Open Access
ARTICLE
Termite Resistance of Heat-Treated Eucalyptus OSB Bonded with Eco-Friendly Castor Oil Adhesive
1 SerQ, Innovation and Competence Forest Center, Sertã, 6100-711, Portugal
2 Materials and Technology Department, Faculty of Engineering and Sciences of Guaratinguetá, São Paulo State University (UNESP), Guaratinguetá, 12516-410, Brazil
3 Department of Civil Engineering, University of Coimbra, Coimbra, 3030-790, Portugal
4 Exact Sciences and Technology Center, Federal University of São Carlos, São Carlos, 13565-905, Brazil
5 Department of Forest and Wood Science, Federal University of Espírito Santo, Jerônimo Monteiro, 29550-000, Brazil
* Corresponding Author: Estefani S. Sugahara. Email:
(This article belongs to the Special Issue: Advances in Eco-friendly Wood-Based Composites: Design, Manufacturing, Properties and Applications – Ⅱ)
Journal of Renewable Materials 2024, 12(11), 1911-1925. https://doi.org/10.32604/jrm.2024.056198
Received 16 July 2024; Accepted 23 September 2024; Issue published 22 November 2024
Abstract
Over the last decade, the oriented strand board (OSB) market presented meaningful growth. However, as a wood-based product, because of its anatomical structure and chemical composition, OSB can be damaged by biodeterioration agents. Given that, the biodeterioration of OSB panels must be investigated to improve its durability. In this way, this work analyses the biological resistance against termites (Cryptotermes brevis and Nasutitermes corniger) of heat-treated OSB panels made with Eucalyptus wood glued with vegetable-based polyurethane-an eco-friendly and sustainable adhesive derived from castor oil. Various panels were produced with different layers compositions (face:core:face of 25:50:25 and 30:40:30) in wood mass proportion and were submitted to postproduction heat treatment (at 175°C and 200°C) replacing the use of chemical insecticides. The influence of the layers variation and heat treatment temperature were evaluated, and these results were compared with commercial panels (made from pinus wood with insecticide). The results showed that the heat treatment did not improve the resistance against termite attack. However, all the experimental panels presented a satisfactory performance that was compatible with the commercial panels produced with insecticide available in the Brazilian market. The combination of Eucalyptus wood and castor oil adhesive to produce OSB, in any variation of layer composition, demonstrated natural resistance against termite attack compatible with the commercial panels, even without using chemical additives to increase durability.Graphic Abstract
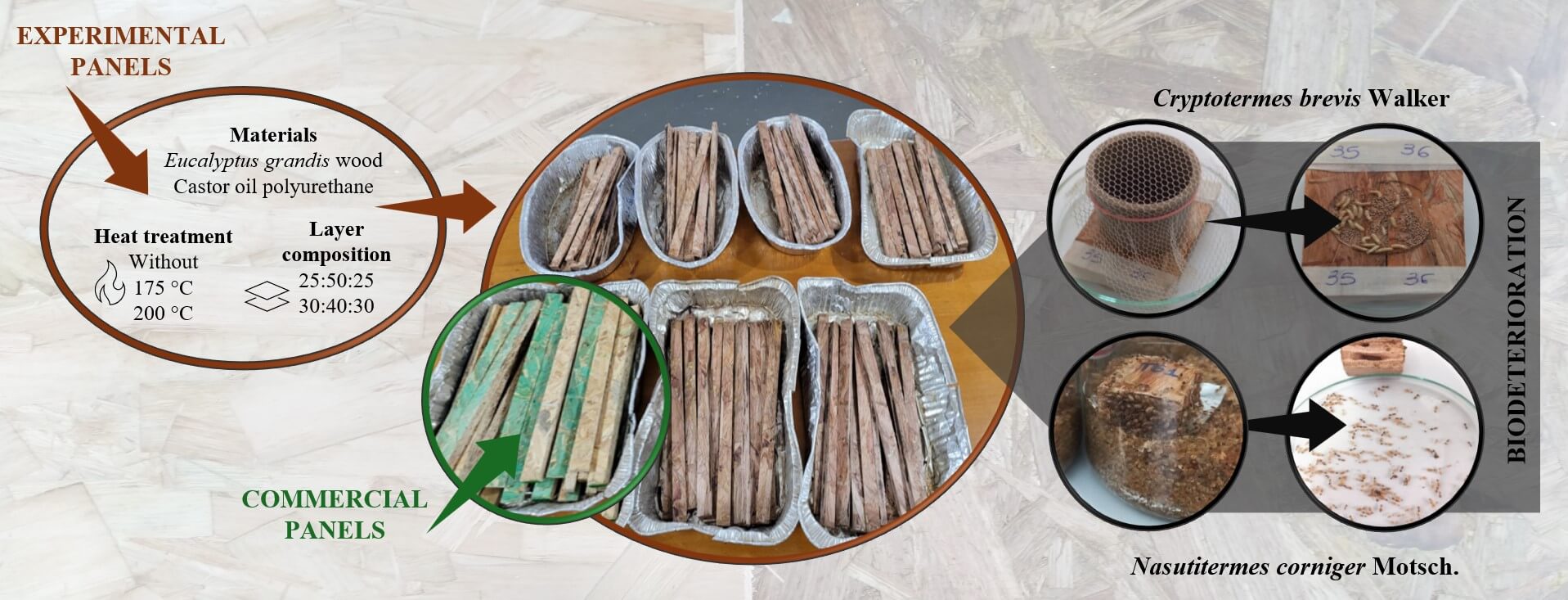
Keywords
The building sector has a substantial social and economic impact; however, it can also be responsible for important negative impacts concerning the environment. Thus, to improve these possible negative impacts, sustainability strategies must be adopted by the industry [1]. The sustainable progress of the construction industry is conditioned by the environmental profile of the materials, requiring the development of innovative sustainable materials that properly can be an effective alternative to traditional materials [2]. With the expanding interest in environmental questions, producing lignocellulosic-based composites has conquered extensive attention, and made satisfactory progress in the construction materials sector [3]. Thus, the use of bio-based materials can contribute to decreasing the environmental impact and achieving sustainable development [4].
Wood has been employed for construction for thousands of years and remains an important construction material [5] favoring many aspects of human life as a versatile natural resource, not only used as a construction material but also applied in a wide range of applications [6] in several industrial sectors [7]. Thus, wood products play a fundamental role in the circular economy, offering a renewable source of materials with a commonly lower carbon footprint [8].
