Open Access
ARTICLE
Improving Antioxidant Properties of Chitosan Films by Incorporating Extracted Fractions of Propolis
Instituto Universitario de Ingeniería de Alimentos (FoodUPV), Universitat Politècnica de València, Camino de Vera s/n, Valencia, 46022, España
* Corresponding Author: Amparo Chiralt. Email:
(This article belongs to the Special Issue: Special Issue in Celebration of JRM 10 Years)
Journal of Renewable Materials 2024, 12(12), 1949-1962. https://doi.org/10.32604/jrm.2024.056404
Received 22 July 2024; Accepted 23 September 2024; Issue published 20 December 2024
Abstract
Chitosan films with propolis aqueous extract were obtained in order to improve the antioxidant capacity of the materials. Likewise, oleic acid (OA) was also incorporated to enhance the film hydrophobicity, with and without previous infusion with propolis, to extract other potentially active compounds. The propolis extracts and the chitosan film-forming dispersions were characterised as to their particle size distribution, zeta potential and rheological behaviour. Chitosan-based films with aqueous extract and with oleic acid, containing or not propolis compounds, were obtained and characterised as to their microstructure, mechanical, barrier and optical properties and antioxidant capacity in order to evaluate their ability to extend the food shelf life. The propolis compounds from the aqueous extract notably modified the chitosan conformation and charge density of chitosan molecules, giving rise to more folded chains with a milder viscous effect. These changes in the polymer gave rise to a coarser film microstructure, which exhibited greater stiffness, and less stretchability and resistance to break, but a greater barrier capacity to water vapour and oxygen. These films had a ten-fold greater antioxidant capacity, thus having great potential to preserve food from oxidative spoilage. The incorporation of OA, containing or not propolis compounds, into these films reduced the film stiffness and resistance to break, and led to a highly enhanced oxygen permeability without there being any notable changes in either the water vapour barrier capacity or antioxidant power.Graphic Abstract
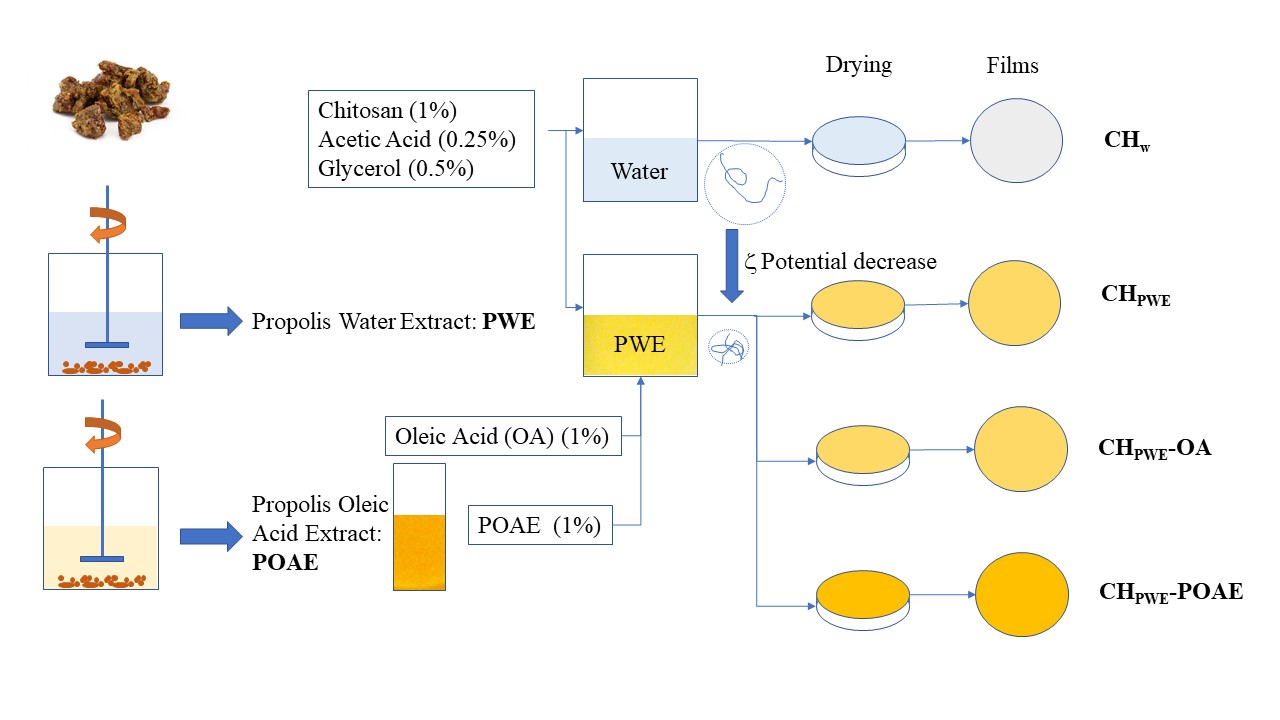
Keywords
Propolis is a natural product that honeybees (Apis mellifera L.) obtained from the resinous fractions of the cracks of leaf buds and tree barks. This complex composition depends on the plant components and collection period. Propolis is used for different curative purposes as an antimicrobial (against bacteria, fungi, viruses, and protozoa), anti-inflammatory, and antitumoral agent. It also has antioxidant, anaesthetic, anticancer, antiseptic, anti-mutagenic, anti-heptatoxic and cytotoxic activity [1]. As the trend towards natural remedies is growing, this product has become a popular alternative and complementary medicinal product [2].
