Open Access
REVIEW
Application of Plant-Based Coagulants and Their Mechanisms in Water Treatment: A Review
1 Higher Normal School of Constantine, Ali Mendjeli Nouvelle Ville, Constantine, 25000, Algeria
2 Laboratory of Process Engineering for Sustainable Development and Health Products (GPDDPS), Department of Process Engineering, National Polytechnic School of Constantine, Constantine, 25000, Algeria
3 Department of Environmental Engineering, Laboratoire de Recherche sur le Médicament et le Développement Durable (ReMeDD), University of Salah Boubnider Constantine 3, El Khroub, 25012, Algeria
4 Laboratory LIPE, Faculty Process Engineering, University of Constantine 3, Ali Mendjeli Nouvelle Ville, Constantine, 25000, Algeria
5 Laboratoire d’Etude et Recherche sur le Matériau Bois (LERMAB). Ecole Nationale Supérieure des Technologies et Industries du Bois (ENSTIB)—University of Lorraine, Epinal, 88000, France
* Corresponding Authors: Abderrezzaq Benalia. Email: ; Antonio Pizzi. Email:
(This article belongs to the Special Issue: Special Issue in Celebration of JRM 10 Years)
Journal of Renewable Materials 2024, 12(4), 667-698. https://doi.org/10.32604/jrm.2024.048306
Received 04 December 2023; Accepted 27 February 2024; Issue published 12 June 2024
Abstract
This review describes the mechanisms of natural coagulants. It provides a good understanding of the two key processes of coagulation-flocculation: adsorption and charge neutralization, as well as adsorption and bridging. Various factors have influence the coagulation/flocculation process, including the effect of pH, coagulant dosage, coagulant type, temperature, initial turbidity, coagulation speed, flocculation speed, coagulation and flocculation time, settling time, colloidal particles, zeta potential, the effects of humic acids, and extraction density are explained. The bio-coagulants derived from plants are outlined. The impact of organic coagulants on water quality, focusing on their effects on the physicochemical parameters of water, heavy metals removal, and bacteriological water quality, is examined. The methods of extraction and purification of plant-based coagulants, highlighting techniques such as solvent extraction and ultrasonic extraction, are discussed. It also examines the parameters that influence these processes. The methods and principles of purification of coagulating agents, including dialysis, freeze-drying, ion exchange, electrophoresis, filtration, and centrifugation, are listed. Finally, it evaluates the sustainability of natural coagulants, focusing on the environmental, technical, and economic aspects of their use. At the end of this review, the readers should have a comprehensive understanding of the mechanisms, selection, extraction, purification, and sustainability of plant-based natural coagulants in water treatment.Graphical Abstract
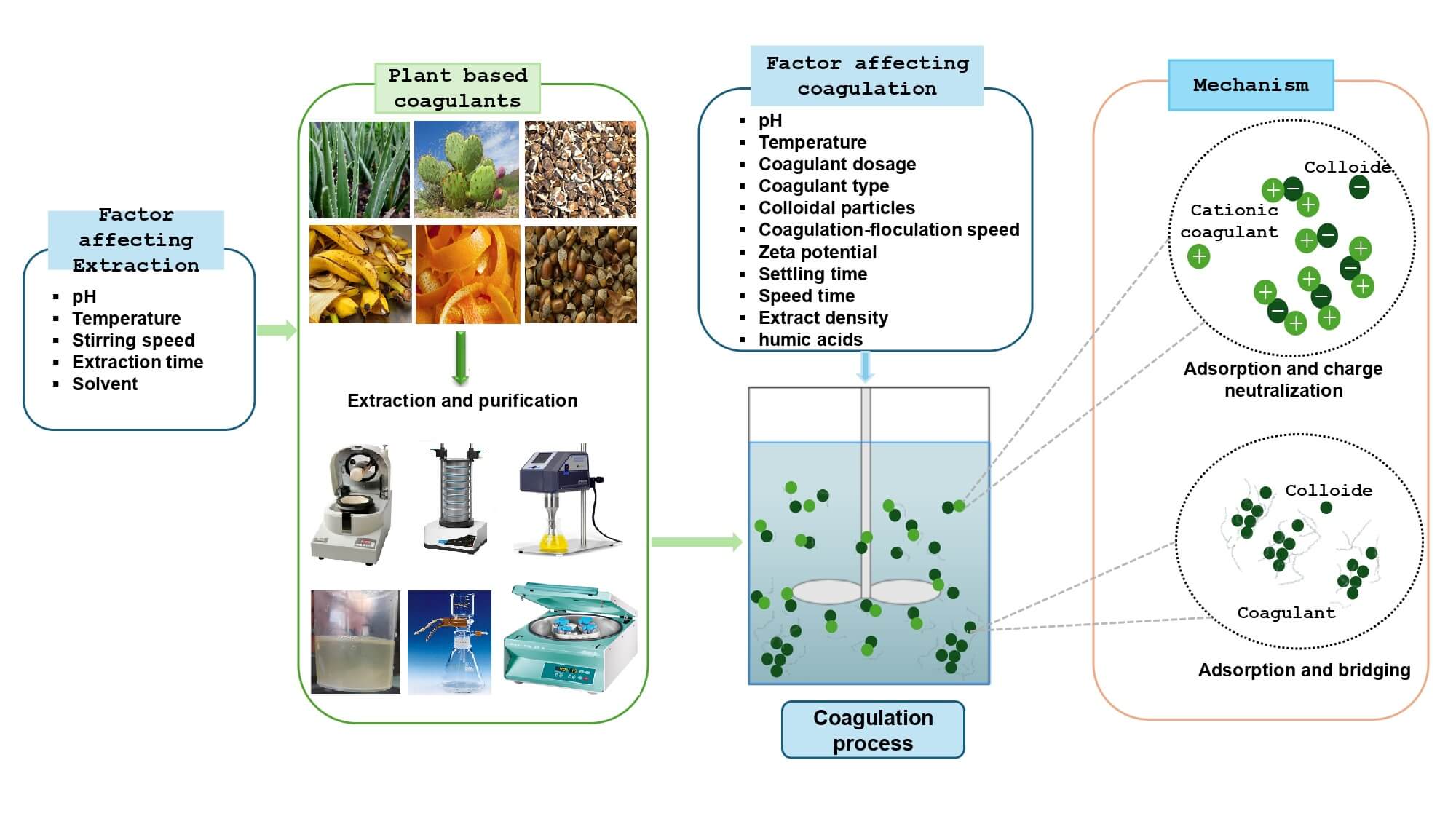
Keywords
Nomenclature
BOD5 | Biochemical oxygen demand after 5 days |
COD | Chemical oxygen demand |
DW | Distilled water |
K | Rate constant |
MO | Moringa oleifera |
NTU | Nephelometric Turbidity Unit |
OM | Organic matter |
P | Pressure |
Pa | Pascal |
R | Perfect gas constant |
Rpm | Revolutions per minute |
T | Temperature |
TA | Total alkalinity |
TDS | Total dissolved solids |
TH | Total hardness |
TSS | Total suspended solid |
Water is an essential element to sustain life and a vital raw material for energy generation, agriculture, industrial manufacture, and other various economic sectors [1]. However, the world’s water demand due to urbanization growth and population growth is on a steady decline trend. This is leading to water scarcity in many parts of the world, according to known forecasts [2,3]. By 2025, the United Nations predicts that almost half the globe’s inhabitants will reside in regions suffering from severe water scarcity. The scarcity of water presents a dual threat, not only to human health but also to food supply, economic development, and the environment [4,5]. In addition, industrial and agricultural pollution and domestic wastewater discharge negatively affect water quality. Such pollutants adversely affect water resources, so water pollution must be addressed as a key environmental issue [6]. Developing sustainable and economically viable water treatment methods is essential for addressing these challenges.
Coagulation-flocculation is a water treatment technique in which colloidal particles are destabilized by the addition of a coagulant. This process forms aggregates, which can then be effectively separated by sedimentation or filtration methods [7]. Coagulation and flocculation are favorable treatment techniques due to their cost-effectiveness, ease of use and effectiveness in removing water pollutants [3,8]. The efficiency and effectiveness of the coagulation process largely depend on the choice of coagulant and its type [9]. There are different types of coagulants: organic and inorganic. Inorganic coagulants, or inorganic salts, are generally a type of aluminum or iron salt [10]; the most widely used inorganic coagulants are iron and aluminum salts, due to their high pollutant removal efficiency, ease of use and low cost [9]. The use of these coagulants has many disadvantages, including the production of a high volume of sludge, the need to adjust alkalinity and pH and the high concentration of residual metals in the water [8,11]. The presence of aluminum in water can cause human diseases such as Alzheimer’s [12–14]. To address the problems associated with chemical coagulants, researchers have shown significant interest in the development of natural, environmentally friendly coagulants. The use of natural coagulants for water and wastewater clarification has been in use for many years in countries such as China, India and other African countries [9]. Using a natural coagulant involves agglomerating colloidal particles in the same way as chemical coagulants but producing a smaller quantity of biodegradable sludge, which can be used as fertilizer. In addition, pH and alkalinity adjustments are not required [15,16].
This review aims to explore the properties of natural organic coagulants and their effect on the physico-chemical and bacteriological parameters of water, as well as their durability.
This article aims to give an overview of the possibilities and challenging drawbacks due to bio-coagulant use so as to induce further research and development on these materials. The aim of this work is then to bring a contribution to the increasingly growing know-how on environment-friendly but also sustainable technology for the treatment of water to help to satisfy the ever-growing requirements for proper drinking water while simultaneously minimizing their impact on the environment.
2 Natural Coagulant Mechanisms
The main mechanisms of coagulation are four. These are double-layer compression, polymer bridging, neutralization of charge and scanning coagulation.
Generally, coagulation has been used to destabilize negatively charged colloidal particles. There are two types of colloidal particles: hydrophilic and hydrophobic. (1) Hydrophilic particles have a high affinity with water. These particles are surrounded by an envelope of water attached by physico-chemical bonds to certain groups in the material, such as -COOH, -NH2 or -OH. Hydrophilic colloidal particles are most often organic in nature. Such substances include natural organic matter. Hydrophilic colloids are more difficult to destabilize than hydrophobic solutions [5,17]. This is because the surrounding water molecules must first be acted upon to enable them to agglomerate. (2) Hydrophobic mineral colloids have no affinity for water. Their stability is due to the electric charge they carry, which creates a mutual repulsion that prevents them from agglomerating. They are generally mineral particles such as silica and clays. Few colloids are exclusively hydrophilic or hydrophobic. The difference lies in the presence or absence of a hydrophilic grouping, which induces a greater or lesser degree of hydration [3].
For natural coagulants only two of these are possible, namely (1) adsorption with neutralization of charge and (2) adsorption with polymer bridging [18,19].
2.1 Adsorption and Charge Neutralization
What happens in this mechanism is the adsorption of certain chemical species with opposite charges on the surface, which reduces the extent of the repulsive interaction caused by the double layer among other particles that are colloidal [19,20]. Destabilization by adsorption differs from destabilization by double-layer compression in that adsorbable species cause colloids to destabilize at a much lower dosage than non-adsorbable species. As such, destabilization by adsorption is stoichiometric, whereas the quantity of electrolyte required for double-layer compression coagulation is not stoichiometric and is practically independent of colloid concentration. This means that an overdose of an adsorbable species has the potential to destabilize the colloidal particles by reversing their charge.