One of its principal applications is to produce oriented strand board (OSB), which is a panel composed of wood strands bonded with an adhesive frequently used for structural purposes in the civil construction and furniture industry [9,10].
Over the last decade, the OSB market presented meaningful growth [9] with an increase in worldwide consumption [11]. The Brazilian wood panel market, in which the OSB panels are inserted, has also been rising, but in Brazil, the OSB panels are still being only produced with pine wood [12]. Thus, to avoid a lack of wood supply can be a critical issue in the future, researching alternative lignocellulosic raw materials is essential [13] to produce quality products that meet usage requirements [14], when compared to other products, however, with a more sustainable production [15].
Because of its anatomical structure and chemical composition, wood can be damaged by biodeterioration agents, such as coleopteran, termites, fungi, and marine borers [7]. However, if treated with proper techniques to resist decay, wood can last hundreds of years [16]. Given that, wood biodeterioration processes must be investigated to apply the material correctly and reduce costs and waste generation caused by the substitution of deteriorated materials [7].
The xylophagous organisms like subterranean, arboreal, or soil termites can invade urban centers and deteriorate any objects fabricated with wood or wood products causing irreversible deterioration. Among these, individuals of the Nasutitermes (arboreal or soil termites) and Cryptotermes (drywood termites), for example, can be mentioned as principal sources of wood losses [17] causing problems to wood in service with significant economic importance [18] and also affecting the structure, possibly exposing people to risks [17]. In this sense, it is imperative to apply preservative treatments to protect wood from these agents.
In the chemical modification of wood, a reaction occurs between the wood constituents (lignin, hemicelluloses, and cellulose) and the preservatives, forming a stable covalent bond between the preservative and the cell wall [19]. But nowadays there are rising concerns involving the harmful potential of traditional chemical wood preservatives [18]. Thus, the study of alternative treatment methods is important both to reduce costs and impacts on the environment, since reducing damage to the ecosystem and health is part of the interests of the wood treatment industry [7]. Therefore, heat treatments can be applied as they can add qualities to wooden products to meet market demands [20].
The heat treatment is a wood modification method that applies temperature to cause a managed wood degradation, in order to refine some of its properties [21] reducing the thickness swelling and making it less hygroscopic [22]. In this way, the heat treatment of lignocellulosic materials proves to be an alternative to improve biological resistance to fungi and termites, and the dimensional stability, however, can affect the mechanical properties [14,23,24].
Even though the development of the interest of the scientific community in wood modification, heat treatments still present far fewer studies compared to other techniques. Likewise, it turns out that, despite the number of publications about OSB panels, there is still a minor number of studies published aiming to evaluate the effects of heat treatment on it [23]. In an analogous way is noted that although wood boards are promising products for the furniture and building industries, there is still little information about their resistance to attacks by xylophagous deterioration organisms [17].
