Open Access
ARTICLE
Matrix Assisted Laser Desorption Ionization Time of Flight (MALDI-TOF)-Mass Spectrometry and 13C-NMR-Identified New Compounds in Paraberlinia bifoliolata (Ekop-Beli) Bark Tannins
1 Laboratory of Forest Resources and Wood Valorization, Training Unit in Engineering Sciences, Post Graduate School of Fundamental and Applied Sciences, University of Douala, P.O. Box 1872, Douala, Cameroun
2 Laboratory of Mechanics, Training Unit in Engineering Sciences, Post Graduate School of Fundamental and Applied Sciences, University of Douala, P.O. Box 2701, Douala, Cameroun
3 Laboratory of Studies and Research on Wood Material (LERMAB), University of Lorraine, Nancy, France
* Corresponding Author: Antonio Pizzi. Email:
(This article belongs to the Special Issue: Special Issue in Celebration of JRM 10 Years)
Journal of Renewable Materials 2024, 12(3), 553-568. https://doi.org/10.32604/jrm.2023.046568
Received 07 October 2023; Accepted 21 December 2023; Issue published 11 April 2024
Abstract
Extracts of plant origin, particularly tannins, are attracting growing interest for the sustainable development of materials in the industrial sector. The discovery of new tannins is therefore necessary. The aim of this work was to contribute to the understanding of the properties of Paraberlinia bifoliolata tannin by Matrix Assisted Laser Desorption Ionization Time of Flight Mass Spectroscopy MALDI-TOF/MS and Carbon 13 Nuclear Magnetic Resonance (13C NMR). The chemical composition of tannin extracted from Paraberlinia bifoliolata bark was determined, as was the mechanical strength of the resin hardened with Acacia nilotica extracts. Yield by successive water extraction was 35%. MALDI-TOF/MS analysis revealed the presence of three new compounds in this tannin, previously unknown in this family of extracts. These are 3-hydroxyproline acid, N-methyl-4-hydroxypipecolic acid and N-methyl-5-dihydroxypipecolic acid. The identification of the above molecules means that this tannin can be used for industrial applications, as a resin in the manufacture of particleboard and in the formulation of green corrosion inhibitors. This information is reinforced by 13C NMR spectrometry, which indicates the presence of several polyflavonoid units, confirming the condensed nature of the tannin. Thermomechanical analysis of the resin formed by the purified tannin of Paraberlinia bifoliolata to which a vegetal biohardener has been added provided a Modulus of Elasticity (MOE) value of 4840 MPa at 150°C, confirming its possible use as a binder resin in the manufacture of wood panels as well as for the formulation of a corrosion inhibitor.Keywords
Although tannin has long been used in the leather tanning process, it has only recently been industrially used for other applications [1]. The reason for this interest is to substitute urea-formaldehyde (UF) resins which are now commonly used in the manufacture of wood composite materials [2]. Over 80% of composites manufactured worldwide with wood are bonded with UF adhesives [3]. Particleboard alone accounts for more than 50% of the world’s production volume. Moreover, in excess of 100 million cubic metres of UF-bonded panels are produced worldwide every year [4]. One of the main drawbacks of using these resins is their emission of formaldehyde, classified as a carcinogen by the International Agency for Research on Cancer (IARC) [5]. However, much work has been carried out with the aim of identifying plant species rich in extractables and which could provide guidance in the characterization of new tannins to help for commercial purposes..
The Fabaceae is a botanical group much sought-after for its chemical and pharmacological properties [6]. Species in the Fabaceae family are particularly rich in flavonoids and related compounds. Alkaloids, terpenoids, steroids and tannins are examples of substances found in many species of this family [7,8]. Among these secondary metabolites, the condensed tannins present in plants have been the subject of extensive studies on their activities [9]. Wender, for example, presented a new condensed tannin identified in Stryphnodendron pulcherrimum, an Amazonian plant belonging to the Fabaceae family [10]. The characterized condensed tannin contains catechin units (flavan-3-ols) and could be used to regulate digestion in the gut [9,10]. In general, proanthocyanidins are widely studied for their beneficial effects on health [11,12]. Konai et al. have shown that the species Daniellia oliveri (Cesalpinaceae) can contain up to 29% condensed tannin in its bark, which is used as a wood glue in panel manufacture [13]. Conversely, Ndiwe et al. have shown that the tannin of Gilbertiodendron dewevrei (Limbali) is of the condensed type and consists mainly of catechin gallate units. It has also been tested as a wood adhesive.
Tannins moreover are now widely used in other fields, such as pharmaceutical and medical applications, the food industry, additives and antioxidants, precipitation of pollutants by complexation of heavy metals in liquids, metal corrosion inhibitors, etc. [1]. However, as the quantity of commercial tannin available was of just 200,000 tonnes per year in 2006 [14,15], this quantity is insufficient when considering the different areas of application. It is, therefore, necessary to determine the characteristics of new environmentally friendly tannins and to identify their various areas of application.
