Open Access
ARTICLE
Optimizing Household Wastes (Rice, Vegetables, and Fruit) as an Environmentally Friendly Electricity Generator
1 Department of Chemistry, Faculty of Mathematics and Natural Sciences, Universitas Negeri Malang, Malang, 65145, Indonesia
2 Chemistry and PKWU, SMAN 3 Sidoarjo, Sidoarjo, 61215, Indonesia
* Corresponding Author: Sumari Sumari. Email:
(This article belongs to the Special Issue: Special Issue in Celebration of JRM 10 Years)
Journal of Renewable Materials 2024, 12(2), 275-284. https://doi.org/10.32604/jrm.2023.043419
Received 02 July 2023; Accepted 09 November 2023; Issue published 11 March 2024
Abstract
The high consumption of electricity and issues related to fossil energy have triggered an increase in energy prices and the scarcity of fossil resources. Consequently, many researchers are seeking alternative energy sources. One potential technology, the Microbial Fuel Cell (MFC) based on rice, vegetable, and fruit wastes, can convert chemical energy into electrical energy. This study aims to determine the potency of rice, vegetable, and fruit waste assisted by Cu/Mg electrodes as a generator of electricity. The method used was a laboratory experiment, including the following steps: electrode preparation, waste sample preparation, incubation of the waste samples, construction of a reactor using rice, vegetable, and fruit waste as a source of electricity, and testing. The tests included measuring electrical conductivity, electric current, voltage, current density, and power density. Based on the test results, the maximum current and voltage values for the fruit waste samples were 5.53 V and 11.5 mA, respectively, with a current density of 2.300 mA/cm2 and a power density of 12.719 mW/cm2. The results indicate the potential for a future development. The next step in development involves determining the optimum conditions for utilizing of rice, vegetable, and fruit waste. The results of the electrical conductivity test on rice, vegetable, and fruit waste samples were 1.51, 2.88, and 3.98 mS, respectively, with the highest electrical conductivity value found in the fruit waste sample.Keywords
Nomenclature
MFC | Microbial Fuel Cell |
CD | Current Density |
PD | Power Density |
The high consumption of electrical energy has caused problems in the 21st century. Indonesian electricity consumption was 1,133 kilowatt hours (kWh) per capita in 2020, and it increased to 1,173 kWh/capita in 2022 [1]. Fossil products such as oil, gas and coal have been extensively exploited, leading to a low availability of fossil resources in the coming years. The consequences of this include rising energy prices and the depletion of fossil resources. Furthermore, the environment has been polluted due to harmful emissions from fossil fuels. These challenges have prompted us to search for alternative, renewable and environmentally friendly energy sources.
The development of alternative energy sources has been pursued by numerous researchers to reduce reliance on fossil resources. One promising technology with the potential for widespread, cost-effective, and environmentally friendly use is the Microbial Fuel Cell (MFC) [2]. MFC is an applied science involving electrochemical cells that rely on specific microbes to convert chemical energy into electrical energy by degrading organic materials and producing electrons [3]. Many organic materials can be utilized in MFC, but they must be abundant, renewable, and not compete with essential primary needs [4]. One of the materials as a source of electrical energy is household waste which can come from leftover rice, fruits, and vegetables [5].
Several types of fruit and vegetables in household wastes that have acidic properties are tomatoes, carrots, oranges, chilies, kale, spinach, and mustard greens [6]. However, they usually become unutilized household wastes. Household wastes, including leftover rice, rotting fruits and vegetables, are often found in homes and even in vegetable and fruit markets [7]. The wastes are typically discarded, leading to unpleasant odors and unsightly conditions [8]. They are generated as a result of human activities or natural processes, lack economic value, and can contribute to environmental pollution [9]. Therefore, rice, vegetable and fruit wastes can serve as suitable substrates for MFC [10]. Such potential household waste can offer an alternative source of electrical energy in commercial activities and daily household needs, such as lighting and act as a backup during power outages.
MFC functions based on the principles of a galvanic cell. When two metals are immersed in an electrolyte solution, a spontaneous reduction-oxidation reaction occurs, resulting in the generation of electric current [11]. This concept can be applied to using fruits as an alternative source of electrical energy. A voltaic cell or galvanic cell generates electric current through a chemical reaction [12]. When atoms in electrodes lose or gain electrons, they become positively or negatively charged, creating a potential energy difference between them [13]. By connecting a conducting wire between electrodes with different charges, energy transfer occurs, maintaining an electric voltage as long as a potential difference exists. This electric voltage can be generated through an electric generator in an electric circuit, defined as the potential difference between two points [14].