Wood products will not achieve their greatest potential use until they are fully described and the processes that control their performance properties are entirely understood so that it is possible to manipulate these properties to achieve the desired optimal performance [16]. Regarding wood panels, the susceptibility to termite attack is determined by the quality of the panel. This quality is affected by the attributes related to the materials used (wood, adhesive, and additives) like particle moisture content, geometry, and distribution [25], and other factors related to the process, such as temperature, time, types of organisms to which are exposed, wood species, and use conditions [26].
Termite tests are usually evaluated considering mass loss, a visual rating scale for the intensity of damage, and mortality. Mass loss is the simpler evaluation method since it is based on measuring the weight samples at a constant moisture content before and after exposure. The degree of attack is visually assessed by the human eye which can evaluate degrees of damage on materials, especially at low and high levels. Finally, termite mortality provides a simple evaluation of termite survival. Some mortality is normally expected in a termite test. However, the mortality must be smaller with untreated controls [27].
In this way, to fill knowledge gaps in the area related to the evaluation of biodeterioration of wood panels, studies were carried out associated with particleboards [14,17,25,28,29], oriented structural straw board (OSSB) [30], solid wood panels [26], thermally compressed wood panels [26], plywood [31–33], hybrid sandwich panels [34], and bamboo-based panels [35].
Particularly to OSB panels, the study of Ferro et al. [36] assessed the termite resistance of OSB manufactured with paricá (Schizolobium amazonicum) wood and chromated copper arsenate (CCA) and chromated copper borate (CCB) preservatives. Cheng et al. [37] evaluated the termite resistance of OSB panels made with soy flour as a partial substitute for the resin methylene diphenyl diisocyanate (pMDI). Lee et al. [38] studied the mechanical properties and termite and decay resistance performance of wood-based panels used in wooden houses (OSB, structural particleboard, and particleboard). Tascioglu et al. [39] researched the biological decay and termite resistance of five types of commercially available wood-based composites (softwood and hardwood plywood, medium density fiberboard, OSB, and particle board post-treated with alkaline copper quaternary (ACQ) and copper azole (CA).
However, the resistance against termite attack in OSB panels made by eucalyptus wood and adhesive derived from castor oil and heat treated replacing the use of chemical insecticides has not yet been analyzed by any previous study.
Another important concern is related to the use of formaldehyde-based adhesives in wood products, which is a colorless gas that can possibly originate protein denaturation and may cause cancer [4]. Thus, one of the alternatives to avoid resin toxicity is the employment of formaldehyde-free adhesives [40]. Consequently, formaldehyde-free bio-adhesives derived from renewable materials are receiving increasing interest from industry and researchers due to strict emission regulations added to consumer preference for healthier and sustainable products [41].
Researching innovative solutions that are environmentally cleaner than the existing ones has become crucial [42]. In this sense, aiming to find alternatives that can overcome these possible environmental and health problems, previous studies were carried out where OSB panels made with Eucalyptus wood and adhesive derived from castor oil, subjected to postproduction heat treatment replacing the use of wood chemical preservatives already presented technical viability to be used as a structural construction material, since demonstrated physical and mechanical properties [43] compatible with requirements of the European Standard-EN 300 [44].
However, only the evaluation of the mechanical properties is not sufficient to classify the employability of bio-based products. It is required to carry out studies that assess the effects of the attack by woody organisms in diagnosing the quality of materials [17]. In line with this, the recently published Brazilian Standard-NBR 16936-Light Wood Frame Buildings, Brazilian Association of Technical Standards–ABNT [45], determines the minimum requirements for OSB panels, detailing not only values for the physical and mechanical properties, but also for the resistance properties against termite attack and decaying fungi, based on the requirements of the American Wood Protection Association-AWPA E1-22 and AWPA E30-22 [46–47] standards, respectively.
Cryptotermes brevis is one of the most common drywood termites in Brazil. It can cause great economic losses due to its geographic distribution and its feeding preference. It attacks manufactured wooden objects and parts but does not infest living trees. This species lives inside the wood on which it feeds and where it forms colonies [48].
Subterranean termites of the species Nasutitermes corniger develop their colonies and nests in or near the soil. They build tunnels in the ground and around obstacles until they reach the wood, which is used for feeding. The survival of these termites depends on humidity, with which they must always be in contact, whether from the attacked wood or from the soil in which they live. They do not establish themselves in buildings isolated from contact with the soil, nor are they transported inside furniture, like drywood termites. They attack the wood by accessing it through the soil, maintaining their colonies in places outside the attack site [48].
However, despite the differences between them, both species (Cryptotermes and Nasutitermes) attack furniture and wooden structures in both rural and urban environments [18], leading to the weakening of the wood and making the affected areas susceptible to collapse [25].