The main constituents of propolis are resin and vegetal balsam (50%), whose main compounds are flavonoids and phenolic acids, waxes (30%), and essential and aromatic oils (10%). It also contains a minor proportion of pollen (5%) and other organic compounds, such as proteins, sugars, amino acids, minerals and vitamins (5%) [1,3]. Flavonoids are the major phenolic compounds of propolis, which greatly contribute to its biological/functional properties. Of the phenolic acids, cinnamic, ferulic, p-cumaric, caffeic, benzoic, and salicylic acids are the main constituents [4]. Other basic constituents are saturated and unsaturated hydrocarbons, aliphatic and aromatic esters, fatty acids (mainly oleic, palmitic, linoleic and stearic) and steroids. Likewise, different uronic acids, sugars, and sugar alcohols are also found [4].
Different types of propolis have been identified around the world. Based on their main constituents and plant origin, there are seven main classified types of propolis with some compositional particularities [5]. In its non-volatile fraction, the main bioactive compounds found in Mediterranean propolis contain flavonoids, such as pinocembrin, galangin, naringenin, acacetin and chrysin [6], while some sesquiterpenes, such as β-eudesmol, and monoterpenes, such as α-pinene, constitute the volatile fraction [5].
Ethanolic extraction has mainly been used in the preparation of commercial propolis products, since the bioactive compounds are mainly soluble in this solvent and around 90% of the flavonoids and many phenolic acids, such as p-coumaric acid, are extracted [7]. However, ethanol-based propolis extracts have limited use, and an alternative green extraction process would expand their application in new fields [8]. In this sense, previous studies point to the use of water, lipids or surfactants as more suitable alternatives [8]. The concentration of hydroxycinnamic acids (p-coumaric, ferulic and caffeic acids) in aqueous propolis extracts obtained by applying microwave-assisted extraction did not differ significantly from the concentration determined in ethanolic propolis extracts [8].
On the basis of propolis bioactivity, some studies have analysed their potential for developing active food packaging/coating materials, using different polymers and propolis ethanolic extracts [9–11]. The use of chitosan represents an interesting alternative due to its good film-forming ability and its intrinsic antimicrobial properties [12–14].
Segueni et al. [11] reviewed previous studies carried out on chitosan films with incorporated propolis ethanolic extracts and reported the enhanced antimicrobial and antioxidant capacity of these chitosan films, which points to their potential for food preservation applications in order to prevent microbial or oxidative food spoilage. Likewise, the ethanolic propolis extract improved the mechanical and barrier properties of the chitosan films when incorporated in different ratios. The water vapour and oxygen permeability values decreased when the propolis concentration rose, which was attributed to the interactions between the functional groups of chitosan and polyphenols. de Carli et al. [10] also found that there was a greater thermal stability of chitosan when the films contained propolis extract. Both the type of chitosan (with different molecular weights or acetylation degrees) and the propolis extract concentration affected the film properties [12]. The best antimicrobial activity and barrier capacity were observed for the films consisting of high molecular weight chitosan and 5% propolis extract. Stefanowska et al. [14] also reported an excellent antiradical effect, comparable to Trolox (92.8%), for solutions of chitosan and ethanolic propolis extracts.
In the present study, the potential of green propolis aqueous extract to improve the antioxidant capacity of chitosan films was analysed. Moreover, oleic acid was incorporated to improve the film hydrophobicity, with and without a previous infusion with propolis, to extract other potentially active compounds. Therefore, propolis extracts were obtained in water and in oleic acid, and the chitosan film-forming dispersions with both extracts were characterised as to their relevant stability properties, such as particle size distribution, zeta potential and rheological behaviour. Likewise, chitosan-based films with aqueous extract and with oleic acid (containing or not propolis compounds) were obtained and characterised as to their microstructure, their mechanical, barrier and optical properties and their antioxidant capacity in order to evaluate their ability to extend the shelf life of food.
Film-forming dispersions (FFDs) and films were prepared by using medium molecular weight chitosan (CH, CAS number 9012-76-4) with a deacetylation degree of 75%–85%, supplied by Sigma-Aldrich Química, S.A. (Madrid, Spain). Likewise, oleic acid (CAS number 112-80-1), glacial acetic acid (CAS number 64-19-7) and glycerol (CAS number 56-81-5) were supplied by Panreac Química S.L.U. (Barcelona, Spain), and pure propolis by Bonamel Organic S.L. (Alicante, Spain).
2.2 Preparation of Film-Forming Dispersions
The propolis aqueous extract (PWE) and propolis oleic acid extract (POAE) were prepared as follows: 10 g of crushed pure propolis was extracted with 100 mL of distilled water or OA, respectively, and maintained under magnetic stirring at room temperature in darkness for 4 days. The mixtures were filtered through filter paper (60 g/m2) and the solution was used as PWE or POAE, respectively. The solid content of the extracts was determined gravimetrically in each sample: in the case of the aqueous extract, by means of the drying and weighing of the dried solids of the extract, and in the case of the oily extract, through the difference between the initial mass of propolis and that of the filtered solid, after its washing with petroleum ether and the evaporation of the solvent.
The FFDs were obtained by dissolving 1% wt. of chitosan in distilled water (CHW) or propolis aqueous extract (CHPWE) at room temperature for 4 h, both of which contained glacial acetic acid (0.25% v/v) to favour the chitosan dissolution. Subsequently, 0.5% wt. glycerol with respect to the polymer was added as plasticiser. Other aqueous dispersions were obtained by incorporating 1% wt. of OA (CHW-OA and CHPWE-OA) or POAE (CHPWE-POAE) to the CHW or CHPWE FFDs. All of the FFDs were homogenised in a rotor-stator Ultraturrax (DI25 Yellow Line, IKA®, Staufen, Germany) at 13,500 rpm for 4 min. Afterwards, these were degasified at room temperature using a vacuum pump (MZ 2C NT, Vacuumbrand GMBH+CO KG, Wertheim, Germany).