Numerous previous studies have reported the use of biomass-based substrates such as agricultural wastes [15], and organic fruit wastes [16], in microbial fuel cell (MFC) systems. The choice of substrates plays a crucial role in the efficiency and application of MFC [17]. Thus, there is a need to explore and develop the most suitable substrate [4]. Additionally, MFC performance must be optimized and integrated with other technologies. For instance, single-chamber MFC technology is preferred due to its simplicity and cost-effectiveness [18]. In this study, a modified MFC was created, connected in a series circuit of a single chamber [19], representing a scale-up of MFC technology. The progress of this study contributes significantly to the advancement and application of MFC systems. In a previous study, a modified MFC using Cu/Mg electrodes for banana stem waste generated 0.5 V of electricity [19]. In this work, household wastes can contribute to the sustainability of MFC substrates. Based on the information presented, this study evaluates the potential of “Optimization of Household Wastes (Rice, Vegetables, and Fruit) as an Environmentally Friendly Electricity Generator”. This study aims to determine the potency of rice, vegetable, and fruit wastes assisted by Cu/Mg electrodes as a generator of electricity.
This research required several tools, i.e., a cup (50 mL), a knife, a mortar, a pestle, a 500 mL Beaker, plastic wrap, a cable, an LED lamp, a multi-tester. The materials used included distilled water, rice waste, vegetable wastes which consisted of chicory, kale, and spinach, as well as fruit wastes including oranges, tomatoes, and watermelons. Additionally, Cu electrodes and Mg electrodes, each measuring 5 cm × 1 cm × 0.1 cm, were employed along with lights.
2.2 Preparation of Waste Samples
Waste rice, vegetables (chili, kale, spinach) and fruits (oranges, tomatoes, watermelon) were initially crushed using a mortar and pestle. The mixture was then filtered to extract the essence. The resulting smooth sample was subsequently incubated for seven days by placing it in a container and tightly sealing it. This seven-day incubation period allowed for the decomposition and degradation of the rice, vegetable and fruit waste to progress, ultimately producing electrons through the oxidation reaction.
2.3 Performance of the Circuit
This research was conducted following a series of circuits (Figs. 1 and 2) using various types of waste extracts under ambient conditions. The circuit design was modified from a previous study [20]. Each circuit unit consisted of Cu and Mg electrodes, waste sample extracts, and wires connecting circuits that were placed close to each other. Four units were used for a single circuit performance. The waste sample extracts included 50 mL of rice waste extract, 50 mL of vegetable waste extract, and 50 mL of fruit waste extract with a testing duration of 10 days. Additionally, 10 mL of waste extract was extracted to test the electrical conductivity of the electrolyte material derived from the waste.
Figure 1: Series of circuits
Figure 2: One unit of the circuit
2.4 Measurement of Current Density and Power Density
To measure the amount of electrical energy produced in this study, a multi-tester was employed to measure conductivity, voltage and current. Prior to taking measurements, the multi-tester was calibrated. Data collection was conducted over varying time intervals. The data, including current and voltage, were processed to determine the current density (CD) (mA/m2), representing the current per unit area of the electrode surface [21], and power density (PD) (mW/m2) indicating the power per unit area of the electrode surface [22]. Current density and power density were calculated using Eqs. (1) and (2), respectively [19].
3.1 Optimization of Waste Samples (Rice, Vegetables, and Fruit) as Electrolytes
In this study, rice, vegetable and fruit waste, including oranges, chilies, carrots, kale, mustard greens and spinach were used as electrolytes. Distilled water (500 grams) was placed in a sealed container to degrade the waste samples. As a result, the solution generated electrons through an oxidation reaction. Consequently, waste samples of rice, fruits and vegetables became more reactive with the electrodes, leading to higher voltage outputs compared to fresh rice, vegetables, and fruits.
Rice waste, as an electrolyte, contains electrically conductive substances, such as carbohydrates, water (H2O), vitamins (B1, B2, B3, and B6), protein, iron, phosphorus, selenium, manganese, magnesium, starch, and amylopectin. The degradation and incubation processes of rice samples are in a low level of acidity (high pH), leading to the production of fewer ions and, consequently, a smaller electric current and conductivity [23].
