrylamide were introduced on the molecular chain of sodium alginate by grafting polymerization. K2S2O4 and N,N,N’,N’-tetraethylethylenediamine were used as oxidation-reduction radical initiators. Attapulgite nanofibers were dispersed in as-prepared sodium alginate-g-polyacrylamide solution to enhance the adsorption capacity of core. Chitosan mixed with actived carbon powder as a shell was used to adsorb methylene blue. Chitosan, a cationic polysaccharide, is soluble in acid solution but insoluble in basic solution. Based on the rapid phase inversion, the chitosan shell form around the core as soon as it was dropped into the NaOH solution, resulting in a core-shell structured hydrogel bead.
The morphologies of the freeze-dried CS1 are presented in Fig. 2. CS1 exhibits a core-shell structure, which is clearly visible in Fig. 2A. The core exhibits a porous structure, as shown in Figs. 2B and 2C. Attapulgite nanofibers are distributed on the porous core, as shown in Fig. 2D. CS1 exhibits a coarse surface due to the presence of a large amount of activated carbon powder, as seen in Figs. 2E and 2F.
Figure 2: Microphotograph and SEM micrographs of CS1: (A) microphotograph of split CS1(100×); (B) SEM micrographs of split CS1(100×); (C) core of CS1(300×); (D) attapulgite nanofibers in core (10000×); (E) surface of CS1(300×); (F) actived carbon on the suface of CS1 (3000×)
Fig. 3 shows the FTIR spectra of sodium alginate, acrylamide, and sodium alginate-g-acrylamide. In the spectrum of sodium alginate, the peaks observed at 1616 and 1427 cm−1 correspond to the anti-symmetric and symmetric stretch vibrations of the COO− group in sodium alginate, respectively. In the spectrum of sodium alginate-g-acrylamide, the peak at 3201 cm−1 is assigned to N-H stretching vibration [34]. The bands centered at about 3500 and 3200 cm−1 are due to the stretching vibration of -NH2 groups coupled with -OH band of sodium alginate at 3445 cm−1 [35]. The peak at 2935 cm−1 corresponds to the stretch vibration of –CH2–, which is generated by polymerization of acrylamide. The peaks at 1668 cm−1 correspond to the C=O stretch vibration of -CONH2. The peaks at 1610 cm−1 correspond to the N-H bending vibration [36]. The peaks at 1460 cm−1 correspond to the deformation vibration of -CH2- of grafted polyacrylamide. The peak at 1418 cm−1 represents the symmetric stretch vibration of the COO− group in sodium alginate. These findings suggest that acrylamide has been successfully grafted onto sodium alginate through polymerization.
Figure 3: FTIR spectra of sodium alginate, acrylamide, and sodium alginate-g-poly(acrylamide)
Fig. 4 shows the water uptake behavior of cores and core-shell beads with varying attapulgite nanofiber content. C0 (sodium alginate-g-poly(acrylamide) hydrogel bead) exhibits the highest water uptake ratio. As the attapulgite nanofiber content increases, the water uptake ratio decreases. The attapulgite nanofibers are physically trapped within the cross-linked structure formed by Ca2+ and sodium alginate–g–poly(acrylamide) molecules. An increased presence of attapulgite nanofibers establishes a structural impediment that prevents the diffusion of water molecules into the interior regions of the hydrogel bead, subsequently resulting in a reduced water uptake ratio.
Figure 4: Water uptake ratio of different cores (A) and core-shell hydrogel beads (B)
The core with a high water uptake ratio provides a driving force for solution absorption. The solution is forced to pass through the shell and is subsequently adsorbed by the attapulgite nanofibers within the core. During this process, the shell adsorbs methylene blue molecules with chitosan and activated carbon powder.
In Fig. 5A, the adsorption capacities of the core beads are displayed. As the content of attapulgite nanofiber increases, the adsorption capacity experiences an initial increase, followed by a subsequent decrease. C2 exhibits the highest adsorption capacity, while C5 and C6 display similar levels.