2.3 Characterisation of the Chitosan Dispersions
The pH of the different dispersions was determined at 25°C, with a pH meter (GLP21+, Crison Instruments, S.A., Barcelona, Spain), in triplicate.
The particle size distribution of the different dispersions was analysed, in triplicate, by light scattering (MasterSizer 2000, Malvern Instruments Ltd., Worcestershire, UK), at 25°C, applying the Mie’s theory. To this end, the dispersions were diluted in distilled water until an obscuration rate of 8%–10%, considering a refractive index of 1.52 and an absorption value of 0.01.
The zeta potential values of the dispersed particles were analysed in triplicate through their electrophoretic mobility, using ZetaSizer equipment (Nano-Z, Malvern Instruments Ltd., Worcestershire, UK). The electrophoretic mobility was converted into zeta potential values using the Smoluchowsky model.
The rheological characterisation of the dispersions was carried out, in triplicate, using a rotational rheometer (HAAKE RheoStress 1, Thermo Fisher Scientific, Surrey, UK) with coaxial cylinders (ISO 3219 Z34DIN sensor system), at 25°C. The flow curves were obtained after 5 min rest of the sample in the coaxial cylinder. The up and down curves were obtained from 0 to 512 s−1 shear rate in 5 min. The consistency index (K) and flow behaviour index (n) were obtained by fitting the power law model (Eq. (1)) to the flow curves.
To obtain the films, the chitosan dispersions (CHw, CHPWE, CHPWE-OA and CHPWE-POAE) were poured on levelled teflon plates (15 cm diameter) and dried at 25°C and 60% relative humidity (RH). The film thickness was controlled by pouring the corresponding amount of the dispersion to obtain a solid surface density of 56 g/m2. The peeled-off, dry films were conditioned at 25°C and 53% RH, in desiccators containing an oversaturated Mg(NO3)2 solution, before their characterisation. A digital micrometer (Electronic Digital Micrometer, Comecta S.A., Barcelona, Spain) was used to measure the film thickness.
The colour and transparency of the films were measured in the conditioned samples. To this end, the infinite reflectance (R∞) spectra and the internal transmittance (Ti) spectra of the films were determined by applying the Kubelka–Munk theory [15], by measuring the film surface reflectance on black and white backgrounds, as described by Pastor et al. [9], using a spectro-colorimeter (CM-3600d, Minolta Co., Tokyo, Japan) with a 10 mm diameter window. CIE-L*a*b* coordinates (D65 illuminant/10° observer), chrome (C*ab) and hue (h*ab) were obtained from of the R∞ spectra of the films and the whiteness index (WI) was determined by applying Eq. (2):
The ASTM Standard D 523-89 [16] was applied to obtain the gloss of the films (60° incidence angle) using a flat surface gloss meter (Multi-Gloss 268, Minolta Co., Tokyo, Japan). The gloss values were expressed as gloss units (GU), relative to a highly polished black glass standard (GU near 100).
Five replicates per sample were carried out for each optical parameter.
The tensile properties of the conditioned films were analysed at 25°C, by using a universal test machine (TA-XTplus, Stable Micro Systems, Surrey, UK) according to ASTM Standard D 882-12 [17] The film samples (25 mm × 100 mm) were mounted in the tensile grips (A/TG model), 50 mm apart, and stretched at 50 mm/min until breaking. The elastic modulus (EM), tensile strength (TS) and percentage of elongation (% E) at break were determined from the stress-strain curves. Twelve replicates were carried out per film sample.
2.5.3 Barrier Properties and Equilibrium Moisture Content
The equilibrium moisture content of the films was estimated by considering the weight of equilibrated films at 53% RH and the corresponding dry solids of the poured FFDs.
The water vapour permeability (WVP) of films was measured by means of the ASTM Standard E 96-95 [18] gravimetric method, using the McHugh et al. modification [19]. Payne permeability cups (Elcometer SPRL, Hermelle/s Argenteau, Belgium) of 3.5 cm in diameter were used and four replicates were carried out for each type of film. The cups were filled with distilled water (100% RH), covered with the fitted films and placed into a desiccator containing oversaturated Mg(NO3)2 (53% RH), at 25°C. The cups were weighed periodically (every 2 h, for 10 h) after the steady state was reached, using an analytical balance (±0.0001 g). The slope of the weight loss vs: time data was used to calculate the water transmission rate, from which the WVP was determined.
The oxygen transmission rate of the films was measured by using the equipment OX-TRAN 1/50, Mocon (Minneapolis, MN, USA), at 25°C and 53% RH [20], in triplicate. The film samples into the test cell were exposed to different oxygen pressure in each side of the film and the oxygen permeability (OP) was calculated from the oxygen transmission rate, considering the oxygen pressure gradient and the average film thickness.
The film’s antioxidant activity was measured in triplicate through the radical scavenging capacity of ABTS [2,2’-azinobis-(3-ethyl-benzothiazoline-6-sulfonic acid)], as described by Re et al. [21]. To this end, ABTS was dissolved in deionised water to a concentration of 7 mm and ABTS.+ was produced by its reaction with potassium persulfate (2.45 mm), throughout 12–16 h in darkness, at room temperature. The ABTS.+ solution was water diluted to an absorbance at 734 nm of ≅ 0.700, measured in a spectrophotometer (Helios Zeta UV-Vis, Thermo Fisher Scientific, Surrey, UK).
Film solutions were obtained from 0.1 g dry film in 10 mL deionised water plus 25 μL glacial acetic acid and maintained under magnetic stirring at room temperature for 12 h in darkness. Then, 1 mL of the film solution was diluted with deionised water up to 5 mL.