After incubating waste samples of vegetables and fruits for 7 days, the acidity of the fruits and vegetables increased due to the fermentation process, resulting in higher electrolytes [24]. Essentially, electrical energy is obtained from various sources, including fruits and vegetables [23]. Waste samples of vegetables and fruits can be categorized as reactive elements that conduct electricity. Electrolytes that dissociate completely in water are referred to as strong electrolytes, while those that only partially dissociate in water are known as weak electrolytes.
Electrical conductivity in a solution is a value of electrical conductivity depending on the type and concentration of ions in a solution. Free ions have a large electrical conductivity. The conductivity of a solution is highly dependent on the concentration of ions and the temperature of the water. The greater the value of electrical conductivity, the stronger the ability to conduct electricity [25].
In this study, an electrical conductivity test was carried out for samples of rice, vegetable, and fruit wastes. The following results were obtained, as shown in Fig. 3. Based on Fig. 3, the electrical conductivity values in the three samples were measured at 23°C. The electric current generated in the three wastes can be defined as the flow of electrons in a conductor (conducting ion) within a certain time. The more acidic a solution, the more ions it produces. In other words, the solution will be more electrolyte. Conversely, the weaker the acidity of the solution, the fewer ions will be produced, so the conductivity will decrease (the electric current produced will be weaker).
Figure 3: Conductivity of rice, vegetable, and fruit wastes
Fig. 3 shows that the result of the fruit waste (average: 3.98 mS) is higher than the samples of vegetable waste (average: 2.88 mS) and rice waste (average: 1.52 mS). In fruit waste, 500 grams of citrus, chili and tomato waste contain various substances conducting electricity, such as vitamins, iron and phosphorus in tomatoes, and contain vitamin C, vitamin B1, vitamin B2, calcium, phosphorus, potassium, iron, copper and other substances in citrus. The content of citric acid in fruit waste produces cellular energy and increases the acidity value [26], when the fruit and vegetables have rotted because the fermentation and incubation process produce more acid, thereby increasing the strength of the electrolyte in the waste of citrus, chilies, and tomatoes.
In vegetable wastes, kale, mustard greens, and spinach have the second highest electrical conductivity value after fruit waste and they are higher than rice waste. Vegetable wastes contain a small amount of citric acid, which produces cell energy and can increase in pH, if it is stored for a long time. Meanwhile, rice contains a lot of carbohydrates and calories, so the electrolytes produced are lower than those produced by fruit and vegetable waste, which contain a lot of electrolytes from reactive elements.
Compared with some of the mineral content of spinach, tomatoes, carrots, and mustard greens, it can produce electrical properties because of several hydrosoluble vitamins such as vitamin B1 (Thiamin), vitamin B2 (Riboflavin), vitamin B3 (Niacin), vitamin B6, vitamin B5 (Pantothenic Acid) [27]. The content of vitamin C can conduct electricity because the constituent elements of this vitamin are strong acids that decompose in solution easily [28]. These results are based on the electrical properties that only involve the transport of electrons which are separated by electrodes in a conductive medium or an electrolyte providing the driving force of the electrons (electric potential and electric current). At the electrode, the electrons presented in the ion samples undergo electrolysis. Electrolysis means a chemical change produced by passing an electric current through an electrolyte solution [29].
3.3 Testings of Waste Samples as Electricity Generator
In this study, the circuit design was connected in series. The anode used magnesium metal and the cathode used copper metal. Each MFC system in a series circuit will work individually, so the total resulting higher current and voltage. Current and voltage values measured can be seen in Fig. 4, while external resistance applied to the system was not measured.
Figure 4: Voltage, current, and power values of rice, vegetable, and fruit wastes vs. time
The highest current and voltage values in the fruit wastes were shown after 48 h, namely 11.5 mA and 5.53 V. The strong relationship between electric current and fruit acidity is inversely proportional. The more acidic of fruit sample, the stronger the electric current in the solution, and vice versa. The more alkaline of fruit sample, the smaller the electric current in the solution. Current and voltage testings are intended to analyze the effectiveness of rice, vegetable, and fruit wastes as a source of electrical energy. Before the testing, it was prepared them by mixing with distilled water and incubation for seven days.
There is a gradual decrease in both current and voltage. The voltage and electric current generated in this study can be explained by principle of a voltaic cell. If two different electrodes are placed in an electrolyte solution, it will produce electrical energy as a result of a chemical reaction spontaneously. The results of the current and voltage are then processed into current density (Table 1) and power density (Table 2).