Ions can occasionally be adsorbed to a charge reversal despite being beyond the point of charge neutralization as a result of chemical interactions that dominate over electrostatic repulsion effects [19,21].
Colloids are generally negatively charged. To neutralize this charge, positive ions are added to the water, forming a layer around the colloid [22,23]. Firstly, a layer of positive ions covers the entire surface of the colloid, ensuring the neutrality of the whole (adherent or fixed layer). The layer of positive ions is unevenly distributed around the colloid; neutrality is achieved at greater distances (diffuse layer), and finally the formation of a double layer, asserts that the first layer adheres to the colloid, where the potential rapidly decreases. The second layer is more diffuse, with slower potential decay [24].
A polymer capable of ionization is used as a coagulant in the mechanism by neutralization of charge to destabilize the colloidal particles. As these are charged negatively, they tend to repel each other.
Therefore, the particles are destabilized by the poly-cation, leading to a zeta potential near zero. The optimal dose of polyelectrolyte is necessary depending on its charge density. A lower addition dose of coagulant is thus correlated to a higher charge density [21].
The electrostatic correction mechanism (Fig. 1) means that areas of negative and positive charge exist on the particle surface. These charged areas induce further particles attraction of the particles to each other since the alignment of the opposite signs charges is correct [19,20,25].
Figure 1: Adsorption and charge neutralization
Coagulant properties are a feature of well-known natural compounds like cellulose, starch, proteins, and polysaccharides, alongside numerous synthetic polymers. These materials are characterized by their vast molecular mass and possess diverse electrical charges dispersed across their atomic chains. Polymer adsorption precedes polymer bridging. Polymer adsorption consists of the attaching of long-chain polymers to the colloidal particles’ surface due to their respective affinity for each other. Only some polymer parts are linked to the particle surface, the rest being unattached and forming tails and loops [20,26].
The bonding structure of the polymer is due to its loops and tails, allowing linkage of the colloidal particle to other ones of the same kind, thus forming larger flocs by aggregating them, as shown in Fig. 2.
Figure 2: Adsorption and bridging
Thus, there is a requirement for the particle surface to be unoccupied enough to allow an effective poly-surface area. Integration and transition need to be of sufficient scope to overcome interparticle repulsion. These requirements are then met by adding a polymer dose high enough via a natural coagulant to provide enough bridging links and unoccupied surface areas on the particle to allow good interparticle bridging [27,28].
After being destabilized, colloidal particles tend to agglomerate when they come into contact with each other, leading to an increase in floc size and a decrease in the number of particles in solution [3]. Flocculation can be caused by three mechanisms: (1) Peri-kinetic flocculation: contact between particles is caused by their random motion, known as Brownian motion; (2) Ortho-kinetic flocculation: this is caused by agitation of the water. Agitation facilitates aggregation by increasing the probability of collisions between particles, as fluid velocity varies in space and time. The relative movement of particles is due to the difference in density between them (sedimentation process) [29].
3 Factors Influencing the Coagulation Flocculation Process
A number of parameters influence the coagulation-flocculation process: pH, coagulant dose, coagulant type, temperature, initial turbidity, colloidal particles, coagulation time, flocculation time, coagulation and flocculation speed, settling time, Zeta potential, extract density and humic acids.
pH is a very important parameter for the optimal functioning of any mineral or natural coagulant [30,31], so determining its optimal value is necessary [32]. The nature of the medium (acid or base) has a significant impact on coagulant efficiency, as it can modify the surface charge of the coagulant and/or pollute it [15].
Several researchers have studied the effects of acidic and basic conditions on coagulant performance. For example, Usefi et al. investigated the effect of pH on the performance of the Moringa oleifera plant as a coagulant for textile wastewater treatment in terms of turbidity and color. In this study, the pH range was between 2 and 6 (acidic medium). The results obtained showed that pH 2 gave the best coagulant performance, with percentage reductions in turbidity and color at 81% and 77%, respectively [33].
Another study has shown that coagulant dosage is one of the most important factors taken into account when determining the optimum pH for coagulation flocculation. This study has also shown that for natural organic matter, acid pH facilitates their elimination through charge neutralization mechanisms [34].
Another study was carried out by Benalia et al. on the application of Aloe vera as a bio-coagulant in wastewater treatment with an initial pH variable between 2 and 12. The results obtained show that the maximum reduction in turbidity (99.13%), TSS (94.01%), organic matter (56.26%) and aromatic matter (88.34%) was obtained at pH 12 (basic medium) [35].
Musa paradisica (banana) peels can produce stable coagulants over a wide pH range and Dolichos lablab (Indian bean) seeds extract is typically more sensitive to pH changes [36]. Interestingly, the response of these coagulants to pH changes can vary, highlighting the importance of tailoring treatment conditions to the unique attributes of the coagulant used. Coagulants that exhibit stability over a wide pH range can be particularly advantageous, as they help to maintain a constant level of efficiency during the coagulation process, thus ensuring successful attenuation of water turbidity [36].
3.2 Effect of Coagulant Dosage
Coagulant dosage is an important parameter in the bio-coagulation process, as its effectiveness on the interaction between the coagulant agent and the colloidal particles present in the water [35,37,38]. The addition of coagulant reduced turbidity and other water parameters such as TSS (total suspended solids) and chemical oxygen demand (COD) [39,40]. This can be explained by the fact that the coagulant particles destabilize the negatively charged colloids present in the water to be treated, by neutralizing the charges that generate the repulsive forces between the colloids. An overdose of coagulant causes colloidal particles to re-stabilize and prevents the formation of inter-particle bridges, resulting in coagulant-laden water with poor clarification. Mineral coagulants, which are usually highly acidic, tend to lower the pH of the water. The optimum coagulant concentration has been determined in the laboratory using the jar test as an experimental technique [17].
When separating a liquid/solid mixture by coagulation-flocculation, coagulan’s choice is very wide and very important, as it has a major influence on the separation processes used (filtration, decantation, and flotation) and on minimizing the volume of sludge produced.
The type of coagulant, whether chemical or organic, affects coagulation flocculation in water treatment processes, and it can be used alone or in combination with other coagulants to increase the efficiency of the coagulation and flocculation process [34]. Chemical and organic coagulants differ in their role in stabilizing suspended particles and promoting floc formation. They have different effects on floc size and strength.
By way of illustration, chemical coagulants promote the formation of smaller, less robust flocs, while organic coagulants facilitate the creation of larger, stronger flocs [41].
On the other hand, the nature of the organic coagulants (fruits, leaves, etc.), their chemical structure, the nature of the coagulating agent (proteins, tannin, etc.) and the mechanisms by which they interact with the pollutants are essential in order to optimize the necessary processes [5].
Temperature is a very important factor in the coagulation-flocculation process [42,43], with low temperature resulting in increased water viscosity slowing down floc settling with low coagulant solubility [44]. According to Feng et al., low temperature had a significant negative effect on the rate of floc agglomeration [42].
Other studies have investigated the effect of temperature on the optimum pH range, i.e., with increasing temperature, the coagulation pH should be slightly increased. This is also linked to coagulation stoichiometry, which is also influenced by temperature. Results obtained by Guan et al. show that turbidity removal efficiency was improved by increasing water temperature in the pH range 6 to 9, where the maximum turbidity reduction was 66.20% and 76.06% for temperatures 10°C and 18°C, respectively [45].
We know that decreasing temperature slows down the rate of chemical reactions, according to the Arrhenius law between temperature and rate constant K [46]:
where A: frequency factor or pre-exponential factor;
Ea: activation energy (Joule);
R: perfect gas constant (Joule/mol.k);
T: temperature (k).
3.5 Effect of Initial Turbidity
Turbidity is also a parameter that affects the efficiency of coagulation flocculation [47], so increasing the concentration of colloidal particles must be followed by increasing the dose of coagulant used [17].
The influence of initial turbidity was also noted, since increasing initial turbidity increased coagulation efficiency [48]. Low-turbidity water is generally difficult to coagulate due to the low concentration of stable particles, and sometimes turbidity is artificially added (addition of clays) to the water to form heavier lumps that can stabilize [48].
Several studies have been carried out on the effect of initial water turbidity on coagulation function, for example, Odiyo et al. found that the highest coagulation efficiency was observed in waters with high initial loads (380 NTU) with a percentage removal of 71%, coagulation activity was reduced with decreasing initial turbidity (65 NTU), and in these cases the maximum reduction in turbidity was 34.3% [49]. Another experiment was carried out by Nkurunziza et al. It was observed that Moringa oleifera is not a good coagulant for raw water at low turbidity (50 NTU) compared to high turbidity (450 NTU), noting that the turbidity removal obtained was 88.06% and 99.80% for 50 and 450 NTU, respectively. Optimum dosages were 150 mg/L for 50 NTU and 125 mg/L for 450 NTU, indicating that the optimum dosage decreases with increasing turbidity leve (2016) classified turbidity two games: low and high according to their values (in NTU); high turbidity water with a value between 90 and 120 NTU, while low turbidity is between 20 and 35 NTU. In other words, when turbidity is less than (20–35) NTU, water has low turbidity, while water has high turbidity when the turbidity value is greater than (90–120) NTU [50].
Another study was carried out by Asrafuzzaman et al. on the application of bio-coagulants (Moringa oleifera, Cicer arietinum, and Dolichos lablab) for turbidity reduction in different ranges: (i) low: if turbidity is less than (25 to 35) NTU; (ii) medium: if turbidity between 40 and 50 NTU; (iii) high: if turbidity exceeds (25 to 35) NTU. The results obtained show that the bio-coagulants used work better with very turbid waters than with slightly or moderately turbid waters. For example, when Cicer arietinum was used as a coagulant, turbidity reduction efficiencies were 71.29%, 81.63% and 95.89% for low, moderate and very turbid waters, respectively [51].
3.6 Effect of Coagulation Rate
The coagulation rate is one of the most important factors in achieving better removal of pollutants from water during the coagulation-flocculation process [30].
After adding the coagulant to the water to be treated, rapid agitation is used to disperse the coagulant and homogenize the final solution (water-coagulant). Intense agitation prevents particle aggregation, whereas prolonged agitation breaks the bonds between the coagulant and the surface of colloidal particles, and the extended segments are subsequently folded back onto the particle surface [48].
In experiments carried out by Zheng et al., the effects of agitation speed between 50–600 rpm on turbidity and COD removal efficiency were investigated; in this study, at 100 rpm agitation, the removal efficiency was 99.5% and 73.5% for turbidity and COD, respectively [30].
3.7 Effect of Flocculation Speed
High-speed mixing and low-speed mixing are two important stages in the coagulation flocculation process. Rapid mixing promotes interaction between coagulant and suspended particles to form microflocs [41]. In other words, rapid agitation promotes uniform dispersion of the coagulant in the water, thereby increasing the risk of collisions between coagulant and colloidal particles. This accelerates the process of neutralizing the charges on the particles [52].
Slow mixing favors the agglomeration of microflocs to form larger flocs or macroflocs. Slow agitation is beneficial for the collision, adhesion and agglomeration of neutralized particles. Aggregated particles begin to form flocs, forming larger, heavier aggregates. This stage is important for water purification, as large flocs are more easily separated by sedimentation or filtration [52]. Muyibi et al. studied the influence of flocculation speed, in which the optimum gradient of slow mixing speed recorded was 20 and 40 rpm for low and high turbidity waters, respectively [48].
3.8 Effect of Coagulation and Flocculation Time
During the coagulation-flocculation process, adequate time must be provided to allow the formation of particles of sufficient size to enable their efficient removal in the settling process. Floc formation time (flocculation time) is one of the operating parameters of greatest concern for any treatment plant [34].