Cameroon possesses the second-largest forest in Central Africa after the Democratic Republic of Congo. It covers almost 22 million hectares, or 46.25% of the national surface area [14]. Cameroon’s forest heritage is diverse, with more than 600 species, 300 of which are commercially exploitable, and around sixty species exploited for timber [16]. The quantity of logs produced annually is estimated at 2.7 million m3, of which 78% [17] are sold abroad in the logs form, with the remainder processed locally and exported in the form of sawn timber. For economic reasons, legislation in the CEMAC zone prohibits the export of logs, this being to benefit the local industry. Nevertheless, it should be noted that the timber industry produces large quantities of waste, which is either recovered for energy purposes or deposited in the environment [14]. In both cases, they pose a real danger to the environment, since they emit greenhouse gases that contribute to the destruction of the ozone layer [17]. They could, however, be used to produce materials that are environmentally friendly and sustainable. Among the many species of wood exploited in Cameroon, one has been chosen for its tannin content, as it has been shown that the biomass generated by the wood industry can be used as a raw material for extracting tannins [14,18,19].
Paraberlinia bifoliolata is a tropical species in the Fabaceae-Caesalpiniaceae (angiosperms) family. It is a medium to large tree, growing up to 45 m tall. It has a cylindrical trunk 20 m long, without branches, straight or slightly curved, up to 200 cm in diameter, with thin buttresses up to 2 m high; the bark is smooth, grey to orange-brown or reddish, exfoliating into small scales, the inner bark is fibrous, reddish and difficult to detach. It is a medium to heavy wood, with a density of 670 to 860 kg/m³ and a moisture content of 12%. It is restricted to tropical Africa and is found in Cameroon, Equatorial Guinea, Gabon, Congo and the west of the Democratic Republic of Congo. Marketed under the names Ekop-Beli (Cameroon), Awoura or Beli (Gabon), and Zebrali (France and Germany). It is particularly suited for cabinetmaking, joinery and sliced veneers. It can also be used in light construction, moderate parquetry, interior joinery, staircases, shipbuilding, carpentry, ladders, sports equipment, toys, trinkets, agricultural utensils, handles and sculpture [18].
The volume of Ekop-béli wood harvested in Cameroon in 2015 was 74,377 m3 of logs, which is high enough to generate significant waste when we know that for a log of wood, only 30% is finally valorised [14]. Its bark, little used even in traditional medicine, could therefore be useful for extracting tannins, in view of previous work carried out on this family of compounds [14]. The aim of this study is to assess the lack of specific use of the chemical properties of tannin extracted from the bark of tropical plants (Paraberlinia bifoliolata) in industry.
Barks were collected in November 2022, in an industrial wood processing company (TRANSBOIS) in the city of Douala, Cameroon (4°2′60″N 9°41′60″E). A 35 kg of wet bark was collected, then bagged for easy transport to the Laboratory of Forest Resources and Wood Valorization at the University of Douala. Barks were then dried for 7 days in the air sun and ground using a rotary knife mill until a fine powder with a particle diameter of 1mm was obtained. Extraction involved introducing 400 g of bark powder into an aqueous solution containing 2% sodium bisulphite and 0.5% sodium bicarbonate (the water/bark ratio being 6:1). The mixture was stirred continuously at 60°C for 4 h. The bark solution was sieved and filtered through a No. 2 Wattmann paper and the filtrate obtained was recovered and then concentrated at 60°C using a rotary evaporator, then frozen using liquid nitrogen and a laboratory-scale spray dryer (Buchi Mini Spray Dryer B 290). The tannin powder, which is easier to use, has been obtained and packaged [20,10]. The extraction percentage is calculated using the Eq. (1):
With
mbarks: the mass of bark powder taken for extraction.
Matrix-assisted laser Desorption/ionisation Time of Flight analysis has been used to determine the structure and characteristics of polyflavonoid tannins that have proved difficult using other methods. The molecules to be analysed are incorporated into a light-absorbing matrix, where they are ionised and then desorbed. The principle involved dissolving 5 mg of sample in 1 ml of acetone. The sample solution was mixed with another solution consisting of 2,5-dihydroxybenzoic acid as a matrix and acetone (10 mg/mL acetone). Ion formation was enhanced by adding sodium chloride (NaCl) to the matrix (10 mg/mL in distilled water). The resulting solutions were evaporated on the MALDI target before being placed in the spectrometer. Spectra were recorded on a KRATOS compact AXIMA PERFORMANCE MALDI TOF 2 instrument. The irradiation source was a pulsed nitrogen laser (wavelength: 337 nm, laser pulse length 3 ns and target type: ground steel) [20–23].