The percentage inhibition of the ABTS.+ radical by the film solutions was determined by incorporating 990 μL of diluted ABTS.+ solution into 10 μL of film solution. The absorbance reading at 734 nm was taken immediately after the initial mixing and up to 6 min, when a 20–80% reduction in absorbance was produced. The percentage inhibition with respect to the initial value was calculated and referred to the equivalent concentration of Trolox standard reference (0–2 mm).
2.5.5 Scanning Electron Microscopy (SEM)
The film microstructure was analysed in the conditioned samples at 0% RH, by using a scanning electron microscope (JSM-6300, JEOL Ltd., Tokyo, Japan). The film cross-section was observed after their cryofracture in liquid N2 and gold coating. An accelerating voltage of 10 kV was applied.
A statistical analysis of data was performed through a one-way analysis of variance using Statgraphics® Plus for Windows 5.1. Homogeneous sample groups were obtained by using the LSD test (95% significance level).
3.1 Properties of the Film-Forming Dispersions
The aqueous solutions/dispersions used for film preparation have been characterised, as well as the PWE and POAE (water dispersed at 1% v/w) and 1% (v/w) dispersions of OA in chitosan water solution (CHw-OA), for comparison purposes. Table 1 shows the average values and standard deviations of pH, ζ-potential and rheological parameters of these aqueous systems.
Propolis aqueous extracts exhibited negative values of ζ-potential (−17 mV), as observed by other authors [22], which indicates the presence of negatively ionised compounds such as fatty or phenolic acids (oleic, myristic and palmitic acids or caffeic and ferulic acids), previously found in the propolis aqueous extracts [23]. The ζ-potential of the POAE aqueous dispersion was also negative (−27 mV ± 4 mV) and higher than that previously reported [24] for the 1% v/w OA aqueous dispersion (−3.12 mV), while both exhibited similar pH (5.1–5.2). The negative charge of OA droplets is a result of the ionisation of the carboxylic group (pKa COOH/COO¯ ~ 4.8), which is negatively charged at the pH of the FFD. The greater ζ-potential of the POAE reflected the extraction efficiency of the oleic acid of more negatively ionisable compounds, thus providing the FFD with more negatively charged species. The POAE aqueous dispersion also exhibited a higher charge than the PWE, which may be related with the respective effectiveness and selectivity of each extraction solvent. In fact, the solid content of the water and oily extracts of propolis were 1.2% and 4.6%, respectively. Thus, the extraction efficiency of oleic acid was around 4 times greater than that of water for the propolis compounds, in agreement with the higher content of non-polar compounds of propolis.
The aqueous chitosan dispersion (CHW) showed a positive charge value due to prevalent ammonium groups (pKa NH3+/NH2 ~ 6.5) in the chitosan chains at the pH of the dispersions, the ζ-potential value being similar to that found by other authors [24]. The incorporation of pure OA into the chitosan polymeric solution did not cause significant changes in the ζ-potential with respect to the chitosan solution. In contrast, when chitosan was dissolved in the aqueous propolis extract (CHPWE), the zeta potential significantly decreased (p < 0.05), especially when the samples incorporated oleic acid with and without propolis compounds (CHPWE-POAE and CHPWE-OA). This reflects the neutralising effect of the negatively charged propolis components on the positively charged amino group of chitosan. The presence of OA seems to favour this neutralising effect, which could be explained by the partition of propolis compounds, depending on their affinity, between the aqueous and lipid phases, altering the fraction of ionisable groups available to bond with the ammonium groups of chitosan.
As concerns the rheological properties, the obtained flow curves were fitted to the Ostwald model, and the flow behaviour index (n) and consistency index (K) were obtained. Table 1 shows these values with the determination coefficients (r2), and apparent viscosity at 100 s−1. In terms of rheological behaviour, two sample groups can be differentiated: the samples in which the solvent was water (CHW and CHW-OA), and those in which the solvent was the PWE (CHPWE, CHPWE -OA and CHPWE-POAE). Aqueous chitosan samples, with or without OA (CHw-OA and CHW), exhibited pseudoplastic behaviour with apparent viscosity in the range reported previously for similar systems [24]. In contrast, the presence of propolis components in the aqueous phase promoted a marked decrease in the viscosity while these dispersions exhibited Newtonian behaviour (n~1). This change in the flow behaviour indicates a notable re-arrangement of the polymer molecules due to the interactions with the propolis compounds, also detected in the decrease in the zeta potential values. The less charged chitosan chains would not be as extended/unfolded in the water medium of PWE, exhibiting a lower hydrodynamic volume, milder viscous effect and less sensitivity to unfolding by the shear forces (pseudoplastic behaviour) [25]. The chain charge density also affects the levels of solvent immobilisation and viscosity [26]. Therefore, the incorporation of propolis extracts into chitosan-based FFDs provoked a reduction in the charge density in the polymer chains, leading to a folded and less entangled conformation, thus reducing the solution viscosity.
The particle size distribution curves (Fig. 1) show that the PWE was not a solution but a dispersion with a broad multimodal particle size distribution (0.34–100 μm) and a main peak located around 8 μm. This indicates that some non-water-soluble compounds which were carried over in the aqueous extraction were not removed in the subsequent filtration. The dispersion of OA in aqueous chitosan (CHW-OA) exhibited an almost monomodal particle size distribution with small droplets, ranging between 0.3 and 8.7 μm, and a low ratio of the largest OA droplets, which is attributed to flocs or aggregates. The dissolution of CH in PWE provoked the appearance of bigger particles than those initially present in PWE, which suggests the formation of clusters as a result of the decrease in the zeta potential and, subsequently, in the repulsion forces between the dispersed particles. This effect was more marked when incorporating oleic acid (pure or with propolis compounds) in these aqueous systems, in which there was a remarkable reduction in the number of the finest particles, coherently with the biggest drop in the particle charge.