Considering the potential growth of the sector and the relative scarcity of studies in the area, it is necessary to investigate the effectiveness of different methods of both OSB production and treatment, to validate alternatives that could upgrade productivity, increase possibilities of raw materials supply, and also reduce environmental concerns providing evidence to support decision-makers about the behavior, feasibility, durability, and safety of these materials. Thus, this study investigates the biological resistance to termites (Cryptotermes brevis and Nasutitermes corniger) of heat-treated OSB panels made with Eucalyptus wood glued with vegetal polyurethane, an eco-friendly and sustainable adhesive derived from castor oil.
2.1 Production and Heat Treatment of OSB Panels
The panels were produced and heat-treated following the procedures adopted by Sugahara et al. [43] with dimensions of 42 cm × 42 cm × 1.2 cm (length × width × thickness), the nominal density of 0.78 g cm−3, using 1500 g of Eucalyptus grandis wood particles, from 7 years-old trees (from a planted forest in the southwest region of the state of São Paulo, Brazil, donated by the company Vale do Cedro®, Ribeirão Branco, São Paulo, Brazil), without application of preservative treatment, and glued with bicomponent polyurethane adhesive derived from castor oil (AGT 1315, donated by the company Imperveg®, Aguaí, São Paulo, Brazil) in a proportion of 10% of adhesive based on the dry mass of the particles in a 1:1 ratio between polyol and pre-polymer.
The experimental panels were manufactured with three layers, varying the proportions of particle mass between the face:core:face layers in the compositions of 25:50:25 and 30:40:30 (Table 1), were also evaluated generic commercial panels made in Brazil and purchased in a construction material store, which uses chemical preservatives (insecticide against termite attack) in its production.
The pressing (Hidral-Mac PHH 80T, Araraquara, Brazil) was carried out at a temperature of 120°C, a pressure of 4.4 MPa in two cycles of 300 s of pressing with 30 s of pressure relief, as shown in Fig. 1.
Figure 1: Pressing program
The heat treatment was carried out in a conventional oven (Marconi MA 035/3BX, Piracicaba, Brazil), without replacing the atmosphere, where the panels were inserted with the oven still at room temperature (25°C–30°C), and after reaching the temperature defined for each treatment (175°C and 200°C) they were maintained for 60 min for heat treatment and after the oven was turned off (Fig. 2), the door was slightly opened and the panels were removed from the oven only after it returns to room temperature (considering room temperature between 25°C and 30°C).
Figure 2: Thermal modification program
2.2 Drywood Termite Resistance Test (No-Choice Feeding Test)
The test was carried out in an adapted manner based on the procedures defined by the method of the Institute of Technological Research of the State of São Paulo-IPT/DIMAD D-2 [49]. Which is similar to that described by Maistrello [50], except for the number of termites and exposure time. Thus, the termites were collected from school furniture attacked pieces in the municipality of Alegre and Jerônimo Monteiro, State of Espírito Santo, Brazil. A total of 10 samples were used per treatment, with dimensions 2.3 cm × 7.0 cm × 1.2 cm (width × length × thickness).
The mass of the samples was measured after drying in an oven at 60°C ± 2°C, until constant mass. The samples (Fig. 3) were prepared in Petri dishes joined in pairs using adhesive tape at the ends and above each pair, and a polyvinyl chloride (PVC) container measuring 3.5 cm × 4.0 cm (diameter × height) was connected with paraffin.
Figure 3: Forced feeding test with drywood termites
Note: (a) Complete apparatus at the beginning of the test. (b) Top view of the sample after disassembling the apparatus. (c) Side view of the sample during termite counting. (d) Sample after counting termites.
In an equivalent way to that used by Gonçalves et al. [14] and Paes et al. [17], 40 termites (39 workers and one soldier) of the species Cryptotermes brevis Walker were inserted into each container. The top of the tubes was covered with “tulle” type fabric to prevent termites from being eaten by predators, such as ants, spiders, and wall geckos. The test was maintained for 45 days in an air-conditioned room at a temperature of 28°C ± 2°C and relative humidity of 65% ± 5%. After that, the samples were exposed, in an oven, under the same cited conditions, and obtained the mass loss.
The quantity of termites given by each standard differs. However, it is important to use an adequate amount compatible with the samples (exhibition area). The presence of soldiers helps guarantee an intensity of attack analogous to that observed in a colony. At the same time, the number of workers becomes important too since few workers will be incapable of holding off the social structure becoming vulnerable to competing microorganisms, while too many termites can overburden the resources and generate stresses that can change the feeding behavior [27].
Were considered in the assessment of the loss of mass, termite mortality, and damage caused by termites where scores were adopted for visual assessments, being 0 (no damage); 1 (surface damage); 2 (moderate damage); 3 (accentuated damage); and 4 (deep damage).