This method makes it possible to identify the different carbon families likely to be contained in the molecule and to assemble them to find the structure of the molecule. The tannin powder from the extracts obtained was characterised by carbon-13 nuclear magnetic resonance (13C-NMR). The spectra were recorded on a Brüker AVANCE 400 MHz spectrometer (Brüker, Billerica, MA, USA) with a 4 mm probe at a frequency of 12 kHz. Chemical shifts were calculated relative to tetramethylsilane (TMS). The rotor was rotated at 12 KHz on a 4-min Bruker double-bearing probe. Spectra were acquired with recycling delays of 5 s, a 90° pulse of 4.2 µs and a contact time of 1 ms. The number of transients was 3000. The spectra were run with rotating sidebands suppressed [24–27].
The resin was obtained using the following formulation: 40% purified tannin was dissolved in a volume of water representing 50% of the mixture and 10% Accacia nilotica exudate hardener [27–29]. The pH was adjusted to 7 with a 33% NaOH solution [29].
2.5 Thermomechanical Analysis of Resins
This analysis was used to characterize the resins and provide information on the interactions between the polymer and other molecules, and also to determine the rigidity of the resin as a function of temperature [26].
The samples were prepared by applying 25 mg of pre-prepared resin between two smooth plates measuring 21 mm × 6 mm × 1.1 mm. They were then glued and introduced into a Mettler Toledo 40 TMA thermomechanical analyser (Mettler Toledo, Zurich, Switzerland). The beech-resin-beech sandwiches were tested in non-isothermal mode between 25°C and 250°C at a heating rate of 10°C/min. The surface area occupied by the adhesive on the sheets is 200 g/m2. The sheets are decorative plies of beech wood with an average density of 0.750 g/cm3, a thickness of 0.5 mm and a moisture content of 11% [8,11]. The specimens were tested in three-point bending over a span of 18 mm using a force cycle of 0.1/0.5 N with a force cycle of 12s (6s/6s). The mechanical relationship between force and deflection is given in Eq. (2):
where E is the Young’s modulus; L is the length of the span; b and h are the width and thickness of the specimen respectively; F is the force exerted on the joint;
Bark tannin yield from the Paraberlinia bifoliolata was estimated at 35% by weight. This result is in accordance with the results obtained by Konai et al., on the extraction yield of two tropical species, Azadirachta indica and Daniellia oliveri were 35% and 29% respectively. However, tropical wood species had generally higher extraction rates such as Ficus sycomorus (46%) [30], and Butyrospermum parkii (40%) [14]. Bikoro et al., showed that the extraction rate of tannin contained in Khaya. ivorensis bark was between 36.9% and 39.1% and this yield could reach 69% if heartwood and an acetone/water solvent mixture were used for extraction [31]. Feria-Reyes et al. in 2023 extracted tannins from barks of five Mexican tree species, namely Pinus patula, Pinus ayacahuite, Pinus rudis, Pinus douglasiana and Pinus pseudostrobus. The extraction rate obtained with acetone and water are: 0.65% to 5.14% and 0.14% to 1.46%, respectively [32]. Thus, the bark of P. Bifoliolata is then of economical interest in the production of tannins like many tropical woods. Moreover, the presence of different newly compounds in the extract from these barks can lead to further pharmaceuticalor other uses either for thetannin extract or of molecules isolated from it.
Table 1 shows characteristics of oligomers present in P. Bifoliolata tannin obtained after the interpretation of the relevant MALDI-TOF peaks of the extract. Three new compounds were identified: 3-hydroxyproline, N-methyl-4-hydroxypipecolic acid and N-methyl-5-dihydroxypipecolic acid. These are still unknown in tropical wood species barks. Several characteristic peaks were identified, the most relevant being the low intensity 154 Da peak which reveals the presence of the 3-hydroxyproline molecule to which Na+ is bound, in principle 154 Da = m/z 3-hydroxyproline (130 Da) + m/z Na+(24). The peak at 159 Da indicates the presence of N-methyl 4-hydroxypipecolic acid without Na+. The peak at 176 Da of medium intensity indicates the presence of N-methyl 5-dihydroxypipecolic acid without Na+, 177 Da is the m/z value of the protonated molecule. The peak at 198 Da indicates the presence of N-methyl 5-dihydroxypipecolic acid to which Na+ is bound; 199 Da corresponds to the protonated molecule. The peaks at 263, 267, 281, 323, 371, 405, 449, 537 and 669 Da represent multiple units (dimers, trimers) of the same monomer or of several different monomers identified above. Nevertheless, the identification of certain peaks other than those that make this tannin special were identified, showing the presence of other compounds in this tannin. The 176, 177, 198 and 199 Da peaks indicate the presence of glucose in the structure. The 301 Da peak identifies the prodelphinidin monomer. The 405 Da peak characterises chalcone (-3H+) + Glucose + Na; 449 Da is a Trihydroxyflavan-3-p- hydroxybenzoate unit (-H+; + Na+); 537 Da Gallocatechol + Na+; 625 Da Quercetin dimer diprotonated + Na; 780 Da Catechin dimer tetradeprotonated + Chalcone; 811 Da Chalcone (-2H+) + Quercetin + Epigallocatechin + Na; 889 Da Gallocatechin Gallate (-2H+) + Chalcone dimer + Na+; 969 Da Chalcone trimer (+2H+) + Epigal-locatechin + 2 Na; 1085 Da Gallocatechin gallate (3H+) + Epigallocatechin + Quercetin + Na; 1261 Da catechin dimer + Chalcone + Epicatechingallate + Na (-H+; + OH-).