Figure 1: Particle size distributions of FFDs in terms of the volume percentage of the different particles
Therefore, the propolis compounds obtained by aqueous extraction notably modified the chitosan conformation and charge density of chitosan molecules, giving rise to more folded chains with a milder viscous effect. These changes were promoted when OA was dispersed in the aqueous phase, which could modify the propolis compound partition between the aqueous and lipid phases and, thus, the CH conformation and aggregation of lipid droplets. These changes in the polymer and compound arrangement in the FFDs could affect the film microstructure and physical properties to a different extent.
The incorporation of propolis compounds from the aqueous extract notably affected the microstructure of the films since these affected the polymer conformation and the arrangement of the different components during the film drying. Fig. 2 shows the SEM micrographs of the films’ cross sections (SEM). Pure chitosan films exhibited a homogeneous and smooth surface, as previously described by other authors [10,24,27]. Nevertheless, the presence of propolis compounds from the PWE gave rise to a coarser structure due to the less extended conformation of chitosan in the FFD, which will affect the chain packing in the film as the solvent evaporates. A similar effect was observed for the propolis ethanolic extract incorporated into films based on hydroxypropylmethylcellulose [9]. The incorporation of OA, with and without extracted propolis compounds, into these films promoted greater heterogeneity in the film matrix due to the lack of miscibility of lipids and polymer, giving rise to the presence of dispersed lipid particles in the polymer matrix, in both CHPWE-OA and CHPWE-POAE film formulations.
Figure 2: SEM micrographs of the cross-sections of the dried films conditioned at 0% RH: (a) CHW, (b) CHPWE, (c) CHPWE-OA, (d) CHPWE-POAE
3.2.2 Mechanical Properties of Films
The structural changes and compound interactions promoted by the propolis compounds and OA affected the water binding capacity and the tensile properties of the films. Table 2 shows the equilibrium moisture content of the different films and their values of elastic modulus (EM), and tensile strength (TS) and percentage of elongation (% E) at break, for samples conditioned at 25°C and 53% RH. A sharp reduction in the equilibrium water content of the chitosan films was produced in the presence of water extracted propolis compounds, as was also observed by other authors [10]. This can be attributed to the reduction in the charge density in the chains, commented on above, which weakens the hydration forces of the chains and, so, the water bonding capacity of the films. This will also affect the tensile behaviour of the material, since the hydration layer can weaken the interchain forces in the polymer matrix, whereas the weakening of the interchain repulsion forces promotes chain aggregation leading to a tougher matrix [12,14]. In fact, the elastic modulus of the films in samples containing PWE increased notably compared to that of the pure chitosan films. The latter exhibited lower values than those previously reported for glycerol-free films [24] due to the plasticising effect of this compound, which also helps to reduce the tensile strength at fracture/break. However, the coarse structure of films obtained in PWE produced more brittle films, with a lower degree of extensibility and resistance to break, which could be associated with the less extended conformation of the polymer that makes chain slippage during the film extension more difficult.
The incorporation of oleic acid, containing or not propolis compounds, led to a greater reduction in the water binding capacity of the films, reflecting the greater hydrophobicity of the matrix, and affecting its tensile behaviour. The lack of miscibility of the lipid phase in the polymer give rise to structural discontinuities in the polymer network which provokes the expected reduction in the overall cohesion forces of the matrix [14]. Thus, films with OA or POAE had lower values of elastic modulus and resistance to break and smaller elongation capacity, with no significant effect of the propolis compounds carried by POAE.
Therefore, the water-soluble compounds of propolis greatly affected the water binding capacity and tensile behaviour of chitosan-based films. Oily soluble propolis compounds from POAE, on the other hand, did not have a relevant effect, probably due to their presence in the dispersed phase, with no interactions with the polymer. Other authors also described molecular interactions between the polar components of propolis extracts and the hydrophilic groups of the polymer matrix leading to changes in the mechanical response [10].
3.2.3 Optical Properties of Films
Changes in the film microstructure and the presence of coloured compounds affected the optical properties of the films. Table 3 shows the values of colour coordinates (lightness, chrome and hue), whiteness index and gloss at 60° of the different films. The chitosan films obtained with PWE differed remarkably from those obtained in water; the former was all darker, with more saturated and redder colour, mainly due to the natural colour of the PWE, as observed in previous studies [10,13,14]. Coherently, films obtained in PWE had much lower whiteness index values. The incorporation of OA or POAE did not notably modify the colour parameters of the films.
The internal transmittance (Ti) of the films was also mainly affected by the presence of the propolis water extracted compounds, as shown in Fig. 3. Films obtained with PWE had lower Ti values, below 500 nm, than those films obtained in water, which can be attributed to the selective light absorption of the propolis compounds. The presence of dispersed particles in the films would also contribute to the Ti reduction, depending on their size and the differences in the refractive index. Nevertheless, dispersed OA or POAE phases did not notably affect the Ti values.
Figure 3: Internal transmittance spectra of the different films conditioned at 25°C and 53% RH
The gloss of the films is related to the surface homogeneity achieved after the drying process. The values shown in Table 3 revealed that films obtained in PWE were glossier than those obtained in pure water, whereas the incorporation of OA or POAE reduced the gloss. This suggests that the lipids carried in the PWE (commented on above) could be creamed during the film drying step, forming a thin surface layer that created a more homogeneous and shinier surface on the chitosan films. In contrast, the incorporation of OA or POAE gave rise to a more heterogenous microstructure that will also produce a rougher surface with a lower degree of gloss. During the homogenisation of the FFD, the lipids of the PWE would be incorporated into the lipidic OA dispersed phase that seems stable against creaming, probably due to the emulsifying properties of OA.