2.3 Subterranean (Arboreal) Termite Resistance Test (No-Choice Feeding Test)
Choice feeding tests closely replicate natural conditions being more appropriate for evaluating feeding preferences or resistance to harmful substances than no-choice tests. Nevertheless, it is problematic to conclude if the mortality in a no-choice test is caused by nontoxic resistance factors or due to chemical toxicity. In this way, no-choice tests are fundamentally hunger tests, so interferences in feeding result in starvation and high mortality, whether samples are toxic or not [27].
In that way, no-choice feeding test with subterranean termites was carried out following the procedure proposed by AWPA E1-22 [46] with conehead termites of the species Nasutitermes corniger Motsch., collected in the rural area of the municipality of Jerônimo Monteiro, Espírito Santo, Brazil. Ten samples were used per treatment with dimensions of 2.5 cm × 2.5 cm × 1.2 cm (width × length × thickness), dried in an oven at 60°C ± 2°C until reaching constant mass.
The samples (Fig. 4) were placed in glass containers with a capacity of 600 mL, protected from direct contact with sand by applying an aluminum foil with dimensions slightly larger than those presented by the samples. The sand was previously washed, sieved, sterilized in an oven at 130°C, and moistened with distilled water according to its water retention capacity in an analogous way used by Paes et al. [17].
Figure 4: No-choice test with subterranean (arboreal) termites
Note: (a) Side view of the container with the sample. (b) Top view of the container with the sample. (c) Sample before termite counting. (d) Sample after counting termites.
Approximately 400 individuals (termites) were inserted into the containers (1.32 ± 0.05 g), in the proportion existing in the colony (12% soldiers and 88% workers). The test was carried out in an air-conditioned room at 28°C ± 2°C with a relative humidity of 65% ± 5% for 28 days. Mass loss, termite mortality, and visual damage caused by termites to specimens were evaluated by visual rating scale. For damage, grades were assigned to the specimens, being: 10 (Sound, surface nibbles permitted), 9 (Light attack), 7 (Moderate attack, with penetration), 4 (Heavy attack), and 0 (Failure). For mortality, the indices were considered Slight (0%–33%), Moderate (34%–66%), Heavy (67%–99%) and Complete (Total, 100%), as in AWPA E1-22 [46].
A completely randomized design, with a factorial arrangement, consisting of 10 replications per treatment (three temperatures × two compositions plus one positive control) was used to analyze the results from the no-choice feeding test of drywood and subterranean (arboreal) termites. Tukey’s mean contrast test (p < 0.05) was used to check differences in the mean values of the properties evaluated considering the seven treatments investigated. This approach makes it possible to compare the results of manufactured panels while also considering the results of commercial panels.
The Anderson-Darling test (AD; p < 0.05) and the multiple comparison test (MC; p < 0.05) were used to verify the normality and homogeneity of variances between treatments, in order to validate (p-value < 0.05) the results of the Tukey test as well as the results of the analysis of variance (ANOVA, F test p < 0.05). The Johnson transformation (change of scale) was used in cases where normality in the distribution of ANOVA residuals was not detected.
ANOVA (full factorial design 23–6 treatments) was also used to evaluate the influence (p-value < 0.05) of temperature (T), layer composition (Comp) as well as interaction effects (T × Comp) between these two factors in the three properties considered (loss of mass, damage, and mortality).
The results of the analysis of variance were presented using the Pareto chart, the effects of each isolated factor, when significant, were presented graphically using main effects graphs, and the interaction effects were presented using interaction graphs between factors. This approach, in which commercial panels are not considered, makes it possible to identify the influence of factors in isolation as well as the interaction between them.
3.1 Dry Wood Termite Resistance Test
Regarding loss mass (Fig. 5a), a significant difference was found only between T2 (highest mean), and T4 (lowest mean), and the others did not show a significant difference between them. Therefore, the mass loss of all treatments was less than 1%, which according to Gonçalves et al. [14] is considered low.
Figure 5: Summary of results: no-choose feeding test with drywood termites
Note: (a) Loss of mass. (b) Damage (Scores). (c) Mortality. Equal letters imply different treatments associated with statistically equivalent means using the Tukey mean contrast test (p < 0.05). Tr = Treatments.
About the visual damage score (Fig. 5b), T1, T2, and T4 can be classified as 1 (surface damage) while T3, T5, T6, and T7 as 2 (moderate damage). However, there was no statistically significant difference between the damage of all treatments evaluated, as well as the mortality values (Fig. 5c). The p-values of the normality test and homogeneity of variances were higher than the significance level (p < 0.05) in all responses, which validates the results of the Tukey test (p < 0.05).
Such results indicate that the experimental panels, with (T3, T4, T5, and T6) and without heat treatment (T1 and T2) achieve damage and mortality properties with no significant difference to that obtained by the commercial panels (T7), which usually have chemical preservatives in their composition to improve its resistance to biological attack.