Saha et al. in 2012 reported that tropical wood species such as Pterocarpus soyauxii Taubb,Erythrophleum suaveolens, Baillonella toxisperma and Distemonanthus benthamianus contained homopterocarpine and pterocarpine, respectively; catechin, gallic acid and pyrogallol; diterpenes; gallic acid, squalene and triterpenes [33]. At the same time, Konai in 2015 showed that the tannins in Aningeria sppbarks are condensed and made up of catechin, gallogatechin and galloyl units [21]. In 2017, he determined the condensed nature of Ficus sycomorus bark tannin, which consists of catechin, gallocatechin, catechin gallate, fisetinidin, radicinin, chalcone, quercetin and apigenin [22,30]. The tannin of Gilbertiodendron dewevrei was studied by Ndiwe et al. in 2020, who found that catechin gallate was one of the main constituents of Gilbertiodendron dewevrei [34].
Similarly, the tannin in Piptadeniastrum Africanum bark was explored in 2021 by Wedaïna et al., who reported that it was composed of catechin, quercetin, chalcone, gallocatechin, epigallocatechin gallate and epicatechin gallate [35]. Navarette in 2010 found in Pinus maritimus bark tannin from the Landes (France): catechin, epicatechin, epigallocatechin and epicatechin gallate with molecular masses (MW) of 290.3, 290.3, 306.3 and 442.4 Da, respectively [20]. In 2013, Navarette determined the chemical properties of two maritime pine tannins intended for use in particleboard adhesives and found that these two tannins were essentially composed of catechin, epicatechin, epigallocatechin and epicatechin gallate [27]. Similarly, MALDI- TOF /MS analysis of the tannins from Schinopsis spp (Quebracho) and Acacia mearnsii (Mimosa) bark showed the predominance of profisetinidine and prorobinetinidine in these tannins [23].
In the spectra of the tannin extract of P. bifoliolata (Ekop beli) there are several features of interest. First of all, as also found in previous literature for the other Fabaceae species, but for the first time for the species under examination, 3-hydroxyproline N-methyl 4-hydroxypipecolic acid and N-methyl 5-dihydroxypipecolic acid are clearly present (Fig. 1). Also new is that in particular both the N-methyl-dihydroxypipecolic acids do oligomerize, possibly by an enzymatically catalyzed route, or alternatively perhaps during the tannin extraction if this is done with heat. Some dimer of hydroxproline are also present, although much less than those of the N-methyl-dihydroxypipecolic acids.
Figure 1: Structure of new monomers present in P. Bifoliolata tannin: (a) 3-hydroxyproline (b) N-methyl 4-hydroxypipecolic acid (c) N-methyl 5-dihydroxypipecolic acid
Further novelties are that at the higher molecular weights, three types of species are found, two of which have never been observed before. The first is the presence of oligomers of flavonoids constituting part or fractions of the tannin extract. The others are more interesting: one is the presence of flavonoid oligomers esterified by pipecolic acid, without hydroxyl groups. The suspicion here is that this might be a fabrication of the extraction process. If this has been done at a relatively high temperature this has facilitated the esterification of some of the tannin flavonoid units by the N-methyl pipecolic acid, probably with elimination of methanol (CH3OH), in the reaction. One more point to notice in these species is that not only the alcoholic -OH on the flavonoids C3 is esterified but also some phenolic -OHs, however without being able to determine if the flavonoids A or B rings are the preferred phenolic sites for such a reaction. A clear example of this are the species at 498 and 1318 Da (Table 1 and Fig. 2).