Several researchers have studied the influence of coagulation and flocculation time on turbidity removal efficiency [43,48,53].
Under optimized operating conditions, i.e., pH and coagulant dosage, the effect of settling time on coagulation-flocculation performance has been analyzed. The time at which turbidity and/or TSS levels become stagnant or minimal is considered the optimum settling time [54].
The study by Bhatia et al. showed the effect of settling time (30–150 min) on coagulation performance using Moringa oleifera as the main coagulant. In this study, the percentage of suspended solid removal was 90.09% for a time of 114 min [55]. In another study, the optimum settling time was selected on the basis of the wastewater-coagulant combination and the minimum residual turbidity obtained at the optimized dosage. The effect of sedimentation on residual turbidity was studied over a range of 30 to 360 min. Optimized settling times for turbidity reduction were observed at 240, 60 and 120 min for the combinations: dye-rich wastewater—Moringa oleifera, starch-rich wastewater—Moringa oleifera and textile industry wastewater—Moringa oleifera, respectively [54].
3.10 Effect of Colloidal Particles
From a dimensional point of view, there is an appropriate threshold for coagulation. For particles smaller than 1 micron, surface forces become dominant over mass forces. Under these conditions, a stable state of dispersion exists due to the combined effect of Brownian motion [35]. Similarly, the number of electrical charges on colloidal particles has been shown to have a significant effect on the coagulation process. This process is very difficult when colloid concentration is low, as the rate of inter-articular contact can be reduced [17].
The results obtained by Zhu et al. show that particle size distribution can be very useful in designing a suitable filtration treatment system to further treat pig slurry after the coagulation/flocculation and settling processes [53].
The zeta potential is a measure of the total surface charge of all particles and colloids present in water [56]. This potential has a maximum at the particle surface and decreases away from the surface, this decrease being influenced by the nature of the diffuse layer and the number and type of ions in the solution [56,57].
The Zeta potential is defined by the equation:
μ: dynamic viscosity of the liquid (Kg.
D: dielectric constant of the medium;
U: particle mobility (m/s).
According to Jefferson et al., most colloidal particles in natural waters are negatively charged, with a zeta potential between −14 and −30 mV. These surface charges give rise to repulsive forces that prevent aggregation and result in a stable system [58].
3.12 Effect of Extract Density
The results obtained by Hussain et al., clearly show that the optimum extract density was 1.8 g/cm3, with coagulant activity of 78% and 82% at initial turbidity of 67% and 75%, respectively. This implies that as extract densities increase from 1.2 to 1.8 g/cm3, the concentration of active substances in the coagulating extract also increases, maximizing coagulation activity. However, above 1.8 g/cm3, the coagulation activity of the extract begins to decline. It is possible that by further increasing density, black pine extract itself contributes to turbidity and ultimately leads to a reduction in coagulation yield [59].
Coagulation is markedly more affected by humic acids than by other organic compounds, and to remove them has focused a great deal of interest from researchers due to the potential for trichloromethane generation in the disinfection (chlorination) process [60].
Results obtained by Ramavandi confirm the capability of removing turbidity by humic acid when Plantago ovata was used as an organic coagulant. In this study a small amount addition of humic acid (0.5 and 1 mg/L) induced a moderate removal improvement of the initial water turbidity (100 and 300 NTU). Humic acids (41 mg/L) at relatively high concentrations (41 mg/L) lead to the formation of voluminous flocs, resulting in high water turbidity after coagulation treatment [61].
According to the literature, coagulation of turbidity-laden waters in the presence of low humic acid concentrations can be explained by several mechanisms: precipitation, charge neutralization, adsorption and bridging, etc. Mechanisms of a different nature, or combinations of them, may predominate under different conditions.
Due to humic acid’s high molecular concentration and high positive charge, the main role can be played by the combination of charge neutralization and bridging aggregation [28,62].
4 Types of Natural Coagulants of Plant Origin
The disadvantages associated with the use of mineral coagulants have motivated the search for non-toxic, biodegradable organic coagulants. In general, these appear to be more respectful of the environment both when being produced and in their applications. Historically, organic coagulants were used by rural women in Sudan, where they treated drinking water with Moringa oleifera powder seeds [63].
To date, research into natural plant-based coagulants has focused on four types: (1) seeds (Carica papaya and Beans), (2) fruit waste (peels, seeds) such as Mango and Banana peel, (3) fruits (Acorn, Chestnut) and (4) leaves (Cactus, Moringa and Eucalyptus).
A further classification of bio-coagulants is based on the active substance responsible for coagulation flocculation. Work carried out by various researchers confirms that the nature of coagulating agents can be: (i) proteins, (ii) polysaccharides, (iii) tannins, etc.
Background information and the physicochemical properties of various plant coagulants provide an overview of their properties and the active components responsible for coagulation [64].
Benalia et al. study shows the coagulating agents in Moringa seeds and Oak leaves is protein [39,65].
According to Choudhary et al., the coagulating agent is a polysaccharide for Cactus leaves, species of this plant are also used as natural coagulants as they contain certain compounds, such as L-rhamnose, D-xylose, D-galactose, and L-arabinose (see Fig. 3) [66], which are responsible for the bridging action. With contaminants in the water during the coagulation process.
Figure 3: Structural formula of compounds contained in Cactus leaves: L-rhamnose, D-galactose L-arabinose and D-xylose
Various studies on water treatment using tannins as coagulants have shown that the elasticity of tannins mainly depends on the chemical structure of the tannins extracted from plants and the degree of modification of the tannins [67,68], i.e., the chemical activation of tannins based on the Manich chemical reaction. The Mannich reaction applied to tannins involves the enolization of aldehydes or ketones, secondary amines, formaldehyde in aqueous hydrochloric acid as a catalyst, leading to the formation of high molecular weight tannin polymers with characteristic amphoteric activity [67,69].
As an example, the formation of coagulants from the reaction mechanism between the tannin extract and NH4Cl to form the coagulant can be reported in the following reaction [68]:
In addition, the presence of active functional groups in plants, namely hydroxyl (-OH), carboxylic acid (-COOH), CO, C and OH groups of carbohydrates, amines and amides, enhances the efficiency of the coagulation process.
Since 2015, several studies were carried out by our teams on the application of bio-coagulants in the fields of wastewater, drinking water and industrial water treatment, and several results were achieved, as indicated by the number of scientific productions (communications, publications, thesis and graduate student supervision) in Fig. 4.
Figure 4: Number of scientific productions by our teams in the field of water treatment using bio-coagulants, from 2015 to 2023
5 Effect of Organic Coagulants on Water Quality
Before treatment, polluted water may have varying physical, chemical and biological characteristics. To cope with these variations, the plant design may call upon several treatment processes [5]. In general, there are three types of water: (1) wastewater loaded with high COD, phosphate content, microorganisms, suspended solids, etc., (2) industrial waters loaded with heavy metals, and finally drinking water (groundwater and surface water) which are characterized by turbidity, TAC, TH, conductivity, salinity and bacteriological elements, etc. To eliminate the various water pollutants mentioned above, several chemical, physical and biological processes can be applied [70]. One of these processes is the coagulation/flocculation based on natural coagulants of plant origin [3,8].
5.1 Effect of Organic Coagulants on Water Physico-Chemical Parameters
Natural coagulants such as Cactus leaves, Moringa Oleifera, Banana, Fava bean, Pinus nigras, Oak leaves Aloe vera leaves, etc., have been used to reduce TSS, COD and turbidity in water, as well as their effect on total hardness and alkalinity (TH and TA), organic matter (OM) and conductivity (see Table 1). The removal efficiency of the various pollutants is considerably influenced by the coagulating agents of natural coagulants such as total phenolic, proteins, carbohydrates, starches, cellulose, etc.
5.2 Effect of Organic Coagulants on Heavy Metal Removal
The problem of reducing metal traces has become a sensitive one, as their activity is generally very low. However, certain metals that almost always accompany them, such as iron or manganese, can be tolerated at higher doses. Table 2 shows the Algerian standards for heavy metals in industrial water and drinking water.
Processes and techniques for treating wastewater containing heavy metals include adsorption, precipitation, flotation, ion exchange, chemical coagulation, microbial systems, electrochemical, membrane bioreactors and advanced oxidation processes [85,86]. The above-mentioned methods are classified into three main categories: (i) biological, (ii) chemical and (iii) physical. Treatment processes are used according to the type of metal, as each process has certain disadvantages and advantages shown in Table 3. This table also shows an example of the effect of the different processes mentioned above on the percentage removal of certain heavy metals from water.
Despite the good performance of the processes listed in Table 3, it is necessary to stress that choosing the most suitable process depends on a number of factors, such as raw water composition, metal concentration, capital investment and operating costs. However, for effective treatment, a sequence of several techniques is used for the removal of trace metals [100,101].
Heavy metals in water can be removed using a coagulation flocculation process, which uses coagulants to destabilize colloids and facilitate the settling process. During this process, several factors are optimized, namely pH and the coagulant dosage (iron or aluminum based) to reduce repulsive forces, increase attractive forces between particles and reduce various metallic elements in the water. This technique has drawbacks such as increased sludge volume, the production of aluminum- or iron-laden sludge, and the production of metal ions (Fe+3, Al+3, etc.) in treated water [102,103].
To avoid these problems, the use of bio-coagulants can be a better alternative than chemical coagulants, as it removes colloidal particles, produces only a small amount of sludge, and is biodegradable, so that the treated water is free of metals (iron and aluminum) [100,104].
The removal efficiency of trace metals from water is affected by: (1) the raw water characteristics (metal concentration and valence state of the heavy metal), (2) the coagulation conditions (pH, temperature, etc.), (3) the coagulating agent type, and the method of extraction of the coagulating agents [105].
Several researchers have proposed a coagulation flocculation technique using bio-coagulants (Moringa oleifera, Cactus, Banana, etc.) to remove the undesirable heavy metals zinc, lead, iron, nickel and copper (see Table 4).
5.3 Effect of Organic Coagulants on Water Bacteriological Parameters
The water must not contain pathogens, micro-organisms or viruses likely to cause biological contamination and epidemics. The presence of total and fecal coliforms or total and fecal streptococci indicates fecal contamination of the water. The presence of other coliforms, such as Clostridium, indicates this type of contamination. In both cases, measures must be taken to prohibit the use of the water or ensure its treatment.
To eliminate the various types of micro-organisms, water must be disinfected with a variety of disinfectants before it is used as drinking water.
This is done by adding an active antimicrobial agent to the water, which reacts with the bacteriological elements present in the water.
Many biomaterials are used as organic coagulants with an effect on the bacteriological quality of drinking water, i.e., elimination of the various types of bacteria and viruses present in water, such as coliforms, streptococci, Escherichia coli, etc.
Moringa oleifera is considered an environmentally friendly alternative to commonly used disinfectants. Suarez et al. identified and characterized a cationic polypeptide in Moringa oleifera seeds that eliminates turbidity from water. In addition, it was found to have antibacterial activity, capable of eliminating many waterborne pathogens from water [113]. Brodin et al. have eliminated bacteria in water treatment using proteins of Moringa oleifera as a flocculant [114]. Madsen et al. observed 90%–99.99% bacterial removal with Moringa oleifera as a bio-coagulant in water treatment traditionally used in Sudan [115]. Ghebremichael et al. tested the antibacterial properties of proteins of Moringa oleifera for drinking water purification as coagulants and flocculants combined with aluminum sulfate, in this study the percentage of Escherichia coli elimination was around 65% [116]. According to Poumaye et al., the Moringa oleifera-based coagulant eliminated 95%, 62% and 47% of clostridium, streptococci and Escherichia coli, respectively [117], on the other hand, using Carica papaya as an organic coagulant reduced the number of E. coli in treated water by around 88% [118].