3.2.4 Water Vapour and Oxygen Permeability of the Films
The barrier properties (water vapour permeability, WVP, and oxygen permeability, OP) of films conditioned at 25°C and 53% RH are shown in Table 4. As expected, the obtained WVPs of chitosan films were lower than those found for glycerol-free chitosan films by other authors [28]. However, the WVP decreased for films obtained in PWE regardless of the presence of OA or POAE. Therefore, the water soluble propolis compounds were more effective at promoting the water vapour barrier capacity of the chitosan films than the hydrophobic compounds (OA or POAE). This effect is coherent with the reduced water binding capacity of the chitosan films obtained with PWE.
The oxygen barrier capacity also greatly increased in the chitosan films obtained with PWE (85% decrease of OP with respect to the CHW film), despite the apparently greater hydrophobicity of the CHPWE films. This effect can be explained by the higher level of chain aggregation with fewer hydration layers, which reduced the molecular diffusion of the permeants, regardless of their chemical affinity with the matrix. However, a notable (p < 0.05) increase in OP was observed when oleic acid was incorporated (CHPWE-OA), which was even more marked when the POAE extract was added (CHPWE-POAE). In general, the films based on hydrophilic biopolymers have a good oxygen barrier capacity, which decreases when the matrix hydrophobicity rises due to the increase in oxygen solubility in the matrix. The effect of OA and POAE on the OP value of the films could be explained by the high degree of solubility and diffusion of the oxygen in these hydrophobic liquid phases, which accelerates the mass transfer through the matrix. This is intensified with POAE probably due to the presence of highly hydrophobic compounds extracted from propolis, such as waxes, which promote the oxygen solubility.
Therefore, the propolis aqueous extract promoted the water vapour and oxygen barrier capacity of chitosan films as found in previous studies for the ethanolic propolis extracts, where a reduction in WVP of up to 28% and a 62% reduction in OP were reported [12]. Stanicka et al. [12] also found a concentration-dependent effect of the ethanolic propolis extract on the barrier properties of the chitosan films. Taking the solid concentration of the PWE into account, a mass ratio of approximately 1:1 of propolis solids:chitosan was present in the studied samples, which represents a notable concentration of potentially active compounds in the films.
3.2.5 Antioxidant Activity of the Films
Table 4 also shows the antioxidant activity of the different film formulations, expressed as TEAC values. The obtained TEAC values of CHw films were relatively low as reported by Moreno-Vásques et al. [29]. The antioxidant effect of high molecular weight chitosan is different to that exhibited by other typical antioxidants, such as phenolic compounds or vitamins [30]. The free radical scavenging mechanism of chitosan is not clear. Some authors [30,31] have suggested that the unstable free radicals react with the hydroxyl group and/or with the amino group at the pyranose ring carbons (C-2, C-3, and C-6) giving rise to a steady macromolecule.
The chitosan films obtained in PWE exhibited greater antioxidant activity (more than 10 times) than pure chitosan films, thus reflecting the antioxidant action of the propolis phenolic compounds present in the PWE, such as some phenolic acids or flavonoids [10,14]. The radical scavenging capacity of phenols is based on the H donor capacity of the hydroxylic phenol, reacting with the free radical and giving rise to steady aromatic radicals. The ABTS•+ radical cation-based assay is one of the most frequently used antioxidant capacity assays. Nevertheless, the complexity of the reaction pathways in the ABTS/potassium persulfate decolourisation assay makes the comparison of the results obtained in different studies difficult. Even for some standard antioxidants, such as quercetin, naringin, or glutathione, different laboratories may be observed to give varying TEAC values [32]. As described by Ilyasov et al. [32], there are two principal reaction pathways for phenolic compounds (scheme in Fig. 4).
Figure 4: Scheme of the mechanism reactions of phenolic antioxidants (A–H) and the ABTS.+ radical (adapted from Ilyasov et al. [32])
Some phenols may form coupling adducts with ABTS•+ that can suffer subsequent oxidation, whereas others can undergo oxidation through a previous dissociation of the acidic phenol. The coupling of specific reactions depends on the antioxidant compound. This gives rise to controversies in the translation of the results of colorimetric antioxidant tests to a physiological context. Additionally, the fact that the radical trapping rate by antioxidant compounds is unknown represents a problem. The difficulty of predicting chemistry leads to complex kinetics and stoichiometries, and the subsequent uncertainty of results and their interpretation [32]. Nevertheless, the assay carried out in the most standard conditions (6 min reaction time) revealed a notable enhancement in the film antioxidant capacity when the water extracted compounds from propolis were incorporated, coherently with the radical scavenging ability of the constitutive phenolics. In contrast, the oleic acid or POAE addition led to no improvement in the antioxidant activity of CHPWE films, suggesting that the compounds extracted by OA did not have a notable antioxidant capacity.
The use of propolis aqueous extract to obtain chitosan films gave rise to significant changes in the physicochemical properties of the film-forming dispersions and films, mainly due to the reduction of the polymer charge density, associated with the interactions with the negatively ionisable propolis compounds. Likewise, the incorporation of oleic acid, with and without extracted propolis compounds, also provoked changes in both film-forming dispersions and films. Aqueous extracted propolis compounds produced a coarser film microstructure, which exhibited greater stiffness and less stretchability and milder resistance to break, but an improved barrier capacity to water vapour and oxygen, and 10 times more antioxidant capacity. The films also became darker, redder and glossier, with less transparency at low wavelengths. When OA, containing or not propolis compounds, was incorporated into the chitosan films with aqueous propolis extract, the expected decrease in both the film stiffness and resistance to break was observed without there being any notable changes in the water vapour barrier capacity or antioxidant activity. However, OA greatly boosted oxygen permeability, mainly when carrying propolis compounds. Therefore, chitosan films with aqueous propolis extract, without oleic acid, have the greatest potential to preserve food from oxidative spoilage on the basis of their great oxygen barrier capacity and antioxidant activity and exhibit the best properties for application as food packaging materials. Further studies are required to validate this possible application.