Figure 2: MALDI¬TOF/MS spectra of Ekop beli tannin in the range 50–500 Da and 50 to 1000 Da
The 13C-NMR spectrum identified exactly 13 peaks in this tannin sample (Fig. 3): 177 ppm; 165 ppm; 154 ppm; 145 ppm; 131 ppm; 117 ppm; 107 ppm; 72 ppm; 55 ppm; 43 ppm; 36 ppm; 25 ppm and 15 ppm. The 177 ppm, shift despite its low intensity, indicates the presence of the carboxylic acid function -COOH, which may be either that of pipecolic acid (piperidine-2-carboxylic acid) or hydroxyproline acid. Subsequently, the peak at 165 ppm confirms the existence of this acid function as it indicates the presence of a carbon bonded to –a CO-. The C4, C5 and C9 carbons shifts of the procyanidin appear at 154 ppm [36]. The peak at 145 ppm is attributed to the C3′ and C4′ resonances of the procyanidine B ring. The peaks at 131 and 117 ppm are attributable to the C1′ and C5′ resonances of the procyanidin units, respectively [37] and the catechol B rings present in this tannin. The peak at 107 ppm corresponds to C4–C8 interflavonoid linkage characteristic of procyanadins [34]. The peak at 72 ppm is broad and intense and corresponds to the C2 representing the carbohydrate oligomer fragments present in any condensed tannin extract [25,38]. The C2 resonance around 72 ppm indicates the preferential presence of the cis isomer in the structure [36]. The 55 ppm is the shift of lignin methoxy groups, indicating that there is some (small amount) of lignin or lignans extracted with the tannin. The 42 ppm shift is assigned to the -CH3 group linked to the N atom of the pipecolic acids. The 36 ppm is due to the rearrangement of the catechinic acid [30]. The 25 ppm belongs to the unbound flavonoid C4 [20,34]. Maritime pine tannin is mostly composed of procyanidin oligomers, but Navarrete et al. showed that the structure of this tannin was modified by the presence of gallic acid (176 ppm) [25].
Figure 3: 13C NMR analysis spectrum of Ekop beli
These analyses show that the structure of Paraberlinia bifoliolata tannin presents direct substitution of a flavonoid -OH directly by the N of the rings of pipecolic acid such as:
Such a direct substitution of a flavonoid C3 -OHs by nitrogen compounds is well documented in the literature, as in the case of amonia and amines [39–42].
Fig. 4 shows the variation in modulus of elasticity (MOE) as a function of temperature. The resin produced has three phases. The first phase, between 20°C and 55°C, corresponds to the water evaporation phase. During this phase, the tannin-hardener mixture is not very homogeneous, which could explain the resin’s low viscosity. In the second phase, we note that the MOE value increases expressively between 55°C and 150°C to reach a maximum of 4840 MPa at 150°C, which could mean that the resin formulated at this temperature is fully polymerised. This would correspond to the complete resin cure and reticulation. The third phase occurs above 150°C, where the MOE drops very rapidly. This drop is due to both the likely start of the degradation of the wood support in the TMA but also by starting to degrade already at 150°C means that the resin itself could start degrading at temperatures above 150°C. The third phase occurs above 150°C, where the MOE drops very rapidly. The resin could degrade at temperatures above 150°C with degradation of the wood substrate contributing to this degradation from about 200°C. The resin formulated with Paraberlinia bifoliolata tannin and Acacia nilotica bio-hardener performs better than that formulated with Cissus dinklagei tannin hardened with formaldehyde (MOE = 3825 MPa) [43]; as well as those formulated with Butyrospermum tannin (4620 MPa); Azadirachta indica tannin (26500 MPa) and Daniellia oliveri tannin (2370 MPa). And Vachelia Nilotica bio-hardener [34]. The resin formulated with this tannin showed a more interesting rigidity than that of maritime pine hardened with paraformaldehyde (3727 MPa), maritime pine tannin hardened with Acacia siebteriana (4343 MPa), Vachellia seyal (4337 MPa), and Senegalia Senegal (4605 MPa) extracts [44].
Figure 4: Variation in MOE of Paraberlinia Bifoliolata tannin resin hardened with Accacia Nilotica extracts as a function of temperature
The present study contributed to identifying the nature of new tannins of tropical origin. Tannin from barks of Paraberlinia Bifoliolata was extracted using water as the solvent, with an extraction yield of 35%. This ranks this species among the tropical ones with a high extractable potential. Chemical characterisation methods using MALDI-Tof and 13C-NMR showed that this tannin contains new molecules that have not yet been identified in this family of compounds and which could broaden the range of applications for this tannin. The new molecules found are 3 hydroxyproline acid; N-methyl 4-hydroxypipecolic acid; N-methyl 5-dihydroxypipecolic acid. These molecules even linked to the tannin flavonoid units, show however that this tannin is anyhow of the condensed type. Analysis of the resin showed to have a high MOE value peak of 4840 MPa at maximum curing at 150°C. This tannin can therefore be envisaged for the preparation of binders for wood particleboard. In addition, the presence of several -OH bonds, heteroatoms (O, N) and pi electrons in the structure of this tannin makes it a good potential candidate for the formulation of corrosion inhibitors.