Figure 5: Adsorption capacities of core (A) and core-shell hydrogel beads (B) with different content of attapulgite nanofiber in 250 mg·g−1 methylene blue solution
The adsorption primarily results from the attapulgite nanofiber within the core. The negatively charged surface of attapulgite nanofiber electrostatically attracts cationic methylene blue molecules, leading to increased adsorption with increasing nanofiber content. However, a high nanofiber content decreases water uptake of the core bead, reducing the diffusion of methylene blue solution into the core bead. This decrease in diffusion results in fewer methylene blue molecules within the core bead, ultimately leading to a decrease in adsorption capacity.
Fig. 5B displays the adsorption capacities of core-shell hydrogel beads for methylene blue. In comparison to core beads, core-shell hydrogel beads exhibit higher adsorption capacity. CS1 has the highest adsorption capacity of 82.08 mg·g−1. For these core-shell beads, adsorption occurs in two stages: initial shell adsorption followed by core adsorption. Methylene blue molecules are initially adsorbed by chitosan molecules and activated carbon in the shell. Subsequently, these molecules diffuse into the core and are adsorbed by attapulgite nanofibers within the core.
The core not only adsorbs methylene blue molecules but also exerts an attractive force on the methylene blue solution due to water uptake. This force draws more methylene blue solution through the shell. As the solution passes through, methylene blue molecules within the solution are adsorbed by activated carbon and chitosan in the shell.
Fig. 6 illustrates the two-stage adsorption mechanism of the core-shell hydrogel bead for methylene blue. As the core absorbs water, it draws the methylene blue solution through the shell and into the core. In this process, the methylene blue molecules are adsorbed by activated carbon and chitosan in the shell. The remaining methylene blue can be further adsorbed by the attapulgite nanofibers in the core.
Figure 6: Water uptake drived two-stage adsorption mechanism of core-shell hydrgel bead to methylene blue
This adsorption style is rarely reported. According to previous reports on core-shell bead adsorption, most adsorption occurs at the shell. The core’s role is usually to provide supporting material for the shell or to reduce its cost. For example, alginate serves as a core to support a polyethylenimine shell [24]. PVA is used as a solid core to enhance the mechanical strength of PVA core-poly (acrylamide-co-N-isopropylacrylamide) shell beads [21]. Other reports have described cores with adsorption abilities, such as a graphene oxide core protected by a porous polyethersulfone shell [19].
In some research, the core serves a special purpose. For instance, Fe3O4, which has magnetic properties, can be easily separated from aqueous solutions using a magnet [16–18]. To enhance adsorption, TiO2 or ZnO cores with good photodecolorization abilities but low pollutant adsorption abilities are coated with polyacrylamide [22]. Table 1 lists the adsorption capacities of various core-shell beads for methylene blue.
The adsorption kinetics are analyzed using the intra-particle diffusion model, as expressed in Eq. (3):
In Eq. (3), Qt (mg·g−1) represents the adsorption capacity of methylene blue at time t (min). The intra-particle diffusion rate constant, Kid (mg·g−1·min−1/2), characterizes the diffusion rates of the adsorption process. C is a constant.
Fig. 7 reveals that the intra-particle diffusion model exhibits two-linearity, indicating that two distinct stages occur during adsorption. The initial linear segment (red line) represents a rapid stage, where methylene blue rapidly diffuses from the solution to the external surface of the shell [39]. The subsequent linear segment (blue line) represents a slower stage, where methylene blue molecules gradually diffuse into the core, describing the second adsorption stage.
Figure 7: Fitting curves of intra-particle model of CS1 for adsorption of methylene blue
A core-shell hydrogel bead compose of sodium alginate-g-poly(acrylamide)/attapulgite nanofibers and chitosan/activated carbon was successfully fabricated. Sodium alginate-g-poly(acrylamide) was successfully synthesized by grafting polymerization of acrylamide onto sodium alginate, and the resulting sodium alginate-g-poly(acrylamide) was crosslinked by Ca2+ in the presence of attapulgite nanofibers to form the core. A mixture of chitosan and activated carbon was then coated onto the core to create a core-shell bead. FTIR confirmed the successful grafting of polyacrylamide onto sodium alginate. SEM images showed a distinct core-shell structure. The core exhibits a high water uptake ratio, facilitating the diffusion of methylene blue into the core and its adsorption by the shell during the diffusion process. The core-shell hydrogel beads exhibit a good ability to adsorb methylene blue, reaching a maximum capacity of 82.08 mg·g−1.