Acknowledgement: None.
Funding Statement: This work was supported by Generalitat Valenciana (Valencia, Spain) (Grant No. CIPROM/2021/071).
Author Contributions: Amparo Chiralt, Chelo González-Martínez and Maite Cháfer conceived and designed the experiments, analyzed and interpreted the results and reviewed the paper. Clara Pastor performed the experiment and wrote the paper. Amparo Chiralt acquired funding for this work. All authors reviewed the results and approved the final version of the manuscript.
Availability of Data and Materials: Data is available upon request.
Ethics Approval: Not applicable.
Conflicts of Interest: The authors declare no conflicts of interest to report regarding the present study.
References
1. Anjum SI, Ullah A, Khan KA, Attaullah M, Khan H, Ali H, et al. Composition and functional properties of propolis (bee glue): a review. Saudi J Biol Sci. 2019;26(7):1695–703. doi:10.1016/j.sjbs.2018.08.013. [Google Scholar] [PubMed] [CrossRef]
2. Keskin M, Keskin S, Kolayli S. Preparation of alcohol free propolis-alginate microcapsules, characterization and release property. LWT. 2019;108(2):89–96. doi:10.1016/j.lwt.2019.03.036. [Google Scholar] [CrossRef]
3. Stojanovic S, Najman SJ, Bogdanova-Popov B, Najman SS. Propolis: chemical composition, biological and pharmacological activity–a review. Acta Medica Medianae. 2020;59(2):108–13. doi:10.5633/amm.2020.0215. [Google Scholar] [CrossRef]
4. Rebiai A, Seghir BB, Hemmami H, Zeghoud S, Belfar ML, Kouadri I. Determination of some phenolic acids in Algerian propolis. Ovidius Univ Annals of Chem. 2021;32:120–4. [Google Scholar]
5. Costa AG Jr, Yoshida Nídia C, Garcez WS, Perdomo RT, Matos Mde Fátima C, Garcez FR. Metabolomics approach expands the classification of propolis samples from midwest Brazil. J Nat Prod. 2020;83(2):333–43. doi:10.1021/acs.jnatprod.9b00783. [Google Scholar] [PubMed] [CrossRef]
6. Asem N, Abdul Gapar NA, Abd Hapit NH, Omar EA. Correlation between total phenolic and flavonoid contents with antioxidant activity of Malaysian stingless bee propolis extract. J Apic Res. 2019;59(4):437–42. doi:10.1080/00218839.2019.1684050. [Google Scholar] [CrossRef]
7. Ecem Bayram N, Gerçek YC, Bayram S, Togar B. Effects of processing methods and extraction solvents on the chemical content and bioactive properties of propolis. J Food Meas Charact. 2020;14(2):905–16. doi:10.1007/s11694-019-00340-z. [Google Scholar] [CrossRef]
8. Juodeikaitė D, Žilius M, Briedis V. Preparation of aqueous propolis extracts applying microwave-assisted extraction. Processes. 2022;10(7):1330. doi:10.3390/pr10071330. [Google Scholar] [CrossRef]
9. Pastor C, Sánchez-González L, Cháfer M, Chiralt A, González-Martínez C. Physical and antifungal properties of hydroxypropylmethylcellulose based films containing propolis as affected by moisture content. Carbohydr Polym. 2010;82(4):1174–83. doi:10.1016/j.carbpol.2010.06.051. [Google Scholar] [CrossRef]
10. de Carli C, Aylanc V, Mouffok KM, Santamaria-Echart A, Barreiro F, Tomás A, et al. Production of chitosan-based biodegradable active films using bio-waste enriched with polyphenol propolis extract envisaging food packaging applications. Int J Biol Macromol. 2022;213:486–97. doi:10.1016/j.ijbiomac.2022.05.155. [Google Scholar] [PubMed] [CrossRef]
11. Segueni N, Boutaghane N, Asma ST, Tas N, Acaroz U, Arslan-Acaroz D, et al. Review on propolis applications in food preservation and active packaging. Plants. 2023;12(8):1654. doi:10.3390/plants12081654. [Google Scholar] [PubMed] [CrossRef]
12. Stanicka K, Dobrucka R, Woźniak M, Sip A, Majka J, Kozak W, et al. The effect of chitosan type on biological and physicochemical properties of films with propolis extract. Polymers. 2021;13:3888. doi:10.3390/polym13223888. [Google Scholar] [PubMed] [CrossRef]
13. Isfran D, Caicedo Chacon W, Santos J, Monteiro A, Ayala Valencia G. Active films and coatings based on propolis extract and chitosan: physicochemical characterization and potential application in refrigerated shrimps (Litopenaeus vannamei). Starch‐Stärke. 2023;75:2200263. doi:10.1002/star.202200263. [Google Scholar] [CrossRef]
14. Stefanowska K, Wozniak M, Sip A, Mrówczynska L, Majka J, Kozak W, et al. Characteristics of chitosan films with the bioactive substances-caffeine and propolis. J Funct Biomater. 2023;14:358. [Google Scholar] [PubMed]
15. Hutchings JB. Food color and appearance. 2nd ed. Gaithersburg, Maryland, USA: Aspen Publishers Inc.; 1999. [Google Scholar]