The current density measurement was carried out using Eq. (1), and it was found that fruit waste produced the largest current density of 2.300 mA/cm2 compared to rice and vegetable waste samples.
The power density measurement was carried out using Eq. (2), and it was found that fruit waste produced the largest power density of 12.719 mW/cm2 compared to rice and vegetable waste samples. The values correlated with the current density values. On the other hand, the values decreased after 72 h. This observation was also obtained by a previous study [30]. A comparison of the previous study with this study can be listed in Table 3. However, this study has different conditions of the performance of the circuit, like pH, electrodes, and temperature.
Based on Table 3, this study has a potential application. Using magnesium electrodes as anodes has oxidized the fruit wastes which is more than using zinc electrodes. However, the magnesium electrode did not perform well enough to oxidize banana stem waste. It is caused by different compositions, so fruits, vegetable wastes, and rice wastes are easier to oxidize than banana stem waste.
Based on the results of the research, the maximum current and voltage values obtained by the fruit waste samples were 5.53 V and 11.5 mA with a current density of 2.30 mA/cm2 and a power density of 12.719 mW/cm2. The results show that there is potential for future development. The next development needs to determine the optimum conditions for the utilization of rice, vegetable, and fruit wastes in order to obtain a maximum and stable power density. The results of the electrical conductivity on rice, vegetable, and fruit waste samples were 1.52, 2.88, and 3.98 mS, respectively, so that the highest electrical conductivity value was in the fruit waste sample. For future perspective, the study needs to explore the waste management of household waste, analyze microbes in the waste, compare household waste with industrial waste, design the circuit on the pilot scale, and mix the household waste as electrolytes.
Acknowledgement: Thanks to Department of Chemistry, Universitas Negeri Malang for supporting this study.
Funding Statement: The authors received no specific funding for this study.
Author Contributions: The authors confirm contribution to the paper as follows: study conception and design: Deni Ainur Rokhim, Sumari Sumari, Yudhi Utomo; data collection: Deni Ainur Rokhim, Isma Yanti Vitarisma; analysis and interpretation of results: Deni Ainur Rokhim, Muhammad Roy Asrori; draft manuscript preparation: Deni Ainur Rokhim, Muhammad Roy Asrori. All authors reviewed the results and approved the final version of the manuscript.
Availability of Data and Materials: The authors confirm that the data supporting the findings of this study are available within the article.
Conflicts of Interest: The authors declare that they have no conflicts of interest to report regarding the present study.
References
1. Secretariate General The National Energy Council (2021). Indonesia energy outlook 2021 (In Indonesia). Indonesia: National Energy Council. [Google Scholar]
2. Naha, A., Debroy, R., Sharma, D., Shah, M. P., Nath, S. (2023). Microbial fuel cell: A state-of-the-art and revolutionizing technology for efficient energy recovery. Cleaner and Circular Bioeconomy, 5, 100050. https://doi.org/10.1016/j.clcb.2023.100050 [Google Scholar] [CrossRef]
3. Dilip Kumar, S., Yasasve, M., Karthigadevi, G., Aashabharathi, M., Subbaiya, R. et al. (2022). Efficiency of microbial fuel cells in the treatment and energy recovery from food wastes: Trends and applications—A review. Chemosphere, 287, 132439. https://doi.org/10.1016/j.chemosphere.2021.132439 [Google Scholar] [PubMed] [CrossRef]
4. Sonawane, J. M., Mahadevan, R., Pandey, A., Greener, J. (2022). Recent progress in microbial fuel cells using substrates from diverse sources. Heliyon, 8(12), e12353. https://doi.org/10.1016/j.heliyon.2022.e12353 [Google Scholar] [PubMed] [CrossRef]