6 Extraction and Purification of Coagulating Agents from Plants
Solid-liquid extraction is a material transfer operation between a phase containing the “solid” raw material to be extracted and a “liquid” extraction solvent. The aim of this technique is to extract the components mixed with the solid (the coagulating agents) into the solvent [119]. The solid-liquid extraction process is governed by three basic mechanisms: penetration of the solid by the extractor, diffusivity of the analyte in the external space and solubility of the analyte in the extractant [15,120].
Several techniques are available for extracting coagulants. These include ultrasonic extraction, solvent extraction (organic and inorganic), and several protocols for extracting proteins, sugars, polyphenols, etc., which are responsible for coagulation-flocculation processes. However, none of them is considered a standard method, as the effectiveness of these techniques depends on the individual case. For example, the result of each extraction may vary according to the input parameters (pH and temperature), the nature of the solvent, the plant raw material, and the desired product extracted (coagulant agent) [39,121].
Solvent extraction is a selective approach primarily based totally at the solubility of the species to be extracted (proteins, polyphenols, polysaccharides, etc.) in a given solvent. The solubility of coagulants in a solvent depends on its polarity, the nature of the coagulant, the solvent used and the concentration of the solvent. There are three traditional solvent extraction methods for coagulants (distilled water and acid, etc.): [122]. Coagulating agents can be extracted using three traditional techniques, using specific solvents (distilled water and acid, etc.): (i) Infusion: This involves pouring hot water over the flowers, leaves or herbs (stems) of the chosen plants. The extract-rich solution is then left to stand for a few minutes; (ii) decoction: plant stems or roots are boiled in water for a few minutes, to soften them and extract the active ingredients; and (iii) maceration: plant flowers, barks or roots are left to soak in oil, alcohol or water at room temperature for several hours [39].
Ultrasonic extraction can be performed using an ultrasonic bath or an ultrasonic probe. The ultrasound-assisted extraction mechanism involves the destruction of plant tissue by ultrasonic waves, which propagate through plant cells in the form of mechanical vibrations, attacking compression and expansion cycles during the extraction process. These mechanical effects lead to improved extraction rates and prolonged mass change through cell wall degradation, cell content release and increased solvent penetration into the plant material.
The use of ultrasonic assistance during coagulant extraction can reduce extraction time and achieve comparable turbidity removal percentages compared to traditional extraction methods. However, from an ecological factor of view, the conventional extraction procedure is much less pricey than the ultrasound-assisted extraction process, because it calls for much less power to operate [123].
6.2 Factors Influencing the Extraction of Coagulating Agents
Several factors have been shown to influence the extraction rate of coagulating agents, namely temperature, pH, reaction rate, concentration and nature of the solvent, contact time between plant material and solvent, and liquid/solid ratio (solvent/plant). It is, therefore, important to correctly identify and select the parameters that influence the coagulating agent’s extraction.
The extraction of coagulating agents is affected by temperature [39]. At low temperatures, their activity is inhibited, structural modifications are discrete and denaturation is reversible. Although hydrogen bonds are broken at high temperatures (cooking, sterilization, etc.), coagulants cannot restore their native conformation and denaturation is irreversible [124,125].
In general, coagulant extraction efficiency is better at very alkaline pH than at acid pH. In fact, a very alkaline pH can lead to dissociation and degradation of the coagulant. The aim of solubilization is to transfer most of the coagulating agents from the plant’s raw material to the aqueous phase. As an example, proteins possess a neutral charge at pHi (the isoelectric pH). A strong electrostatic attraction between ionized groups in proteins at this pH favors the denature of these macromolecules [124].
6.2.3 Effect of Stirring Speed
Agitation homogenizes the medium in terms of composition and temperature.
6.2.4 Effect of Extraction Time
The added coagulant powder is left in the solvent after the agitation and homogenization stages, to allow extraction to take place. Maceration time influences the extraction process: the quantity of coagulant increases with the extraction time, and the maximum concentration is obtained at a given time, which is the optimal maceration time [126]. This increase can be justified by the increased solubilization of the coagulant contained in the plant [17].
6.2.5 Effect of Solvent on Coagulant Agents Extraction Yield and on Coagulation
The use of different solvents has been reported in diverse investigations for extracting natural coagulants. Distilled water (H2O) extraction is clearly the best choice in terms of availability and cost-effectiveness [127]. To improve extraction efficiency, several solutions have been used in the extraction process, such as salt solutions (NaCl, KCl, BaCl2, MgCl2) [77,128–131], acids and bases [77]. The efficiency of turbidity removal is considerably influenced by coagulants. Table 5 reveals the influence of certain organic coagulants on turbidity reduction achieved through different extracting agents.
Different solutions have noticeable and varied effects. Improvement in the coagulation activity of the extract can be demonstrated by increasing the concentration of coagulating agents. For example, when using salt, this improvement is due to the increase in salt concentration, demonstrated by the Debye-Hacker theory (increased dissolving power), where low salt content increases the solubility of coagulants in water [132]. High salt concentrations can reduce the solubility of coagulants in water, a phenomenon known as the salting-out effect, explained by John Gamble Kirkwood’s principle of decreasing solvent power [132]. The salting-out effect can also occur due to the dehydration of coagulating agents by the presence of excess salt [133]. A slight increase in ionic strength, as observed by Megersa et al., can disrupt protein-protein bonds in Moringa oleifera, leading to enhanced solubility and improved coagulation activity [127].
7 Purification Methods for Coagulating Agents
Previous research has identified various methods for purifying coagulating agents, including: ion exchange [61], dialysis [78], lyophilization [140], filtration [123,141] and centrifugation [140], etc. All of these techniques have proven effective in purifying extracts and can be incorporated into a scale-up facility for water treatment [142].
Dialysis is a laboratory technique commonly used to purify, concentrate, or fractionate macromolecules such as proteins, carbohydrates, etc. This process desalinates the solution by removing or reducing its salt content. Semi-permeable membranes are used to isolate active target substances (proteins, sugars, polyphenols, etc.) [143]. The solution containing the target substance is enclosed in a sealed dialysis tube and immersed in a larger vessel filled with pure water as present in Fig. 5.
Figure 5: Dialysis principle
The porous nature of the dialysis membrane allows smaller molecules, primarily salts and other low-molecular-weight species, to freely equilibrate across the concentration gradient, whereas larger molecules like sugars are effectively sieved out and retained within the dialysis bag [144].
This technique was employed to purify Moringa oleifera coagulant agent, i.e., the supernatant was dialyzed in the dialysis bag for 24–36 h against distilled water until the precipitate was visualized [78].
Lyophilization, or cold drying, is a process that removes water or other solvents from a product, making it stable at room temperature and thus facilitating preservation. This process uses the phenomenon of sublimation to preserve the product’s original appearance. Solvents contained in the product (such as H2O), undergo a direct phase change from solid to gas, bypassing the liquid state [144].
According to Grossmann et al. freeze-drying was carried out to remove water. The different parts of the coagulant solution were freeze-dried using a freeze-dryer. Firstly, The samples were dried at T = −10°C and p = 10 Pa for 24 h. To remove the adsorbed water, the temperature was increased to T = 20°C for another 24 h and the pressure was decreased to p = 1 Pa to obtain a freeze-dried soluble protein extract [140].
Ion exchange primarily uses synthetic materials, or ion exchangers, as adsorbents to adsorb valuable ions.
The classic ion exchanger in the biological industry is ion exchange resin. Ion exchange methods have advantages such as low cost, simple equipment, ease of operation, and minimal or no use of organic solvents.
However, it has disadvantages such as long manufacturing cycles, product quality may be reduced, and pH fluctuates widely during the manufacturing process [122]. With regard to bio-coagulation, a cation and anion exchange resin was used to identify the nature of the charge carried by the active coagulation protein.
Sánchez-martín et al. studied the impact of the degree of protein purification by ion exchange chromatography on coagulation yield. Interestingly, the ion exchange process led to the production of a more purified coagulant containing more active coagulating proteins. This indicates that the purification process can produce a natural coagulant with a higher proportion of active coagulation compounds, which subsequently leads to better performance, even at a lower optimum dosage [145].
Electrophoresis refers to the migration of charged molecules and particles under the action of an electric field, as shown in Fig. 6. In this process, the substance is usually in an aqueous solution, and the charged molecules migrate to the oppositely charged electrode. Due to their different charges and masses, the different molecules and particles in the mixture migrate at different speeds and are therefore separated into different parts. Electrophoretic mobility (as a measure of migration speed) is a characteristic and crucial parameter of each charged molecule [146]. The mobility of an ionic particle is determined by the shape, charge and size of the particle, as well as by temperature during separation, and is constant under given electrophoretic conditions. Electrophoretic conditions are characterized by parameters such as current, voltage, power, ionic strength, pH, viscosity, pore size, etc., which describe the medium in which the particles move [147].
Figure 6: Electrophoresis apparatus (a) Schematic diagram (b) Gel electrophoresis
As an analytical tool, electrophoresis is a simple and relatively rapid technique. It is mainly used for the analysis and purification of large molecules such as total phenolic and proteins [146], this technique was used by Taiwo et al. to purify coagulating agents from Moringa oleifera. In this study, protein purity and molecular weight were analyzed by sodium sulfate gel electrophoresis under specific conditions [65].
Filtration is a mechanical means of separating solids from a liquid suspension by means of a porous medium or screen that allows the liquid to pass through while retaining the solids, as shown in Fig. 7 [144]. The filtration process has been known for a long time, and has been used for the purification of coagulating agents by various researchers, such as Bouchareb et al. [40,124]. After extraction of the active coagulant agent by one of the extraction methods, the mixture (liquid/solid) is filtered through a specific filter (such as the 0.45 μm filter) [148], and the filtrate obtained is used as an organic coagulant.
Figure 7: Filtration mechanism
Centrifugation is a mechanical process in which a sample is centrifuged with a specific force for a specific time to force particles in solution to settle to the bottom of a centrifuge tube (see Fig. 8). Centrifuges vary in number of tubes, size, capacity and speed [149]. To operate efficiently, the centrifuge must be balanced, i.e., the number and weight of samples around the rotor circumference must be equal. A “dummy” tube containing only water can be added to ensure that the centrifuge is balanced before operation [149].
Figure 8: Principle of the centrifuge
According to the study by Baptista et al., Moringa coagulants were separated and purified using a centrifuge at 15,000 rpm for 40 min [78].
8 Sustainability of Natural Coagulants
In the context of wastewater and drinking water treatment processes using bio-coagulants, sustainability can be considered by integrating environmental, technical, and economic aspects [18,150].
8.1 Environmental Sustainability
• The use of bio-coagulants that could reduce sludge volume and not contribute to sludge toxicity could be of interest to water utilities [151]. Organic sludge generated by natural coagulants could offer environmental sustainability if it could be reused for other purposes, such as in the civil engineering industry (concrete, cement and mortar), agricultural and land uses, and wastewater treatment plants [152].
• The purity of the coagulating agents in natural coagulants can be raised to reduce and eliminate impurities [153].