Acknowledgement: The authors would like to express their gratitude to Professor BETENE EBANDA Fabien, Head of the Department of Mechanical Engineering, for allowing access to his laboratory for tannin extractions; to Mr. ETEME NKOA François Louis and Mr. BAYANGBE DIKMO Honoré for collecting bark in the wood yards; to Mr. MBERE TAOGA Michel and Mr. MANSASSOU Claude for their participation in the tannin extraction process; and to Dr. BESSIKE Josias Georges for his encouragement.
Funding Statement: This work was supported by the Institut de la Francophonie pour le Développement Durable (IFDD/Canada)/Projet de Déploiement des Technologies et Innovations Environnementales (PDTIE) funded by Organisation Internationale de la Francophonie (OIF), the Organisation of African, Caribbean and Pacific States and the European Union (EU) (FED/220/421-370), the Local Materials Promotion Authority (MIPROMALO) of the Ministry of Scientific Research and Innovation of Cameroon who made it possible for this scientific work to be carried out.
Author Contributions: The authors confirm their contribution to the paper as follows: study conception, data collection, design, and draft manuscript preparation: Achille Bernard Biwolé, Benoit Ndiwe, Liliane Nga; interpretation of the MALDI ToF: Benoit Ndiwe and Antonio Pizzi; analysis and interpretation of results: Benoit Ndiwe, and Antonio Pizzi; first revisions of the manuscript: Jean Jalin Eyinga Biwole, Joseph Zobo Mfomo. All authors reviewed the results and approved the final version of the manuscript.
Availability of Data and Materials: Data available on request from the authors.
Conflicts of Interest: The authors declare that they have no conflicts of interest to report regarding the present study.
References
1. Pizzi, A. (2019). Tannins: Prospectives and actual industrial applications. Biomolecules, 9(8), 344. [Google Scholar] [PubMed]
2. Dorieh, A., Selakjani, P., Shahavi, M., Pizzi, A., Ghafari, M. et al. (2022). Recent developments in the performance of micro/nanoparticle-modified urea-formaldehyde resins used as wood-based composite binders: A review. International Journal of Adhesion and Adhesives, 114, 103106. [Google Scholar]
3. Boran, S., Usta, M., Gümüşkaya, E. (2011). Decreasing formaldehyde emission from medium density fiberboard panels produced by adding different amine compounds to urea formaldehyde resin. International Journal of Adhesion and Adhesives, 31(7), 674–678. [Google Scholar]
4. Stoeckel, F., Konnerth, J., Gindl-Altmutter, W. (2013). Mechanical properties of adhesives for bonding wood—A review. International Journal of Adhesion and Adhesives, 45, 32–41. [Google Scholar]
5. Mathaweesansurn, A., Detsri, E. (2022). A new colorimetric method for determination of formaldehyde in sea food based on anti-aggregation of gold nanoparticles. Journal of Food Composition and Analysis, 114, 104802. [Google Scholar]
6. Wink, M. (2013). Evolution of secondary metabolites in legumes (Fabaceae). South African Journal of Botany, 89, 164–175. [Google Scholar]
7. Oladeji, O., Adelowo, F., Oluyori, A. (2021). The genus Senna (FabaceaeA review on its traditional uses, botany, phytochemistry, pharmacology and toxicology. South African Journal of Botany, 138, 1–32. [Google Scholar]
8. Awouafack, M., Tane, P., Morita, H. (2017). Isolation and structure characterization of flavonoids. In: Justino, G. C. (Ed.Flavonoids-from biosynthesis to human health. InTechOpen. https://doi.org/10.5772/67881 [Google Scholar] [CrossRef]
9. El-Hashemy, M., Sallam, A. (2020). The inhibitive action of Calendula officinalis flower heads extract for mild steel corrosion in 1 M HCl solution. Journal of Materials Research and Technology, 9(6), 13509–13523. [Google Scholar]
10. Gomes, P., Gomes, E., Carvalho, A., Mannochio-Russo, H., Leão, T. et al. (2023). Characterization of a tetrameric proanthocyanidin in Stryphnodendron pulcherrimum and an overview on potential health benefits of condensed tannins via interaction with gut microbiota. Journal of Functional Foods, 109, 105801. [Google Scholar]