5. Kasipandian, K., Saigeetha, S., Samrot, A. V., Abirame, S., Renitta, R. E. et al. (2021). Bioelectricity production using microbial fuel cell–A review. Biointerface Research in Applied Chemistry, 11(2), 9420–9431. https://doi.org/10.33263/BRIAC112.94209431 [Google Scholar] [CrossRef]
6. Rashid, F., Joardder, M. U. H. (2022). Future options of electricity generation for sustainable development: Trends and prospects. Engineering Reports, 4(10), e12508. https://doi.org/10.1002/eng2.12508 [Google Scholar] [CrossRef]
7. Lau, K. Q., Sabran, M. R., Shafie, S. R. (2021). Utilization of vegetable and fruit by-products as functional ingredient and food. Frontiers in Nutrition, 8, 661693. https://doi.org/10.3389/fnut.2021.661693 [Google Scholar] [PubMed] [CrossRef]
8. Nguyen, T., van den Berg, M.,Nguyen, M. (2023). Food waste in primary schools: Evidence from peri-urban Viet Nam. Appetite, 183, 106485. https://doi.org/10.1016/j.appet.2023.106485 [Google Scholar] [PubMed] [CrossRef]
9. Roy, P., Mohanty, A. K., Dick, P., Misra, M. (2023). A review on the challenges and choices for food waste valorization: Environmental and economic impacts. ACS Environmental Au, 3(2), 58–75. https://doi.org/10.1021/acsenvironau.2c00050 [Google Scholar] [PubMed] [CrossRef]
10. Chaturvedi, V., Verma, P. (2016). Microbial fuel cell: A green approach for the utilization of waste for the generation of bioelectricity. Bioresources and Bioprocessing, 3(1), 38. https://doi.org/10.1186/s40643-016-0116-6 [Google Scholar] [CrossRef]
11. Dange, P., Savla, N., Pandit, S., Bobba, R., Jung, S. P. et al. (2022). A comprehensive review on oxygen reduction reaction in microbial fuel cells. Journal of Renewable Materials, 10(3), 665–697. https://doi.org/10.32604/jrm.2022.015806 [Google Scholar] [CrossRef]
12. Gunawan, Widodo, D. S., Haris, A., Suyati, L., Hadi, S. P. et al. (2019). Energy storage system from galvanic cell using electrolyte from a plant as an alternative renewable energy. IOP Conference Series: Materials Science and Engineering, 509(1), 12045. https://doi.org/10.1088/1757-899X/509/1/012045 [Google Scholar] [CrossRef]
13. Chaudhary, N., Khanuja, M. (2022). 2-Electrochemistry—concepts and methodologies. In: Maruccio, G., Narang, J. B. T. E. S. (Eds.Woodhead publishing series in electronic and optical materials, pp. 31–50. UK: Woodhead Publishing. https://doi.org/10.1016/B978-0-12-823148-7.00002-7 [Google Scholar] [CrossRef]
14. Vujević, S., Modrić, T., Lovrić, D. (2011). The difference between voltage and potential difference. Proceedings of the Joint INDS’11 & ISTET’11, vol. 11, pp. 1–7. https://doi.org/10.1109/INDS.2011.6024799 [Google Scholar] [CrossRef]
15. Flores, S. R., Naveda, R. N., Paredes, E. A., Orbegoso, J. A., Céspedes, T. C. et al. (2020). Agricultural wastes for electricity generation using microbial fuel cells. The Open Biotechnology Journal, 14, 52–58. https://doi.org/10.2174/1874070702014010052 [Google Scholar] [CrossRef]
16. Segundo, R. F., Renny, N. N., Moises, G. C., Daniel, D. N., Natalia, D. D. et al. (2021). Generation of bioelectricity from organic fruit waste. Journal of Environmental Research, Engineering and Management, 77(3), 6–14. https://doi.org/10.5755/j01.erem.77.3.28493 [Google Scholar] [CrossRef]
17. Wang, J., Ren, K., Zhu, Y., Huang, J., Liu, S. (2022). A review of recent advances in microbial fuel cells: Preparation, operation, and application. BioTech, 11(4), 44. https://doi.org/10.3390/biotech11040044 [Google Scholar] [PubMed] [CrossRef]
18. Jinisha, R., Regin, J. J., Maheswaran, J. (2020). A review on the emergence of single-chamber microbial fuel cell on wastewater treatment. IOP Conference Series: Materials Science and Engineering, 983(1), 12002. https://doi.org/10.1088/1757-899X/983/1/012002 [Google Scholar] [CrossRef]
19. Rokhim, D. A., Adid, A. W., Tyas, F. K., Ciptawati, E., Asrori, M. R. (2022). Utilization of banana stem waste extracts assisted by electrode of Cu/Mg as an environmentally friendly electricity producer. Orbital: The Electronic Journal of Chemistry, 14(1), 40–44. https://doi.org/10.17807/orbital.v14i1.1677 [Google Scholar] [CrossRef]