• Bio-coagulant residues are considered organic substances that could potentially interact with disinfectants (from the downstream disinfection process) to form carcinogenic disinfection by-products [154]. Therefore, precautions should be taken when using plant coagulants if a downstream disinfection process is available.
Organic coagulants have a proven track record of removing colloidal particles and total suspended solids (TSS) from polluted water [77]. Appropriate selection of organic coagulants can lead to promising coagulation performance, which is presented as an alternative to inorganic coagulants.
8.2.2 Availability of Plant Raw Materials
Benalia et al. have shown that organic coagulants are reliable and readily available sources and can be obtained from a variety of sources, namely: natural wastes, etc. [17,77].
8.2.3 Compatibility with Other Treatment Processes
Compatibility of natural coagulants with other water treatment processes, such as decantation, filtration and microfiltration.
When extracting, purifying, storing and transporting organic coagulants, many factors affect the performance and effectiveness of these materials, including reaction temperature, pH, maceration time, storage time, microbial effects; for example, storage temperature must be low to avoid degradation of the various active compounds responsible for coagulation, such as tannins, polyphenols, starch, proteins and polysaccharides [155].
8.2.5 Toxicity of Natural Coagulants to Humans and the Environment
Bio-coagulants are generally considered non-toxic due to their natural origin. However, the toxicological study of organic coagulants and their wider effect on living organisms remains unclear. This is particularly interesting for modified natural coagulants since they involve grafting chemicals onto the coagulant, namely acids and bases. A more detailed assessment of the toxicity of natural coagulants on humans and the environment is needed to confirm the safe use of this type of coagulant [18].
8.3 The Economics of Organic Coagulants
Concerning the economics of natural coagulants, it is difficult to convert the benefits of using natural coagulants such as lower sludge production without pH adjustment into cost benefits, as most claims have been made based on laboratory-scale observations. An industrial application of natural coagulants on the coagulation flocculation pilot or at the treatment plant level is required to justify the cost reduction indirectly linked to the benefits of using natural coagulants.
Another way to improve the economics of using natural coagulants is to hybridize them with other coagulants to reduce consumption and cost. Moreover, exploring the multiple functions of natural coagulants can also reduce overall cost, as several treatment processes (coagulation, adsorption, disinfection) can be performed with a single plant material in the treatment unit.
This article reviews important information on plant-based coagulants, including coagulation mechanisms using bio-coagulants, parameters influencing the coagulation flocculation process, coagulant extraction methods, the effect of bio-coagulants on water quality and finally sustainability of natural coagulants. The aim of this study was to investigate the effectiveness of plant-based organic coagulants on water quality. This work presents a study into the possibility of replacing certain mineral coagulants widely used in water treatment because they have adverse effects on the environment, in particular human health. Natural coagulants, on the other hand, are environmentally friendly, non-toxic, produce less sludge and contain no polluting metals. This work allows us to conclude that:
• The efficiency of the coagulation-flocculation process is influenced by several parameters, the most important of which are pH, coagulant dosage, coagulant type and raw water characterization. Understanding these factors is essential to optimize the process and achieve the desired results.
• Several types of coagulants have been responsible for the coagulation flocculation process, including proteins, polysaccharides, tannins, etc.
• The extraction and purification efficiency of coagulants is influenced by several parameters such as temperature, pH, stirring speed, etc.
• Bio-coagulants have been shown to improve the quality of drinking water, wastewater and industrial water, and are extremely effective in removing a wide range of water contaminants, including turbidity, COD, TSS, organic matter, heavy metals (zinc, copper, iron, etc.) and bacteria (total coliforms, fecal coliforms, etc.), with removal rates in many cases exceeding 95%.
It must be considered that the sustainability and renewability of natural coagulants in wastewater and drinking water treatment involve assessing environmental, technical and economic aspects. However, a number of challenges need to be addressed before natural coagulants can be widely used in water treatment plants. These challenges include the lack of long-term data on the environmental and health impacts of natural coagulants and the need for more efficient and cost-effective methods for their extraction and purification.
Acknowledgement: The National Polytechnic School of Constantine (Algeria), Higher Normal School of Constantine (Algeria), University of Constantine 3 (Algeria) and University of Lorraine (France) supported this work.
Funding Statement: The authors received no specific funding for this study.
Author Contributions: Benalia Abderrezzaq: Conceptualization, Investigation, Methodology, and Writing (Review and Editing). Derbal kerroum: Methodology, Investigation, Writing-Original Draft Preparation, Supervision, Writing-Review & Editing and Project Administration. Amrouci Zahra: Formal Analysis and Data Curation. Ouiem Baatache: Methodology and Writing, Review and Editing. Khalfaoui Amel: Data Curation and Supervision, Writing-Review. Antonio Pizzi: Writing-Review, Editing and Project Administration.
Availability of Data and Materials: Data availability is not applicable to this article as no new data were created or analyzed in this study.
Conflicts of Interest: The authors declare that they have no conflicts of interest to report regarding the present study.
References
1. Iwuozor KO, Adeniyi AG, Emenike EC, Ojeyemi T, Egbemhenghe AU, Okorie CJ, et al. Prospects and challenges of utilizing sugarcane bagasse as a bio-coagulant precursor for water treatment. Biotechnol Rep. 2023;39:e00805. doi:10.1016/j.btre.2023.e00805 [Google Scholar] [PubMed] [CrossRef]
2. Emenike EC, Adeniyi AG, Omuku PE, Okwu KC, Iwuozor KO. Recent advances in nano-adsorbents for the sequestration of copper from water. J Water Process Eng. 2022;47:102715. doi:10.1016/j.jwpe.2022.102715. [Google Scholar] [CrossRef]
3. Tony B, Barrett KM, Henry H, Benjes J, Burris B. Handbook of public water systems. 2nd edNew York: Wiley & Sons; 2001. [Google Scholar]
4. Ahmed MJ, Danish M, Anastopoulos I, Iwuozor KO. Recent progress on corn (Zea mays L.)-based materials as raw, chemically modified, carbonaceous, and composite adsorbents for aquatic pollutants: a review. J Anal Appl Pyrolysis. 2023;172:106004. doi:10.1016/j.jaap.2023.106004. [Google Scholar] [CrossRef]
5. Desjardins R. Le traitement des eaux ed Polytechnique P internationales. 2nd edCanada: Ecole Polytechnique de MontrØal; 1997 (In French). [Google Scholar]
6. Hassaan MA, Yglmaz M, Helal M, El-Nemr MA, Ragab S, El Nemr A. Isotherm and kinetic investigations of sawdust-based biochar modified by ammonia to remove methylene blue from water. Sci Rep. 2023;13:12724. doi:10.1038/s41598-023-39971-0 [Google Scholar] [PubMed] [CrossRef]
7. Maddela NR, Chakraborty S, Treatment W. Advances in the domain of environmental biotechnology. Springer; 2021. [Google Scholar]
8. Degrémont SA. Mémento technique de l’eau ed Degrémont (Paris) 10éme édit (In French); 2005. Available from: https://www.lavoisier.fr/livre/environnement/memento-technique-de-l-eau-2-volumes-inseparables-10e-ed/degremont/descriptif-9782743007171. [Accessed 2023]. [Google Scholar]
9. Ibrahim A, Yaser AZ, Lamaming J. Synthesising tannin-based coagulants for water and wastewater application: a review. J Environ Chem Eng. 2021;9:105007. doi:10.1016/j.jece.2020.105007. [Google Scholar] [CrossRef]
10. Verma AK, Dash RR, Bhunia P. A review on chemical coagulation/flocculation technologies for removal of colour from textile wastewaters. J Environ Manag. 2012;93:154–68. doi:10.1016/j.jenvman.2011.09.012 [Google Scholar] [PubMed] [CrossRef]
11. Benalia A, Derbal K. Comparative study between aluminum sulfate and ferric chloride in water treatment: turbidity removal. J des Doctoriales. 2015;1:4–9. [Google Scholar]
12. Exley C. La toxicitØ de l’aluminium chez l’homme. Morphologie. 2016;100:51–5 (In French) [Google Scholar] [PubMed]
13. Rondeau V, Commenges D, Jacqmin-gadda H, Dartigues J. Relation between aluminum concentrations in drinking water and Alzheimer’s disease: an 8-year follow-up study. Am J Epidemiol. 2000;152:59–66. doi:10.1093/aje/152.1.59 [Google Scholar] [PubMed] [CrossRef]
14. Wei X, Yang J, Chen J, Liu X, Zhao X. Chronic exposure to aluminum and risk of Alzheimer’s disease: a metal-analysis. Neurosci Lett. 2016;610:200–6. doi:10.1016/j.neulet.2015.11.014 [Google Scholar] [PubMed] [CrossRef]
15. Benalia A. Extraction et valorisation des produits actifs des plantes naturelles en tant que bio coagulants utiles dans l’amelioration de qualite des eaux (Doctorat Thesis). Université Constantine 3: Algeria; 2023 (In French). [Google Scholar]
16. Karoliny T, Souza F, Almeida CA. Textile science and clothing technology. New York City: Springer; 2018. [Google Scholar]
17. Benalia A, Derbal K. Etude expérimentale et modélisation du processus de la coagulation floculation: application aux eaux destinée a la consommation (Master Thesis). Université Constantine 3: Algeria; 2015 (In French). [Google Scholar]
18. Ang WL, Mohammad AW. State of the art and sustainability of natural coagulants in water and wastewater treatment. J Clean Prod. 2020;20:121267. [Google Scholar]
19. Saleem M, Bachmann RT. A contemporary review on plant-based coagulants for applications in water treatment. J Ind Eng Chem. 2018;72:281–97. [Google Scholar]