In this sense, it is possible to infer that the heat treatment does not improve the resistance against termite damage and mortality. However, it can be assumed that, even without using a preservative method against termites, the experimental panels presented performance compatible with the commercial ones. Indicating that this result is related to the sum of the characteristics presented in the combination of wood (Eucalyptus) and adhesive (polyurethane derived from castor oil) used in the specific form of production adopted.
This result is in line with the study of Ferro et al. [36] that evaluated the termite resistance of OSB manufactured with paricá (Schizolobium amazonicum) wood glued with castor oil-based resin treated with CCA and CCB preservatives. In their work CCB treated and untreated panels did not present significantly different means for termite mortality. Thus, they concluded by saying that OSB manufactured with untreated particles and CCB treated together with castor oil adhesive presented the same resistance against termite attack.
The AWPA E1-22 [46] defines the criteria for the approximate termite mortality as slight (0% to 33%), moderate (34% to 66%), heavy (67% to 99%), and complete (100%). Thus, adopting this classification to the drywood termites’ results obtained, it is verified that all treatments evaluated presented moderate termite mortality (40% to 63.5%).
According to Rocha Medeiros [51], heat treatment of Eucalyptus clones did not affect drywood termite attack in the no-choice feeding test. Conversely, according to Paes et al. [17] the temperature and pressing time used to consolidate the boards might influence the results because these factors are responsible for consolidating the material, and additionally, the castor oil adhesive used may influence the termites’ results reducing the attack due to its hydrophobic character. Composites made with polyurethanes based on vegetable oils, such as castor oil, demonstrate exceptional properties attributable to their characteristic hydrophobicity [52,53].
From Fig. 6a, it was found that the temperature (in isolation) and the interaction between the two factors (T × Comp) significantly affected the loss of mass of the experimental panels, however, the composition (in isolation) did not promote significant changes in this property.
Figure 6: Pareto charts: no-choice feeding test with drywood termites
Note: (a) Loss of mass. (b) Visual damage (Scores). (c) Mortality.
Damage (Fig. 6b) was significantly affected only by temperature, and in relation to mortality (Fig. 6c), it did not suffer significant changes from the isolated effects or the interaction effect.
In Fig. 7a it is possible to observe that for the interaction of T × Comp in loss of mass, for the temperatures of heat treatment of 0°C (reference) and 200°C the loss of mass decreased with the increase of the proportion of wood particles in the core, while for the 175°C the opposite occurred.
Figure 7: Main significant effects and interactions of factors on properties evaluated (drywood termite’s test)
Note: (a) Loss of mass × Comp. (b) Loss of mass × T. (c) Damage × T. The compositions (Comp) were represented only by the proportion of wood particles in the core.
About the influence of T in mass loss (Fig. 7b), 0°C presented a higher mean, as expected for the untreated panels (0.55%), and differed statically of 175°C and 200°C that did not differ from each other and presented means of 0.33% (smaller mean) and 0.36%, respectively. Related to the scores of visual damage × T (Fig. 7c), the higher the temperature, the higher the damage, and the temperature of 200°C differed statistically of 0°C and 175°C.
3.2 Subterranean (Arboreal) Termite Resistance Test
The p-values of the normality test and homogeneity of variances were higher than the significance level (p < 0.05) in all responses, which validates the results of the Tukey test (p < 0.05), and it is worth noting that the Johnson transformation was applied to the loss of mass and mortality results. Some studies indicate that wood mass loss is the main criterion for assessing wood preservatives [27] being a good parameter to evaluate the quality of the panels in relation to the resistance to biological attack.
For the evaluation of the no-choice feeding test with subterranean termites, were not found statistical differences for loss of mass (Fig. 8a) and mortality (Fig. 8c) results for all treatments assessed. Given this, since there were no statistical differences between the panels with chemical preservatives and the others for these properties, it is considered that all the experimental panels, even that one is without any treatment, presented good performance compatible with the panels available in the market.
Figure 8: Summary of results: no-choice feeding test with subterranean termites
Note: (a) Loss of mass. (b) Damage (Scores). (c) Mortality. Equal letters imply different treatments associated with statistically equivalent means using the Tukey mean contrast test (5% significance). Tr = Treatments.
This result is compatible with the founds of Ferro et al. [36] in which the authors concluded that the castor oil resin is efficient against termite attack, once in their work the untreated and treated panels did not show significant differences between the means of loss mass.
In [54], the termite resistance of the boards is affected by their constituent wood species. Thus, due to the promising results, can be considered that the Eucalyptus wood had good behavior to be used to produce OSB with castor oil adhesive, as most results did not demonstrate a significant difference between experimental and commercial panels. Adapting the classification of the AWPA E 1-22 [46] to the subterranean termites’ results, it is verified that all treatments evaluated presented slight termite mortality (16.46% to 32.48%).