Acknowledgement: The author acknowledges the School of Material Engineering, Jinling Institute of Technology for providing all the required infrastructure for carrying out this work.
Funding Statement: The author received no specific funding for this study.
Author Contributions: The only author confirms his overall contribution to the paper.
Availability of Data and Materials: The author confirms that the data supporting the findings of this study are available within the article or its supplementary materials (Suppl. Fig. S1).
Conflicts of Interest: The author declares that they have no conflicts of interest to report regarding the present study.
References
1. Sharma S, Sharma G, Kumar A, AlGarni TS, Naushad M, ALOthman ZA, et al. Adsorption of cationic dyes onto carrageenan and itaconic acid-based superabsorbent hydrogel: synthesis, characterization and isotherm analysis. J Hazard Mater. 2022;421:126729 [Google Scholar] [PubMed]
2. Yang YC, Zhu QL, Peng XW, Sun JJ, Li C, Zhang XM, et al. Hydrogels for the removal of the methylene blue dye from wastewater: a review. Environ Chem Lett. 2022;20(4):2665–85. [Google Scholar]
3. Ren J, Wang XM, Zhao LG, Li M, Yang W. Double network gelatin/chitosan hydrogel effective removal of dyes from aqueous solutions. J Polym Environ. 2022;30(5):2007–21. [Google Scholar]
4. Wang W, Hu JJ, Zhang RD, Yan C, Cui L, Zhu JJ. A pH-responsive carboxymethyl cellulose/chitosan hydrogel for adsorption and desorption of anionic and cationic dyes. Cellulose. 2021;28:897–909. [Google Scholar]
5. Munagapati VS, Wen HY, Gollakota AR, Wen JC, Lin KYA, Shu CM, et al. Magnetic Fe3O4 nanoparticles loaded guava leaves powder impregnated into calcium alginate hydrogel beads (Fe3O4-GLP@CAB) for efficient removal of methylene blue dye from aqueous environment: synthesis, characterization, and its adsorption performance. Int J Biol Macromol. 2023;246:125675 [Google Scholar] [PubMed]
6. Phan VG, Mathiyalagan R, Nguyen MT, Tran TT, Murugesan M, Ho TN, et al. Ionically cross-linked alginate-chitosan core-shell hydrogel beads for oral delivery of insulin. Int J Biol Macromol. 2022;222:262–71 [Google Scholar] [PubMed]
7. Chen KH, Qin FM, Fang ZQ, Li GH, Zhou J, Qiu XQ. Mechanically stable core-shell cellulose nanofibril/sodium alginate hydrogel beads with superior Cu(II) removal capacity. Int J Biol Macromol. 2022;222:1353–63 [Google Scholar] [PubMed]
8. Zhu YL, Wang YW, Xia GG, Zhang XR, Deng S, Zhao XY, et al. Oral delivery of bioactive glass-loaded core–shell hydrogel microspheres for effective treatment of inflammatory bowel disease. Adv Sci. 2023;10:2207418. [Google Scholar]
9. Yan MZ, Chen TT, Zhang SP, Lu T, Sun XM. A core-shell structured alginate hydrogel beads with tunable thickness of carboxymethyl cellulose coating for pH responsive drug delivery. J Biomater Sci Polym Ed. 2021;32(6):763–78 [Google Scholar] [PubMed]
10. Lisuzzo L, Cavallaro G, Parisi F, Milioto S, Fakhrullin R, Lazzara G. Core/shell gel beads with embedded halloysite nanotubes for controlled drug release. Coatings. 2019;9(2):70. [Google Scholar]
11. Gong FR, Jiang LH, Gao YN, Xu J, Wang T. Delivery of soluble ethinylestradiol complex by pH-responsive core-shell composite hydrogel capsules. J Appl Polym Sci. 2023;140(31):e54225. [Google Scholar]