20. Bratby J. Coagulation and flocculation with an emphasis on water and wastewater treatment. England: Uplands Press, Ltd.; 1980. [Google Scholar]
21. Saimi J, Österberg M, Stenius P, Laine J. Surface forces between cellulose surfaces in cationic polyelectrolyte solutions: the effect of polymer molecular weight and charge density. Nord Pulp Paper Res J. 2007;22:249–57. doi:10.3183/npprj-2007-22-02-p249-257. [Google Scholar] [CrossRef]
22. Park SJ, Seo MK. Interface science and technology. 1st edKorea: Elsevier Science Publishing Co., Inc.; 2011. p. 1–57. [Google Scholar]
23. Schmickler W. Double layer theory. J Solid State Electrochem. 2020;24:2175–6. doi:10.1007/s10008-020-04597-z. [Google Scholar] [CrossRef]
24. Chang Q. Colloid and interface chemistry for water quality control. USA: Elsevier; 2016. [Google Scholar]
25. Marobhe NJ, Dalhammar G, Gunaratna KR. Simple and rapid methods for purification and characterization of active coagulants from the seeds of Vigna unguiculata and Parkinsonia aculeata. Environ Technol. 2007;28:671–81. doi:10.1080/09593332808618827 [Google Scholar] [PubMed] [CrossRef]
26. Antov MG, Šćiban MB, Petrović NJ. Proteins from common bean (Phaseolus vulgaris) seed as a natural coagulant for potential application in water turbidity removal. Bioresour Technol. 2010;101:2167–72. doi:10.1016/j.biortech.2009.11.020 [Google Scholar] [PubMed] [CrossRef]
27. Benalia A, Atime L, Baatache O, Khalfaoui A, Fadia A. Removal of lead in water by coagulation flocculation process using Cactus—based natural coagulant: optimization and modeling by response surface methodology (RSM). Environ Monit Assess. 2024;196:1–23. [Google Scholar]
28. Siéliéchi JM, Lartiges BS, Kayem GJ, Hupont S, Frochot C, Ghanbaja J, et al. Changes in humic acid conformation during coagulation with ferric chloride: implications for drinking water treatment. Water Res. 2008;42:2111–23. doi:10.1016/j.watres.2007.11.017 [Google Scholar] [PubMed] [CrossRef]
29. Kolya H, Kang CW. Bio-based polymeric flocculants and adsorbents for wastewater treatment. Sustainability. 2023;15(12):1–136. [Google Scholar]
30. Zheng H, Zhu G, Jiang S, Tshukudu T, Xiang X, Zhang P, et al. Investigations of coagulation–flocculation process by performance optimization, model prediction and fractal structure of flocs. Desalination. 2011;269:148–56. doi:10.1016/j.desal.2010.10.054. [Google Scholar] [CrossRef]
31. Baatache O, Derbal K, Benalia A, Khalfaoui A, Bouchareb R, Panico A, et al. Use of Pine cone as bio-coagulant for heavy metal removal from industrial wastewater: use of Box-Behnken design. Industrial Crops & Products. 2024;210:118185. doi:10.1016/j.indcrop.2024.118185. [Google Scholar] [CrossRef]
32. Dwarapureddi KB, Saritha B. Plant based coagulants for point of use water treatment—A review. Curr Environ Eng. 2016;3:61–76. doi:10.2174/221271780301160527201624. [Google Scholar] [CrossRef]
33. Mohammed Redha Z. Multi-response optimization of the coagulation process of real textile wastewater using a natural coagulant. Arab J Basic Appl Sci. 2020;27:406–22. doi:10.1080/25765299.2020.1833509. [Google Scholar] [CrossRef]
34. Saritha V, Srinivas N, Srikanth Vuppala NV. Analysis and optimization of coagulation and flocculation process. Appl Water Sci. 2017;7:451–60. doi:10.1007/s13201-014-0262-y. [Google Scholar] [CrossRef]
35. Benalia A, Baatache O, Derbal K, Khalfaoui A. The use of central composite design (CCD) to optimize and model the coagulation-flocculation process using a natural coagulant: application in jar test and semi-industrial scale. J Water Process Eng. 2024;57:104704. doi:10.1016/j.jwpe.2023.104704. [Google Scholar] [CrossRef]
36. Daverey A, Tiwari N, Dutta K. Utilization of extracts of Musa paradisica (banana) peels and Dolichos lablab (Indian bean) seeds as low-cost natural coagulants for turbidity removal from water. Environ Sci Pollut Res. 2019;26:34177–83. doi:10.1007/s11356-018-3850-9 [Google Scholar] [PubMed] [CrossRef]
37. Desjardins C, Koudjonou B, Desjardins R. Laboratory study of ballasted flocculation. Water Res. 2002;36:744–54. doi:10.1016/S0043-1354(01)00256-1 [Google Scholar] [PubMed] [CrossRef]
38. Mohd-Asharuddin S, Othman N, Mohd-Zin NS, Tajarudin HA. Removal of total suspended solid by natural coagulant derived from cassava peel waste. Journal of Physics: Conference Series. 2018;995:1–9. [Google Scholar]
39. Benalia A, Chaibraa W, Djeghar S, Derbal K, Khalfaoui A, Mahfouf A, et al. Use of extracted proteins from oak leaves as bio-coagulant for water and wastewater treatment: optimization by a fractional factorial design. Water. 2023;15(11):1–14. [Google Scholar]
40. Bouchareb R, Derbal K, Benalia A. Optimization of active coagulant agent extraction method from Moringa oleifera seeds for municipal wastewater treatment. Water Sci Technol. 2021;84(2):393–403. doi:10.2166/wst.2021.234 [Google Scholar] [PubMed] [CrossRef]
41. Putra RS, Fitria F. Effect of rapid-mixing duration on floc growth in the coagulation of peat water with Sesbania grandiflora seed as measured by DinoCapture 2.0. Netherlands: Atlantis Press International BV; 2023. [Google Scholar]
42. Xiao F, Huang JH, Zhang B, Cui C. Effects of low temperature on coagulation kinetics and floc surface morphology using alum. Desalination. 2009;237:201–13. doi:10.1016/j.desal.2007.12.033. [Google Scholar] [CrossRef]
43. Ezemagu IG, Ejimofor MI, Menkiti MC, Nwobi-okoye CC. Modeling and optimization of turbidity removal from produced water using response surface methodology and artificial neural network. S Afr J Chem Eng. 2021;35:78–88. [Google Scholar]
44. Kang LS, Cleasby JL. Temperature effects on flocculation kinetics using Fe(III) coagulant. J Environ Eng. 1995;121:893–901. doi:10.1061/(ASCE)0733-9372(1995)121:12(893). [Google Scholar] [CrossRef]
45. Guan D, Zhang Z, Li X, Liu H. Effect of pH and temperature on coagulation efficiency in a North-China water treatment plant. Adv Mater Res. 2011;243–249:4835–8. [Google Scholar]
46. Javoy S. DØtermination de constantes de vitesse de rØactions chimiques ØlØmentaires importantes dans la combustion de l’hydrogène en rØgime d’Øcoulement supersonique (In French); 2001. Available from: https://theses.hal.science/tel-02196987v1. [Accessed 2023]. [Google Scholar]
47. Nkurunziza T, Nduwayezu JB, Banadda EN, Nhapi I. The effect of turbidity levels and Moringa oleifera concentration on the effectiveness of coagulation in water treatment. Water Sci Technol. 2009;59:1551–8. doi:10.2166/wst.2009.155 [Google Scholar] [PubMed] [CrossRef]
48. Muyibi SA, Evison LM. Optimizing physical parameters affecting coagulation of turbid water with Moringa oleifera seeds. Water Res. 1995;29:2689–95. doi:10.1016/0043-1354(95)00133-6. [Google Scholar] [CrossRef]
49. Odiyo JO, Bassey OJ, Ochieng A, Chimuka L. Coagulation efficiency of Dicerocaryum eriocarpum (DE) plant. Water SA. 2017;43:1–6. doi:10.4314/wsa.v43i1.01. [Google Scholar] [CrossRef]
50. Janna H. Effectiveness of using natural materials as a coagulant for reduction of water turbidity in water treatment. World J Eng Technol. 2016;4:505–16. doi:10.4236/wjet.2016.44050. [Google Scholar] [CrossRef]
51. Asrafuzzaman M, Fakhruddin ANM, Hossain MA. Reduction of turbidity of water using locally available natural coagulants. ISRN Microbiol. 2011;2011:1–6. doi:10.5402/2011/632189 [Google Scholar] [PubMed] [CrossRef]
52. Ramphal S, Muzi Sibiya S. Optimization of time requirement for rapid mixing during coagulation using a photometric dispersion analyzer. Procedia Eng. 2014;70:1401–10. doi:10.1016/j.proeng.2014.02.155. [Google Scholar] [CrossRef]
53. Zhu K, El-din MG, Moawad AK, Bromley D. Physical and chemical processes for removing suspended solids and phosphorus from liquid swine manure. Environ Technol. 2004;25:1177–87. doi:10.1080/09593332508618385 [Google Scholar] [PubMed] [CrossRef]
54. Padhiyar H, Thanki A, Kumar N, Pandey S, Yadav M. Parametric and kinetic investigations on segregated and mixed textile effluent streams using Moringa oleifera seed powders of different sizes. J Water Process Eng. 2020;34:101159–70. doi:10.1016/j.jwpe.2020.101159. [Google Scholar] [CrossRef]
55. Bhatia S, Othman Z, Ahmad AL. Coagulation—flocculation process for POME treatment using Moringa oleifera seeds extract: optimization studies. Chem Eng J. 2007;133:205–12. doi:10.1016/j.cej.2007.01.034. [Google Scholar] [CrossRef]
56. Holmes M, Reeve P, Pestana C, Chow C, Newcombe G, West J. Zeta potential measurement for water treatment coagulation control. In: Proceedings of the Oz Water. 2015; 2015; Adelaide, Australia. [Google Scholar]
57. López-Maldonado EA, Oropeza-Guzman MT, Jurado-Baizaval JL, Ochoa-Terán A. Coagulation- flocculation mechanisms in wastewater treatment plants through zeta potential measurements. J Hazard Mater. 2014;279:1–10. doi:10.1016/j.jhazmat.2014.06.025 [Google Scholar] [PubMed] [CrossRef]
58. Jefferson B, Sharp EL, Goslan E, Henderson R, Parsons SA. Application of charge measurement to water treatment processes. Water Sci Technol: Water Supply. 2004;4:49–56. [Google Scholar]
59. Hussain S, Ghouri AS, Ahmad A. Pine cone extract as natural coagulant for purification of turbid water. Heliyon. 2019;5:e01420. doi:10.1016/j.heliyon.2019.e01420 [Google Scholar] [PubMed] [CrossRef]
60. Fooladvand M, Ramavandi B, Zandi K, Ardestani M. Investigation of trihalomethanes formation potential in Karoon River water. Iran Environ Monit Assess. 2011;178:63–71. doi:10.1007/s10661-010-1672-4 [Google Scholar] [PubMed] [CrossRef]
61. Ramavandi B. Treatment of water turbidity and bacteria by using a coagulant extracted from Plantago ovata. Water Resour Ind. 2014;6:36–50. doi:10.1016/j.wri.2014.07.001. [Google Scholar] [CrossRef]
62. Ma S, Liu C, Yang K, Lin D. Coagulation removal of humic acid-stabilized carbon nanotubes from water by PACl: influences of hydraulic condition and water chemistry. Sci Total Environ. 2012;439:123–8. doi:10.1016/j.scitotenv.2012.09.046 [Google Scholar] [PubMed] [CrossRef]
63. Jahn S, Dinar H. Studies on natural water coagulants in the Sudan, with special: reference to Moringa oleifera seeds. Water SA. 1979;5:90–106. [Google Scholar]