Regarding damage (Fig. 8b), the results showed some statistical differences between them. For the damage classification, the higher the score, the less damage caused by the attack. In this way, T4, T5, and T7 had heavy attacks (score of 0–4) and no statistical difference between them. T1, T2, and T3 presented moderate attacks (score of 4–7) and no significant difference between them. T6 also showed moderate attack (score of 4–7) but with significant differences compared to T1, T2, and T3. In this sense, the commercial panel (T7) is among the panels with the greatest damage, while the panels with any type of thermal treatment (T1, T2) are among the ones with moderate attack.
In Fig. 9, the graphs and Pareto (ANOVA results) are presented regarding the properties evaluated in the no-choice feeding tests with subterranean termites, highlighting the normality and homogeneity of variances of the ANOVA residues (p-value < 0.05).
Figure 9: Pareto charts of properties evaluated in no-choice feeding tests with subterranean termites
Note: (a) Loss of mass. (b) Damage (Scores). (c) Mortality.
From Fig. 9, mortality and loss of mass were not significantly affected by the two factors alone nor by the interaction between them. However, it was noted that the interaction between the factors (T × Comp) significantly affected the visual damage values (Fig. 10). For the temperatures of heat treatment of 0° (reference) and 200°C the score of damage decreased with the increase in the proportion of wood particles in the core, while for the 175° the opposite occurred.
Figure 10: Interaction of significant factors on the visual damage (subterranean termites test)
Note: The compositions (Comp) were represented only by the proportion of wood particles in the core.
To drywood termite test, the mass loss was low (<1%). The visual damage was classified between surface and moderate, and the mortality was moderate. The temperature of thermal modification and temperature × composition of panels affected the loss of mass. For 200°C the loss of mass decreased with the increase of the proportion of wood particles in the core of panels. On the other hand, for 175°C the result was divergent. The tested temperatures do not provide meaningful results in the biological resistance of the panels tested. On the other hand, visual damage and mortality were not affected by the temperatures or compositions tested.
To subterranean (arboreal) termite test, regardless of the treatment tested, it was observed slight termite mortality. For visual damage, it was a moderate and heavy attack. Mortality and mass loss were not affected by temperature and composition nor by the interaction between them. For 200°C, the visual damage decreased with the increase in the proportion of wood particles in the core. On the other hand, for 175°C the result was divergent.
In general, the heat treatment did not improve the resistance against termite attack. However, the experimental panels had a performance similar to that presented by the commercial panels. Thus, these results provide evidence to support decision-makers about the use of innovative raw materials, environmentally friendly to produce OSB boards.
Acknowledgement: To São Paulo State University, University of Coimbra, SerQ, Federal University of Espírito Santo, Federal University of São Carlos, Imperveg and Vale do Cedro for the support during this research.
Funding Statement: This study was financed by Coordination for the Improvement of Higher Education Personnel, Brazil (CAPES, https://www.gov.br/capes/pt-br) (accessed on 22 September 2024), Finance Code 001 (ESS, FDM). Authors wish to gratefully acknowledge São Paulo State Research Support Foundation (FAPESP, https://fapesp.br/) (accessed on 22 September 2024) (CIC, grant number 2015/04660-0), and National Council for Scientific and Technological Development (CNPq, https://www.gov.br/cnpq/pt-br) (accessed on 22 September 2024) (grant numbers 308937/2021-0 (CIC), 306576/2020-1 (ECB), and 303099/2022-4 (JBP)).
Author Contributions: The authors confirm their contribution to the paper as follows: study conception and design: Estefani S. Sugahara, André M. A. Dias, Juarez B. Paes, André L. Christoforo, Edson C. Botelho, Alfredo M. P. G. Dias, Cristiane I. Campos; data collection: Fernanda D. Maffioletti, Juarez B. Paes, Estefani S. Sugahara; analysis and interpretation of results: André L. Christoforo, Cristiane I. Campos, Juarez B. Paes, Estefani S. Sugahara; draft manuscript preparation: Estefani S. Sugahara, André L. Christoforo, Cristiane I. Campos, Juarez B. Paes. All authors reviewed the results and approved the final version of the manuscript.
Availability of Data and Materials: Data is available on request from the authors.
Ethics Approval: Not applicable.
Conflicts of Interest: The authors declare that they have no conflicts of interest to report regarding the present study.