11. Li, H., Christman, L., Li, R., Gu, L. (2020). Synergic interactions between polyphenols and gut microbiota in mitigating inflammatory bowel diseases. Food and Function, 11(6), 4878–4891. [Google Scholar] [PubMed]
12. Cires, M., Wong, X., Carrasco-Pozo, C., Gotteland, M. (2017). The gastrointestinal tract as a key target organ for the health-promoting effects of dietary proanthocyanidins. Frontiers in Nutrition, 3, 57. [Google Scholar] [PubMed]
13. Shoji, T. (2014). Chemical properties, bioavailability, and metabolomics of fruit proanthocyanidins. In: Watson, R. R., Preedy, V. R., Zibadi, S., (Eds.Polyphenols in human health and diseases, vol. 1, pp. 339–351. Elsevier, NJ, USA: Academic Press. [Google Scholar]
14. Konai, N., Pizzi, A., Danwe, R., Lucien, M., Lionel, K. T. (2020). Thermomechanical analysis of African tannins resins and biocomposite characterization. Journal of Adhesion Science and Technology, 35, 1–8. [Google Scholar]
15. Pizzi, A. (2006). Recent developments in eco-efficient bio-based adhesives for wood bonding: Opportunities and issues. Journal of Adhesion Science and Technology, 20(8), 829–846. [Google Scholar]
16. Tchinda, J. B. S., Ndikontar, M. K., Belinga, A. D., Mounguengui, S., Njankouo, J. et al. (2018). Inhibition of fungi with wood extractives and natural durability of five Cameroonian wood species. Industrial Crops and Products, 123, 183–191. [Google Scholar]
17. Eyinga, J., Biwole, A., Pizzi, A., Zobo, J., Segovia, C. (2023). A review of the advances made in improving the durability of welded wood against water in light of the results of African tropical woods welding. Journal of Renewable Materials, 11(3), 1077–1099. [Google Scholar]
18. Bartolozzi, I., Daddi, T., Punta, C., Fiorati, A., Iraldo, F. (2020). Life cycle assessment of emerging environmental technologies in the early stage of development: A case study on nanostructured materials. Journal of Industrial Ecology, 24(1), 101–115. [Google Scholar]
19. Pizzi, A. (1983). Tannin-based adhesives. In: Wood adhesives chemistry and technology. New York, USA: Dekker. [Google Scholar]
20. Pizzi, A. (1994). Advanced wood adhesives technology. New York: Dekker. [Google Scholar]
21. Konai, N., Pizzi, A., Raidandi, D., Lagel, M. C., L’Hostis et al. (2015). Aningre (Aningeria spp.) tannin extract characterization and performance as an adhesive resin. Industrial Crops and Products, 77, 225–231. [Google Scholar]
22. Konai, N., Raidandi, D., Pizzi, A., Meva’a, L. (2017). Characterization of Ficus sycomorus tannin using ATR-FT MIR, MALDI-TOF MS and 13C NMR methods. European Journal of Wood and Wood Products, 75(5), 807–815. [Google Scholar]
23. Pasch, H., Pizzi, A., Rode, K. (2001). MALDI-TOF mass spectrometry of polyflavonoid tannins. Polymer, 42(18), 7531–7539. [Google Scholar]
24. Saad, H., Khoukh, A., Ayed, N., Charrier, B., Charrier-El Bouhtoury, F. (2014). Characterization of Tunisian Aleppo pine tannins for a potential use in wood adhesive formulation. Industrial Crops and Products, 61(11), 517–525. [Google Scholar]
25. Navarrete, P., Pizzi, A., Pasch, H., Rode, K., Delmotte, L. (2010). MALDI-TOF and 13C NMR characterization of maritime pine industrial tannin extract. Industrial Crops and Products, 32(2), 105–110. [Google Scholar]
26. Meikleham, N., Pizzi, A., Stephanou, A. (1994). Induced accelerated autocondensation of polyflavonoid tannins for phenolic polycondensates, Part 1: 13C NMR, 29Si NMR, X-ray and polarimetry studies and mechanism. Journal of Applied Polymer Science, 54, 1827–1845. [Google Scholar]
27. Navarrete, P., Pizzi, A., Pasch, H., Rode, K., Delmotte, L. (2013). Characterization of two maritime pine tannins as wood adhesives. Journal of Adhesion Science and Technology, 27(22), 2462–2479. [Google Scholar]
28. Garnier, S., Pizzi, A., Huang, Z. (2002). Dry IB forescasting of commercial tannin adhesives-bonded particleboard by TMA bending. European Journal of Wood and Wood Products, 60(5), 372. [Google Scholar]
29. Pizzi, A. (1997). On the correlation of some theoretical and experimental parameters in polycondensation cross-linked networks. Journal of Applied Polymer Science, 63(5), 603–617. [Google Scholar]