64. Choy SY, Murthy K, Prasad N, Wu TY, Raghunandan ME, Ramanan RN. Utilization of plant-based natural coagulants as future alternatives towards sustainable water clarification. J Environ Sci. 2014;6:2178–89. [Google Scholar]
65. Taiwo AS, Adenike K, Aderonke O. Efficacy of a natural coagulant protein from Moringa oleifera (Lam) seeds in treatment of Opa reservoir water, Ile-Ife. Nigeria Heliyon. 2020;6:e03335. doi:10.1016/j.heliyon.2020.e03335 [Google Scholar] [PubMed] [CrossRef]
66. Choudhary M, Ray MB, Neogi S. Evaluation of the potential application of cactus (Opuntia ficus-indica) as a bio-coagulant for pre-treatment of oil sands process-affected water. Sep Purif Technol. 2019;209:714–24. doi:10.1016/j.seppur.2018.09.033. [Google Scholar] [CrossRef]
67. Hameed YT, Idris A, Hussain SA, Abdullah N, Che Man H. Effect of pre-treatment with a tannin- based coagulant and flocculant on a biofilm bacterial community and the nitrification process in a municipal wastewater biofilm treatment unit. J Environ Chem Eng. 2020;8:103679. doi:10.1016/j.jece.2020.103679. [Google Scholar] [CrossRef]
68. Beltrán-Heredia J, Sánchez-Martín J, Dávila-Acedo M. Optimization of the synthesis of a new coagulant from a tannin extract. J Hazard Mater. 2011;186(2–3):1704–12. [Google Scholar]
69. Beltrán-Heredia J, Sánchez-Martín J, Gómez-Muñoz MC. New coagulant agents fro.m tannin extracts: preliminary optimisation studies. Chem Eng J. 2010;162:1019–25. doi:10.1016/j.cej.2010.07.011. [Google Scholar] [CrossRef]
70. Valiron F. Gestion des eaux; alimentation en eau—assainissement. ed ENPC P. Paris: ENPC; 1989 (In French). [Google Scholar]
71. Asadullah, Rathnasiri PG. Optimization of adsorption-coagulation process for treatment of palm oil mill effluent (Pome) using alternative coagulant. In: International Research Symposium on Engineering Advancements (RSEA 2015); 2015; Sri Lanka: Malabe; p. 68–71. [Google Scholar]
72. Seghosime A, Awudza JAM, Buamah R, Kwarteng SO. Comparative studies on proximate composition and phytochemical screening of mango, key lime, African star apple and african pear seeds as possible coagulant aids for water treatment. Am J Environ Sci. 2017;13:325–33. doi:10.3844/ajessp.2017.325.333. [Google Scholar] [CrossRef]
73. Shahimi NSW, Zaidi NS, Bahrodin MB, Amran AH. Utilization of fruit wastes (Jackfruit and mango seeds and banana trunk) as natural coagulants in treating municipal wastewater. IOP Conf Series: Mater Sci Eng. 2021;1144:012049. doi:10.1088/1757-899X/1144/1/012049. [Google Scholar] [CrossRef]
74. Freitas TKFS, Oliveira VM, de Souza MTF, Geraldino HCL, Almeida VC, Fávaro SL, et al. Optimization of coagulation-flocculation process for treatment of industrial textile wastewater using okra (A. esculentus) mucilage as natural coagulant. Ind Crops Prod. 2015;76:538–44. doi:10.1016/j.indcrop.2015.06.027. [Google Scholar] [CrossRef]
75. Jatav KA, Gawas S, Yadav S, Parmar A, Kadam P. Coagulation efficiency of okra seed extract for surface water treatment. Int J Sci Res (IJSR). 2016;5:2100–2. doi:10.21275/23197064. [Google Scholar] [CrossRef]
76. Ndive JN, Obiora-Okafo IA, Onukwuli OD. Optimization studies on the coagulation-flocculation process for PWW treatment using cactus oputia extract: comparative studies for performance evaluation. J Basic App Res Int. 2023;29:16–31. doi:10.56557/jobari/2023/v29i48409. [Google Scholar] [CrossRef]
77. Benalia A, Derbal K, Panico A, Pirozzi F. Use of acorn leaves as a natural coagulant in a drinking water treatment plant. Water. 2019;11:1–12. [Google Scholar]
78. Baptista ATA, Silva MO, Gomes RG, Bergamasco R, Vieira MF, Vieira AMS. Protein fractionation of seeds of Moringa oleifera lam and its application in superficial water treatment. Sep Purif Technol. 2017;180:114–24. doi:10.1016/j.seppur.2017.02.040. [Google Scholar] [CrossRef]
79. Al-gheethi A, Mohamed R, Wurochekke A, Nurulainee N, Mas Rahayu J, Hashim MA. Efficiency of Moringa oleifera seeds for treatment of laundry wastewater. MATEC Web Conf. 2017;103:1–8. [Google Scholar]
80. Kazi T, Virupakshi A. Treatment of tannery wastewater using natural coagulants. Int J Innov Rese Sci Eng Technol. 2010;2:23–46. [Google Scholar]
81. Dehghani M, Alizadeh MH. The effects of the natural coagulant Moringa oleifera and alum in wastewater treatment at the Bandar Abbas Oil Refinery. Environ Health Eng Manag. 2016;3:225–30. doi:10.15171/EHEM.2016.24. [Google Scholar] [CrossRef]
82. Kakoi B, Wambua J, Ndiba P, Thiong G. Banana pith as a natural coagulant for polluted river water. Ecol Eng. 2016;95:699–705. doi:10.1016/j.ecoleng.2016.07.001. [Google Scholar] [CrossRef]
83. Bouteflika A. Valeurs limites des paramètres de rejets d’effluents liquides industriels. J Officiel de la République Algérienne. 2006;26:1–27 (In French). [Google Scholar]
84. Boutteflika A. Paramètres de qualité de l’eau de consommation humaine. J Officiel de la République Algérienne. 2011;18:6–9 (In French). [Google Scholar]
85. Dwivedi AK. Researches in water pollution: a review. Inte Res J Nat App Sci. 2017;4:118–42. [Google Scholar]
86. Vidu R, Matei E, Predescu AM, Alhalaili B, Pantilimon C, Tarcea C, et al. Removal of heavy metals from wastewaters: a challenge from current treatment methods to nanotechnology applications. Toxics. 2020;8:1–37. [Google Scholar]
87. Abdulrasaq OO, Basiru OG. Removal of copper (IIiron (III) and lead (II) ions from Mono- component simulated waste effluent by adsorption on coconut husk. Afr J Environ Sci Technol. 2010;4:382–7. doi:10.5897/AJEST. [Google Scholar] [CrossRef]
88. Kanwal F, Rehman R, Anwar J, Mahmud T. Adsorption studies of cadmium (II) using novel composites of polyaniline with rice husk and saw dust of Eucalyptus camaldulensis. Elect J Environ Agric Food Chem. 2011;10:2972–85. [Google Scholar]
89. Bhattacharyya D, Jumawan AB, Grieves RB. Separation of toxic heavy metals by sulfide precipitation. Sep Sci Technol. 1979;14:441–52. doi:10.1080/01496397908058096. [Google Scholar] [CrossRef]
90. Chen Q, Luo Z, Hills C, Xue G, Tyrer M. Precipitation of heavy metals from wastewater using simulated flue gas: sequent additions of fly ash, lime and carbon dioxide. Water Res. 2009;43:2605–14. doi:10.1016/j.watres.2009.03.007 [Google Scholar] [PubMed] [CrossRef]
91. Alvarez MT, Crespo C, Mattiasson B. Precipitation of Zn(IICu(II) and Pb(II) at bench-scale using biogenic hydrogen sulfide from the utilization of volatile fatty acids. Chemosphere. 2007;66:1677–83. doi:10.1016/j.chemosphere.2006.07.065 [Google Scholar] [PubMed] [CrossRef]
92. Zewail TM, Yousef NS. Kinetic study of heavy metal ions removal by ion exchange in batch conical air spouted bed. Alex Eng J. 2015;54:83–90. doi:10.1016/j.aej.2014.11.008. [Google Scholar] [CrossRef]
93. Elektorowicz M, Muslat Z. Removal of heavy metals from oil sludge using ion exchange textiles. Environ Technol. 2008;29:393–9. doi:10.1080/09593330801984290 [Google Scholar] [PubMed] [CrossRef]
94. Skotta A, Jmiai A, Elhayaoui W, El-Asri A, Tamimi M, Assabbane A, et al. Suspended matter and heavy metals (Cu and Zn) removal from water by coagulation/flocculation process using a new bio-flocculant: lepidium sativum. J Taiwan Inst Chem Eng. 2023;145(4):104792–817. [Google Scholar]
95. Khalil F, Bouaouine O, Chtioui H, Souabi S, Aboulhassan MA, Ouammou A, et al. Traitement des lixiviats de dØcharge par coagulation-floculation. J Mater Environ Sci. 2015;6:1337–42. [Google Scholar]
96. Akbal F, Camcidotless S. Copper, chromium and nickel removal from metal plating wastewater by electrocoagulation. Desalination. 2011;269:214–22. doi:10.1016/j.desal.2010.11.001. [Google Scholar] [CrossRef]
97. Pociecha M, Lestan D. Using electrocoagulation for metal and chelant separation from washing solution after EDTA leaching of Pb, Zn and Cd contaminated soil. J Hazard Mater. 2010;174:670–8. doi:10.1016/j.jhazmat.2009.09.103 [Google Scholar] [PubMed] [CrossRef]
98. Ozaki H, Sharmab K, Saktaywirf W. Performance of an ultra-low-pressure reverse osmosis membrane (ULPROM) for separating heavy metal: effects of interference parameters. Desalination. 2002;144(1–3):287–94. [Google Scholar]
99. Kryvoruchko A, Yurlova L, Kornilovich B. Purification of water containing heavy metals by chelating-enhanced ultrafiltration. Desalination. 2002;144:243–8. doi:10.1016/S0011-9164(02)00319-3. [Google Scholar] [CrossRef]
100. Kurniawan TA, Chan GYS, Lo WH, Babel S. Physico-chemical treatment techniques for wastewater laden with heavy metals. Chem Eng J. 2006;118:83–98. doi:10.1016/j.cej.2006.01.015. [Google Scholar] [CrossRef]
101. Barakat MA. New trends in removing heavy metals from industrial wastewater. Arab J Chem. 2011;4:361–77. doi:10.1016/j.arabjc.2010.07.019. [Google Scholar] [CrossRef]
102. Fu F, Wang Q. Removal of heavy metal ions from wastewaters: a review. J Environ Manag. 2011;92:407–18. doi:10.1016/j.jenvman.2010.11.011 [Google Scholar] [PubMed] [CrossRef]
103. Bouaouine O, Baudu M, Khalil F, Chtioui H, Zaitan H. Comparative study between Moroccan cactus and chemicals coagulants for textile effluent treatment. J Mater Environ Sci. 2017;2:2687–93. [Google Scholar]
104. Ali EN, Tien Seng H. Heavy metals (Fe, Cu, and Cr) removal from wastewater by Moringa oleifera press cake. MATEC Web Conf. 2018;150:1–5. [Google Scholar]
105. Shah MP. Removal of emerging contaminants through microbial processes. 1st edIndia: Springer Singapore; 2021. [Google Scholar]
106. Derbe T, Dargo H, Batu W. Cactus potential in heavy metal (Pb and Cd) removal in water sample collected from rural area around adigrat town. Chem Mater Res. 2015;7:84–93. [Google Scholar]
107. Martínez-velarde R, Vargas-solano SV, Rodríguez-gonz F, Jonathan MP. Removal of heavy metals present in water from the Yautepec River Morelos México, using Opuntia ficus-indica mucilage. Environ Adv. 2022;7:100160. doi:10.1016/j.envadv.2021.100160. [Google Scholar] [CrossRef]
108. Nharingo T, Zivurawa MT, Guyo U. Erratum to: exploring the use of cactus Opuntia ficus indica in the biocoagulation–flocculation of Pb(II) ions from wastewaters. Int J Environ Sci Technol. 2015;12:3803. doi:10.1007/s13762-015-0835-9. [Google Scholar] [CrossRef]
109. Mataka LM, Henry EMT, Masamba WRL, Sajidu SM. Lead remediation of contaminated water using Moringa stenopetala and Moringa oleifera seed powder. Int J Environ Sci Technol. 2006;3:131–9. doi:10.1007/BF03325916. [Google Scholar] [CrossRef]