16. ASTM. Standard test method for specular gloss. ASTM D523-14(2018). In: Annual book of ASTM standards. West Conshohocken, PA, USA: ASTM International; 1999. vol. 06.01, p. 1–5. doi:10.1520/D0523-14R18. [Google Scholar] [CrossRef]
17. ASTM. Standard test method for tensile properties of thin plastic sheeting. ASTM D882-18. In: Annual book of ASTM standards. West Conshohocken, PA, USA: ASTM International; 2012. vol. 08.01, p. 1–12. doi:10.1520/D0882-18. [Google Scholar] [CrossRef]
18. ASTM. Standard test methods for water vapor transmission of materials. ASTM E96-00. In: Annual book of ASTM standards. West Conshohocken, PA, USA: ASTM International; 1995. vol. 04.06, p. 1–8. doi:10.1520/E0096-00. [Google Scholar] [CrossRef]
19. McHugh TH, Avena-Bustillos R, Krochta JM. Hydrophilic edible films: modified procedure for water vapor permeability and explanation of thickness effects. J Food Sci. 1993;58:899–903. [Google Scholar]
20. ASTM. Standard test method for oxygen gas transmission rate through plastic film and sheeting using a Coulometric sensor. ASTM D3985-24. In: Annual book of ASTM standards. West Conshohocken, PA, USA: ASTM International; 2005. vol. 15.10, p. 1–7. doi:10.1520/D3985-24. [Google Scholar] [CrossRef]
21. Re R, Pellegrini N, Proteggente A, Pannala A, Yang M, Rice-Evans C. Antioxidant activity applying an improved ABTS radical cation decoloration assay. Free Radical Biol Med. 1999;26:1231–7. doi:10.1016/S0891-5849(98)00315-3. [Google Scholar] [PubMed] [CrossRef]
22. Aytekin AA, Tuncay Tanrıverdi S, Aydın Köse F, Kart D, Eroğlu İ., Özer Ö. Propolis loaded liposomes: evaluation of antimicrobial and antioxidant activities. J Liposome Res. 2020;30(2):107–16. doi:10.1080/08982104.2019.1599012. [Google Scholar] [PubMed] [CrossRef]
23. Al-Juhaimi FY, Özcan MM, Mohamed Ahmed IA, Alsawmahia ON, Özcan MM, Ghafoor K, et al. Bioactive compounds, antioxidant activity, fatty acid composition, and antimicrobial activity of propolis from different locations in Turkey. J Apic Res. 2022;61(2):246–54. doi:10.1080/00218839.2021.1898785. [Google Scholar] [CrossRef]
24. Vargas M, Albors A, Chiralt A, González-Martinez C. Characterization of chitosan-oleic acid composite films. Food Hydrocolloids. 2009;23:536–47. doi:10.1016/j.foodhyd.2008.02.009. [Google Scholar] [CrossRef]
25. Costa CN, Teixeira VG, Delpech MC, Souza JVS, Marcos AS, Costa MAS. Viscometric study of chitosan solutions in acetic acid/sodium acetate and acetic acid/sodium chloride. Carbohydr Polym. 2015;133:245–50. doi:10.1016/j.carbpol.2015.06.094. [Google Scholar] [PubMed] [CrossRef]
26. Cho J, Heuzey MC, Begin A, Carreau PJ. Viscoelastic properties of chitosan solutions: effect of concentration and ionic strength. J Food Eng. 2006;74:500–15. doi:10.1016/j.jfoodeng.2005.01.047. [Google Scholar] [CrossRef]
27. Flores Z, San Martín D, Beldarraín-Iznaga T, Leiva-Vega J, Villalobos-Carvajal R. Effect of homogenization method and carvacrol content on microstructural and physical properties of chitosan-based films. Foods. 2021;10(1):141. doi:10.3390/foods10010141. [Google Scholar] [PubMed] [CrossRef]
28. Rivero S, Damonte L, García MA, Pinotti A. An insight into the role of glycerol in chitosan films. Food Biophys. 2016;11(2):117–27. doi:10.1007/s11483-015-9421-4. [Google Scholar] [CrossRef]
29. Moreno-Vásquez MJ, Plascencia-Jatomea M, Sánchez-Valdes S, Tanori-Córdova JC, Castillo-Yañez FJ, Quintero-Reyes IE, et al. Characterization of epigallocatechin-gallate-grafted chitosan nanoparticles and evaluation of their antibacterial and antioxidant potential. Polymers. 2021;13(9):1375. doi:10.3390/polym13091375. [Google Scholar] [PubMed] [CrossRef]
30. Riaz Rajoka MS, Zhao L, Mehwish HM, Wu Y, Mahmood S. Chitosan and its derivatives: synthesis, biotechnological applications, and future challenges. Appl Microbiol Biotechnol. 2019;103(4):1557–71. doi:10.1007/s00253-018-9550-z. [Google Scholar] [PubMed] [CrossRef]
31. Muthu M, Gopal J, Chun S, Devadoss AJP, Hasan N, Sivanesan I. Crustacean waste-derived chitosan: antioxidant properties and future perspective. Antioxidants. 2021;10(2):228. doi:10.3390/antiox10020228. [Google Scholar] [PubMed] [CrossRef]
32. Ilyasov IR, Beloborodov VL, Selivanova IA, Terekhov RP. ABTS/PP decolorization assay of antioxidant capacity reaction pathways. Int J Mol Sci. 2020;21(3):1131. doi:10.3390/ijms21031131. [Google Scholar] [PubMed] [CrossRef]
Cite This Article
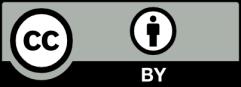
This work is licensed under a Creative Commons Attribution 4.0 International License , which permits unrestricted use, distribution, and reproduction in any medium, provided the original work is properly cited.