20. Hidayat, S., Aghnia, D. W., Kardena, E., Helmy, Q. (2020). Kinerja Microbial Fuel Cell dengan Variasi Hambatan Eksternal dalam Menghasilkan Energi Listrik dan Menyisihkan Senyawa Organik pada Limbah Cair. Jurnal Presipitasi : Media Komunikasi dan Pengembangan Teknik Lingkungan, 17(3), 223–232. https://doi.org/10.14710/presipitasi.v17i3.223-232 [Google Scholar] [CrossRef]
21. Foad Marashi, S. K., Kariminia, H. R. (2015). Performance of a single chamber microbial fuel cell at different organic loads and pH values using purified terephthalic acid wastewater. Journal of Environmental Health Science and Engineering, 13(1), 27. https://doi.org/10.1186/s40201-015-0179-x [Google Scholar] [PubMed] [CrossRef]
22. Choudhury, P., Bhunia, B., Bandyopadhyay, T. K., Ray, R. N. (2021). The overall performance improvement of microbial fuel cells connected in series with dairy wastewater treatment. Journal of Electrochemical Science and Technology, 12(1), 101–111. https://doi.org/10.33961/jecst.2020.01284 [Google Scholar] [CrossRef]
23. Meiliyadi, L. A. D., Damayanti, I., Syuzita, A. (2023). Characteristic of ceremai fruit electrical properties as electrolyte solution in galvanic cells. Jurnal Penelitian Pendidikan IPA, 9(2), 478–484. https://doi.org/10.29303/jppipa.v9i2.1880 [Google Scholar] [CrossRef]
24. Vassel, S., Vassel, N., Vertiy, N., Kovalevskii, V. (2021). Electricity from salty and fresh water: The concept of concentration galvanic cell with Cl2/Cl–electrodes. JP Journal of Heat and Mass Transfer, 23(1), 29–33. https://doi.org/10.17654/HM023010029 [Google Scholar] [CrossRef]
25. Gapparov, A., Isakova, M. (2023). Study on the characteristics of water resources through electrical conductivity: A case study of Uzbekistan. IOP Conference Series: Earth and Environmental Science, 1142(1), 12057. https://doi.org/10.1088/1755-1315/1142/1/012057 [Google Scholar] [CrossRef]
26. Rahman, W., Yusup, S., Mohammad, S. N. A. A. (2021). Screening of fruit waste as substrate for microbial fuel cell (MFC). AIP Conference Proceedings, 2332(1), 020003. https://doi.org/10.1063/5.0043556 [Google Scholar] [CrossRef]
27. Yaman, M., Çatak, J., Uğur, H., Gürbüz, M., Belli, İ. et al. (2021). The bioaccessibility of water-soluble vitamins: A review. Trends in Food Science & Technology, 109, 552–563. https://doi.org/10.1016/j.tifs.2021.01.056 [Google Scholar] [CrossRef]
28. Assiry, A. M., Sastry, S. K., Samaranayake, C. P. (2006). Influence of temperature, electrical conductivity, power and pH on ascorbic acid degradation kinetics during ohmic heating using stainless steel electrodes. Bioelectrochemistry, 68(1), 7–13. https://doi.org/10.1016/j.bioelechem.2005.02.005 [Google Scholar] [PubMed] [CrossRef]
29. Rustana, C. E., Sunaryo, Muchtar, S. J., Sugihartono, I., Sasmitaningsihhiadayah, W. et al. (2021). The effect of voltage and electrode types on hydrogen production from the seawater electrolysis process. Journal of Physics: Conference Series, 2019(1), 12096. https://doi.org/10.1088/1742-6596/2019/1/012096 [Google Scholar] [CrossRef]
30. Prasidha, W., Majid, A. I. (2020). Electricity production from food waste leachate (fruit and vegetable waste) using double chamber microbial fuel cell: Comparison between non-aerated and aerated configuration. Rotasi, 22(3), 162–168. https://doi.org/10.14710/rotasi.22.3.162-168 [Google Scholar] [CrossRef]
31. Khan, A. M., Obaid, M. (2015). Comparative bioelectricity generation from waste citrus fruit using a galvanic cell, fuel cell and microbial fuel cell. Journal of Energy in Southern Africa, 26(3), 90–99. https://doi.org/10.17159/2413-3051/2015/v26i3a2143 [Google Scholar] [CrossRef]
Cite This Article
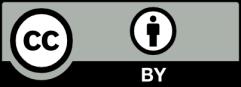
This work is licensed under a Creative Commons Attribution 4.0 International License , which permits unrestricted use, distribution, and reproduction in any medium, provided the original work is properly cited.