110. Nand V. Water purification using Moringa oleifera and other locally available seeds in fiji for heavy metal removal. Int J App Sci Technol. 2012;2:125–9. [Google Scholar]
111. Al-Samawi AA, Hama AR. The use of okra as a coagulant and coagulant aids in the removal of heavy metals of solid waste leachates. Eng Technol J. 2012;30:3524–35. doi:10.30684/etj.30.20.4. [Google Scholar] [CrossRef]
112. Righetto I, Al-Juboori RA, Kaljunen JU, Mikola A. Multipurpose treatment of landfill leachate using natural coagulants—Pretreatment for nutrient recovery and removal of heavy metals and micropollutants. J Environ Chem Eng. 2021;9:105213. doi:10.1016/j.jece.2021.105213. [Google Scholar] [CrossRef]
113. Suarez M, Entenza JM, Doerries C, Meyer E, Bourquin L, Sutherland J, et al. Expression of a plant-derived peptide harboring water-cleaning and antimicrobial activities. Biotechnol Bioeng. 2002;81:13–20. [Google Scholar]
114. Broin M, Santaella C, Cuine S, Kokou K, Peltier G, Joet T. Flocculent activity of a recombinant protein from Moringa oleifera Lam. seeds. Appl Microbiol Biotechnol. 2002;60:114–9. doi:10.1007/s00253-002-1106-5 [Google Scholar] [PubMed] [CrossRef]
115. Madsen M, Jørgen S. Effect of water coagulation by seeds of Moringa oleifera on bacterial concentrations. J Trop Med Hyg. 1987;90:101–9 [Google Scholar] [PubMed]
116. Ghebremichael K, Abaliwano J, Amy G. Combined natural organic and synthetic inorganic coagulants for surface water treatment. J Water Supply. 2009;58:267–76. doi:10.2166/aqua.2009.060. [Google Scholar] [CrossRef]
117. Poumaye N, Mabingui J, Lutgen P, Bigan M. Contribution to the clarification of surface water from the Moringa oleifera: case M’Poko River to Bangui. Chem Eng Res Des. 2012;90:2346–52. doi:10.1016/j.cherd.2012.05.017. [Google Scholar] [CrossRef]
118. Amran AH, Zaidi NS, Muda K, Loan LW. Effectiveness of natural coagulant in coagulation process: a review. Int J Eng Technol. 2018;7:34–7. [Google Scholar]
119. Nejia H. Extraction et purification de substances naturelles: Comparaison de l’extraction au CO2- supercritique et des techniques conventionnelles (In French); 2013. Available from: https://theses.hal.science/tel-04237879v1/document. [Accessed 2023]. [Google Scholar]
120. Chanioti S, Tzia C. Food engineering handbook. Boca Raton: CRC Press; 2014. p. 254–83. [Google Scholar]
121. Djema R, Rahma F, Benalia A, Derbal K, Khalfaoui A. Extraction And purification of the active substance contained in the aloe vera for their use as a natural flocculant. All Sci Proc. 2023;1(1):14. [Google Scholar]
122. Outline C. Technologies for biochemical conversion of biomass. 8th edAmsterdam: Elsevier Inc; 2017. p. 197–217. [Google Scholar]
123. Abidin ZZ, Mohd NS, Madehi N, Sobri S. Optimisation of a method to extract the active coagulant agent from Jatropha curcas seeds for use in turbidity removal. Ind Crops Prod. 2013;41:319–23. doi:10.1016/j.indcrop.2012.05.003. [Google Scholar] [CrossRef]
124. Selmane D. Etude de l’extraction des protØines de coproduits d’abattage et de leur valorisation comme ingrédients fonctionnels (In French); 2010. Available from: https://theses.hal.science/tel-00719205. [Accessed 2023]. [Google Scholar]
125. Preece KE, Hooshyar N, Zuidam NJ. Whole soybean protein extraction processes: a review. Innov Food Sci Emerg Technol. 2017;43:163–72. doi:10.1016/j.ifset.2017.07.024. [Google Scholar] [CrossRef]
126. Jovanovic A, Petrovic P, Đordjevic V, Zdunic G, Savikin K, Bugarski B. Polyphenols extraction from plant sources. Lekovite Sirovine. 2017;37:45–9. [Google Scholar]
127. Megersa M, Gach W, Beyene A, Ambelu A, Triest L. Effect of salt solutions on coagulation performance of Moringa stenopetala and Maerua subcordata for turbid water treatment. Sep Purif Technol. 2019;221:319–24. doi:10.1016/j.seppur.2019.04.013. [Google Scholar] [CrossRef]
128. Baatache O, Derbal K, Benalia A, Aberkane I, Guizah QE, Khalfaoui A, et al. Valorization of pine cones (Pinus nigras) for industrial wastewater treatment and crystal violet removal: a sustainable approach based on bio-coagulants and a bio-adsorbent. Water. 2024;16:260. doi:10.3390/w16020260. [Google Scholar] [CrossRef]
129. Vishali S, Roshini SK, Samyuktha MR, Ashish K. Towards zero waste production in the paint industry wastewater using an agro-based material in the treatment train. Environ Monit Assess. 2018;190:587–95. doi:10.1007/s10661-018-6904-z [Google Scholar] [PubMed] [CrossRef]
130. Dotto J, Fagundes-klen MR, Veit MT, Palácio SM, Bergamasco R. Performance of different coagulants in the coagulation/flocculation process of textile wastewater. J Clean Prod. 2018;20:656–65. [Google Scholar]
131. Vishali S, Karthikeyan R. Cactus opuntia (ficus-indicaan eco-friendly alternative coagulant in the treatment of paint effluent. Desalination Water Treat. 2015;56:1489–97. doi:10.1080/19443994.2014.945487. [Google Scholar] [CrossRef]
132. Baldwin RL. How hofmeister interactions affect protein stability. Biophys J. 1996;71:2056–63. doi:10.1016/S0006-3495(96)79404-3 [Google Scholar] [PubMed] [CrossRef]
133. Benalia A, Derbal K, Khalfaoui A, Bouchareb R, Panico A, Gisonni C, et al. Use of aloe vera as an organic coagulant for improving drinking water quality. Water. 2021;13:2024–39. doi:10.3390/w13152024. [Google Scholar] [CrossRef]
134. Benalia A, Derbal K, Khalfaoui A, Pizzi A, Medjahdi G. The use of as natural coagulant in algerian drinking water treatment plant. J Renew Mater. 2022;10(3):625–37. doi:10.32604/jrm.2022.017848. [Google Scholar] [CrossRef]
135. Behera B, Balasubramanian P. Natural plant extracts as an economical and ecofriendly alternative for harvesting microalgae. Bioresour Technol. 2019;283:45–52. doi:10.1016/j.biortech.2019.03.070 [Google Scholar] [PubMed] [CrossRef]
136. Hadadi A, Imessaoudene A, Bollinger JC, Assadi AA, Amrane A, Mouni L. Comparison of four plant- based bio-coagulants performances against alum and ferric chloride in the turbidity improvement of bentonite synthetic water. Water. 2022;14(20):1–16. [Google Scholar]
137. Carvalho MS, Alves BRR, Silva MF, Bergamasco R, Coral LA, Bassetti FJ, et al. CaCl2 applied to the extraction of Moringa oleifera seeds and the use for Microcystis aeruginosa removal. Chem Eng J. 2016;304:469–75. doi:10.1016/j.cej.2016.06.101. [Google Scholar] [CrossRef]
138. Ahmad A, Rozaimah S, Abdullah S, Hasan HA, Othman AR, Ismail NI. Potential of local plant leaves as natural coagulant for turbidity removal. Environ Sci Pollut Res. 2021;29:2579–87. [Google Scholar]
139. Amran AH, Zaidi NS, Syafiuddin A, Zhan LZ, Bahrodin MB, Mehmood MA, et al. Potential of Carica papaya seed-derived bio-coagulant to remove turbidity from polluted water assessed through experimental and modeling-based study. Appl Sci. 2021;11:5715. doi:10.3390/app11125715. [Google Scholar] [CrossRef]
140. Grossmann L, Ebert S, Hinrichs J, Weiss J. Production of protein-rich extracts from disrupted microalgae cells: impact of solvent treatment and lyophilization. Algal Res. 2018;36:67–76. doi:10.1016/j.algal.2018.09.011. [Google Scholar] [CrossRef]
141. Benalia A, Hamdane H, Tabchich A, Amirou S, Pizzi A. Use of tannin mimosa based natural coagulants for wastewater treatment: optimization and modeling using RSM methodology. All Sci Abstr. 2023;1(1):17. [Google Scholar]
142. Al-Sahari M, Al-Gheethi AAS, Radin Mohamed RMS. Prospects of fresh market wastes management in developing countries. USA: Springer; 2020. pp. 17–31. [Google Scholar]
143. Andrew SM, Titus JA, Zumstein L. Dialysis and concentration of protein solutions. Curr Protoc. 2001;3:1–5. [Google Scholar]
144. Fetterolf DM. Lyophilization. J Validation. 2010;14(4):18–23. [Google Scholar]
145. Sánchez-martín J, Ghebremichael K, Beltrán-heredia J. Comparison of single-step and two-step purified coagulants from Moringa oleifera seed for turbidity and DOC removal. Bioresour Technol. 2010;101:6259–61. doi:10.1016/j.biortech.2010.02.072 [Google Scholar] [PubMed] [CrossRef]
146. Mayer HK, Fiechter G. Comprehensive analytical chemistry. 1st edNetherlands: Elsevier; 2013. p. 251–78. [Google Scholar]
147. Fritsch RJ, Krause I. Encyclopaedia of food science. 2nd edAmsterdam: Academic Press; 2003. p. 2055–62. [Google Scholar]
148. Bouaouine O, Bourven I, Khalil F, Baudu M. Identification of functional groups of Opuntia ficus-indica involved in coagulation process after its active part extraction. Environ Sci Pollut Res. 2018;25:11111–9. doi:10.1007/s11356-018-1394-7 [Google Scholar] [PubMed] [CrossRef]
149. Walker GS. Sample preparation for chromatographic applications in forensic science. Australia: Elsevier; 2012. [Google Scholar]
150. Kamali M, Suhas DP, Elisabete M, Capela I. Sustainability considerations in membrane-based technologies for industrial e ffluents. Chem Eng J. 2019;368:474–94. doi:10.1016/j.cej.2019.02.075. [Google Scholar] [CrossRef]
151. Dorca J, Veit MT, Juchen PT, Gonçalves C, Palácio SM, Fagundes-Klen M. Use of different coagulants for cassava processing wastewater treatment. J Environ Chem Eng. 2018;6:1821–7. doi:10.1016/j.jece.2018.02.039. [Google Scholar] [CrossRef]
152. Ahmad T, Ahmad K, Alam M. Sustainable management of water treatment sludge through 3‘R’ concept. J Clean Prod. 2016;124:1–13. doi:10.1016/j.jclepro.2016.02.073. [Google Scholar] [CrossRef]
153. Barbosa AD, da Silva LF, de Paula HM, Romualdo LL, Sadoyama G, Andrade LS. Combined use of coagulation (M. oleifera) and electrochemical techniques in the treatment of industrial paint wastewater for reuse and/or disposal. Water Res. 2018;145:153–61. doi:10.1016/j.watres.2018.08.022 [Google Scholar] [PubMed] [CrossRef]
154. Oladoja NA. Headway on natural polymeric coagulants in water and wastewater treatment operations. J Water Process Eng. 2015;6:174–92. doi:10.1016/j.jwpe.2015.04.004. [Google Scholar] [CrossRef]
155. Abidin ZZ, Madehi N, Yunus R, Derahman A. Effect of storage conditions on jatropha curcas performance as biocoagulant for treating palm oil mill effluent. J Environ Sci Technol. 2019;12:92–101. doi:10.3923/jest.2019.92.101. [Google Scholar] [CrossRef]
Cite This Article
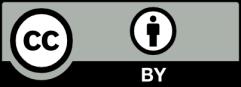