12. Eid M, Sobhy R, Zhou PY, Wei XL, Wu D, Li B. β-cyclodextrin-soy soluble polysaccharide based core-shell bionanocomposites hydrogel for vitamin E swelling controlled delivery. Food Hydrocoll. 2020;104:105751. [Google Scholar]
13. Athipornchai A, Pabunrueang P, Trakulsujaritchok T. Mangiferin loaded carrageenan/chitosan core-shell hydrogel beads: preparation, characterization and proposed application. Food Hydrocoll. 2023;147:109394. [Google Scholar]
14. Zu S, Zhang ZH, Liu QP, Wang ZG, Song ZY, Guo YT, et al. 4D printing of core-shell hydrogel capsules for smart controlled drug release. Bio-Des Manuf. 2022;5(2):294–304. [Google Scholar]
15. Deng KL, Dong LR, Shi YE, Gou YB, Li Q, Wang SL. Drug release behaviors of a pH/temperature sensitive hydrogel bead with core-shelled structure. Adv Mater Res. 2011;148:1427–30. [Google Scholar]
16. Atta A, Akl MA, Youssef AM, Ibraheim MA. Superparamagnetic core-shell polymeric nanocomposites for efficient removal of methylene blue from aqueous solutions. Adsorpt Sci Technol. 2013;31(5):397–419. [Google Scholar]
17. Pooresmaeil M, Mansoori Y, Mirzaeinejad M, Khodayari A. Efficient removal of methylene blue by novel magnetic hydrogel nanocomposites of poly(acrylic acid). Adv Polym Tech. 2018;37(1):262–74. [Google Scholar]
18. Zhou YM, Li T, Shen JL, Meng Y, Tong SH, Guan QF, et al. Core-shell structured magnetic carboxymethyl cellulose-based hydrogel nanosorbents for effective adsorption of methylene blue from aqueous solution. Polymers. 2021;13(18):3054 [Google Scholar] [PubMed]
19. Zhou JK, Chen SQ, Xu S, Zhang X, Zhao WF, Zhao CS. Graphene oxide-based polyethersulfone core-shell particles for dye uptake. RSC Adv. 2016;6(104):102389–97. [Google Scholar]
20. Das D, Pal A. Adsolubilization phenomenon perceived in chitosan beads leading to a fast and enhanced malachite green removal. Chem Eng J. 2016;290:371–80. [Google Scholar]
21. Shoueir KR, Sarhan A, Atta AM, Akl MA. Adsorption studies of Cu2+ onto poly (vinyl alcohol)/poly (acrylamide-co-N-isopropylacrylamide) core-shell nanogels synthesized through surfactant-free emulsion polymerization. Sep Sci Technol. 2016;51(10):1605–17. [Google Scholar]
22. Morsi RE, Elsalamony RA. Superabsorbent enhanced-catalytic core/shell nanocomposites hydrogels for efficient water decolorization. New J Chem. 2016;40(3):2927–34. [Google Scholar]
23. Song MH, Kim S, Reddy DHK, Wei W, Bediako JK, Park S, et al. Development of polyethyleneimine-loaded core-shell chitosan hollow beads and their application for platinum recovery in sequential metal scavenging fill-and-draw process. J Hazard Mater. 2017;324:724–31 [Google Scholar] [PubMed]
24. Yan YZ, An QD, Xiao ZY, Zheng W, Zhai SR. Flexible core-shell/bead-like alginate@PEI with exceptional adsorption capacity, recycling performance toward batch and column sorption of Cr(VI). Chem Eng J. 2017;313:475–86. [Google Scholar]
25. Chen SQ, Zhang X, Huang H, Zhang M, Nie CX, Lu T, et al. Core@shell poly (acrylic acid) microgels/polyethersulfone beads for dye uptake from wastewater. J Environ Chem Eng. 2017;5(2):1732–43. [Google Scholar]
26. Dong YR, Xiao CM. Formation and cleaning function of physically cross-linked dual strengthened water-soluble chitosan-based core-shell particles. Int J Biol Macromol. 2017;102:130–5 [Google Scholar] [PubMed]