30. Gérard, J., Guibal, D., Paradis, S., Vernay, M., Beauchêne, J. et al. (2011). Caractéristiques technologiques de 245 essences tropicales et tempérées. Tropix. Montpellier, France. [Google Scholar]
31. Bikoro Bi Athomo, A., Engozogho, S. P., Anris, Safou, R. T., Eyma, F. (2021). Identification of phenolic compounds from K. ivorensis by selected chromatographic and spectrometric techniques. Journal of Renewable Materials, 9(1), 35–48. https://doi.org/10.32604/jrm.2021.013626 [Google Scholar] [CrossRef]
32. Feria-Reyes, R., Ramírez-Cruz, S., Ruiz-Aquino, F., Robledo-Taboada, L., Sánchez-Medina, M. et al. (2023). Pine bark as a potential source of condensed tannin: Analysis through fourier transform infrared spectroscopy (FTIRscanning electron microscopy (SEMand energy dispersive X-ray (EDX). Forests, 14(7), 1433. [Google Scholar]
33. Saha, J. B. T., Daouda, A., Dumarçay, S., Ndikontar, M., Gérardin, P. et al. (2013). Antioxidant activities, total phenolic contents and chemical compositions of extracts from four Cameroonian woods: Padouk (Pterocarpus soyauxii TaubbTali (Erythrophleum suaveolensMoabi (Baillonella toxispermaand Movingui (Distemonanthus benthamianus). Industrial Crops and Products, 41, 71–77. [Google Scholar]
34. Ndiwe, B., Pizzi, A., Tibi, B., Danwe, R., Konai, N. et al. (2019). African tree bark exudate extracts as biohardeners of fully biosourced thermoset tannin adhesives for wood panels. Industrial Crops and Products, 132, 253–268. [Google Scholar]
35. Wedaïna, G., Pizzi, A., Nzie, W., Danwe, R., Konai, N. et al. (2021). Performance of unidirectional biocomposite developed with Piptadeniastrum Africanum tannin resin and Urena Lobata fibers as reinforcement. Journal of Renewable Materials, 9(3), 477–493. [Google Scholar]
36. Zhang, L. L., Lin, Y. M., Zhou, H. C., Wei, S. D., Chen, J. H. (2010). Condensed tannins from mangrove species Kandelia candel and Rhizophora mangle and their antioxidant activity. Molecules, 15(1), 420–431, 2010. [Google Scholar] [PubMed]
37. Davis, A. L., Cai, Y., Davies, A. P., Lewis, J. (1996). 1H and 13C NMR assignments of some green tea polyphenols. Magnetic Resonance in Chemistry, 34(11), 887–890. [Google Scholar]
38. Pizzi, A., Pasch, H., Rode, K., Giovando, S. (2009). Polymer structure of commercial hydrolyzable tannins by matrix-assisted laser desorption/ionization-time-of-flight mass spectrometry. Journal of Applied Polymer Science, 113(6), 3847–3859. [Google Scholar]
39. Hashida, K., Makino, R., Ohara, S. (2009). Amination of pyrogallol nucleus of condensed tannins and related polyphenols by ammonia water treatment. Holzforschung, 63(3), 319–326. [Google Scholar]
40. Braghiroli, F., Fierro, V., Pizzi, A., Rode, K., Radke, W. et al. (2013). Reaction of condensed tannins with ammonia. Industrials Crops and Products, 44, 330–335. [Google Scholar]
41. Santiago-Medina, F. J., Pizzi, A., Basso, M., Delmotte, L., Celzard, A. (2017). Polycondensation resins by flavonoid tannins reaction with amines. Polymers, 9(2), 37. [Google Scholar] [PubMed]
42. Zhang, B., Chen, X., Pizzi, A., Petrissans, M., Dumarcay, S. et al. (2023). Highly branched tannin-tris (2-aminoethyl) amine-urea wood adhesives. Polymers, 15(4), 890. [Google Scholar] [PubMed]
43. Njom, A. E., Mewoli, A., Ndengue, M. J., Ebanda, E. F., Nitidem, A. D. (2022). Hybid composite based on natural rubber reinforced with short fibers of the triumfetta cordifolia/Saccharum officinarum L.: Performance evaluation. Journal of Minerals and Materials Characterization and Engineering, 10(5), 385–399. [Google Scholar]
44. Ndiwe, B., Konai, N., Pizzi, A., Karga, L., Kaoutoing, M. D. et al. (2022). Mechanical performance of a particleboard based on natural hardener. Wood Material Science & Engineering, 18, 1–9. [Google Scholar]
Cite This Article
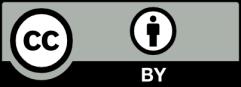
This work is licensed under a Creative Commons Attribution 4.0 International License , which permits unrestricted use, distribution, and reproduction in any medium, provided the original work is properly cited.