27. Zhang ZH, Hu J, Tian X, Guo FL, Wang CL, Zhang JM, et al. Facile in-situ growth of metal-organic framework layer on carboxylated nanocellulose/chitosan aerogel spheres and their high-efficient adsorption and catalytic performance. Appl Surf Sci. 2022;599:153974. [Google Scholar]
28. Huang F, Tian X, Wei W, Xu XL, Li JY, Guo YF, et al. Wheat straw-core hydrogel spheres with polypyrrole nanotubes for the removal of organic dyes. J Cleaner Prod. 2022;344:131100. [Google Scholar]
29. Liu YX, Zhong H, Li XR, Bao ZL, Cheng ZP, Zhang YJ, et al. Fabrication of attapulgite-based dual responsive composite hydrogel and its efficient adsorption for methyl violet. Environ Technol. 2022;43(10):1480–92 [Google Scholar] [PubMed]
30. Li Y, Liu SJ, Chen FM, Zuo JE. High-strength apatite/attapulgite/alginate composite hydrogel for effective adsorption of methylene blue from aqueous solution. J Chem Eng Data. 2019;64(12):5469–77. [Google Scholar]
31. Lin XX, Liu ZS, Chen RP, Hou Y, Lu R, Li S, et al. A multifunctional polyacrylamide/chitosan hydrogel for dyes adsorption and metal ions detection in water. Int J Biol Macromol. 2023;246:125613 [Google Scholar] [PubMed]
32. Wang YQ, Lu Y. Sodium alginate-based functional materials toward sustainable applications: water treatment and energy storage. Ind Eng Chem Res. 2023;62(29):11279–304. [Google Scholar]
33. Houben S, Pitet LM. Ionic crosslinking strategies for poly (acrylamide)/alginate hybrid hydrogels. React Funct Polym. 2023;191:105676. [Google Scholar]
34. İsmail O, Gökçe Kocabay Ö. Absorption and adsorption studies of polyacrylamide/sodium alginate hydrogels. Colloid Polym Sci. 2021;299(5):783–96. [Google Scholar]
35. Şolpan D, Torun M, Güven O. The usability of (sodium alginate/acrylamide) semi-interpenetrating polymer networks on removal of some textile dyes. J Appl Polym Sci. 2008;108(6):3787–95. [Google Scholar]
36. Zhou CJ, Lee S, Dooley K, Wu QL. A facile approach to fabricate porous nanocomposite gels based on partially hydrolyzed polyacrylamide and cellulose nanocrystals for adsorbing methylene blue at low concentrations. J Hazard Mater. 2013;263:334–41 [Google Scholar] [PubMed]
37. Sasaoka M, Kawamura A, Miyata T. Core-shell microgels having zwitterionic hydrogel core and temperature-responsive shell prepared via inverse miniemulsion RAFT polymerization. Polym Chem. 2022;13(23):3489–97. [Google Scholar]
38. Chen XY, Song XX, Sun YH. Attapulgite Nanofiber-cellulose nanocomposite with core-shell structure for dye adsorption. Int J Polym Sci. 2016;2016:2081734. [Google Scholar]
39. Zhou CJ, Wu QL, Lei TZ, Negulescu II. Adsorption kinetic and equilibrium studies for methylene blue dye by partially hydrolyzed polyacrylamide/cellulose nanocrystal nanocomposite hydrogels. Chem Eng J. 2014;251:17–24. [Google Scholar]
Supplementary Materials
Figure S1: Photos of prepared core-shell hydrogel beads. (A) CS2, (B) CS3, (C) CS4, (D) CS5, and (E) CS6
Cite This Article
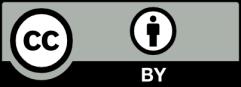
This work is licensed under a Creative Commons Attribution 4.0 International License , which permits unrestricted use, distribution, and reproduction in any medium, provided the original work is properly cited.