References
1. Marques B, Tadeu A, Almeida J, António J, de Brito J. Characterisation of sustainable building walls made from rice straw bales. J Build Eng. 2020;28:1–12. doi:10.1016/j.jobe.2019.101041. [Google Scholar] [CrossRef]
2. Dauletbek A, Li H, Xiong Z, Lorenzo R. A review of mechanical behavior of structural laminated bamboo lumber. Sustain Struct. 2021;1:1–18. doi:10.54113/j.sust.2021.000004. [Google Scholar] [CrossRef]
3. Yang P, Shen A, Cao Y, Zhu Z, Liu X, Zhou Z, et al. Effects of air-plasma treatment in enhancing the mechanical properties of oriented strand boards. Int J Adhes Adhes. 2023;125:1–9. doi:10.1016/j.ijadhadh.2023.103435. [Google Scholar] [CrossRef]
4. Shan B, Wang G, Lei P, Li T, Xiao Y, Qin S, et al. Experimental research on formaldehyde emission characteristics from glubam by climate chamber test. Sustain Struct. 2023;3:1–14. doi:10.54113/j.sust.2023.000027. [Google Scholar] [CrossRef]
5. Cappellazzi J, Konkler MJ, Sinha A, Morrell JJ. Potential for decay in mass timber elements: a review of the risks and identifying possible solutions. Wood Mater Sci Eng. 2020;15:351–60. doi:10.1080/17480272.2020.1720804. [Google Scholar] [CrossRef]
6. Akbar MI, Armynah B, Tahir D. Sandwich composite structure (SCSreview of wood-based materials with polymers and metals as a filler for electromagnetic interference (EMI) shielding. Ind Crops Prod. 2024;215:1–10. doi:10.1016/j.indcrop.2024.118619. [Google Scholar] [CrossRef]
7. Nicacio MA, Paes JB, Bufalino L, Lopez YM, Sousa SF, Verly Lopes DJ. Effect of silica bionanoparticles obtained from rice husk for the treatment of wood of Pinus elliottii. J Wood Chem Technol. 2022;42:158–70. doi:10.1080/02773813.2022.2055074. [Google Scholar] [CrossRef]
8. Zhang D, Gong M, Zhang S, Zhu X. A review of tiny houses in North America: market demand. Sustain Struct. 2022;2:1–13. doi:10.54113/j.sust.2022.000012. [Google Scholar] [CrossRef]
9. Copak A, Jirouš-Rajković V, Španić N, Mikleĉić J. The impact of post-manufacture treatments on the surface characteristics important for finishing of OSB and particleboard. Forests. 2021;12:1–12. doi:10.3390/f12080975. [Google Scholar] [CrossRef]
10. Wang Z, Xie W, Lu Y, Li H, Wang Z, Li Z. Dynamic and static testing methods for shear modulus of oriented strand board. Constr Build Mater. 2019;216:542–51. doi:10.1016/j.conbuildmat.2019.05.004. [Google Scholar] [CrossRef]
11. FAO. Yearbook of forest products 2020. Rome: Food and Agriculture Organization of the United Nations; 2022. [Google Scholar]
12. Ferro FS, Silva DAL, Rocco Lahr FA, Argenton M, González-García S. Environmental aspects of oriented strand boards production. A Brazilian case study. J Clean Prod. 2018;183:710–9. doi:10.1016/j.jclepro.2018.02.174. [Google Scholar] [CrossRef]
13. Fiorelli J, Bueno SB, Cabral MR. Assessment of multilayer particleboards produced with green coconut and sugarcane bagasse fibers. Constr Build Mater. 2019;205:1–9. doi:10.1016/j.conbuildmat.2019.02.024. [Google Scholar] [CrossRef]
14. Gonçalves FG, Alves SD, de Alcântara Segundinho PG, de Oliveira RGE, Paes JB, Suuchi MA, et al. Feasibility of incorporating thermally treated lignocellulosic waste in particleboard composites. Eur J Wood Wood Prod. 2022;80:647–56. doi:10.1007/s00107-022-01804-8. [Google Scholar] [CrossRef]
15. de Carvalho Araújo CK, Bigarelli Ferreira M, Salvador R, de Carvalho Araújo CKC, Camargo BS, de Carvalho Araújo Camargo SK, et al. Life cycle assessment as a guide for designing circular business models in the wood panel industry: a critical review. J Clean Prod. 2022;355:1–14. doi:10.1016/j.jclepro.2022.131729. [Google Scholar] [CrossRef]
16. Rowell RM. Handbook of wood chemistry and wood composites. 43rd ed. Boca Raton: CRC Press; 2005. doi:10.1201/9780203492437. [Google Scholar] [CrossRef]
17. Paes JB, Maffioletti FD, Silva MR, Ramalho AHC, de Medeiros JR, Lopez YM, et al. Biological resistance of sandwich particleboard made with sugarcane, thermally-treated Pinus wood and malva fiber. J Wood Chem Technol. 2022;42:171–80. doi:10.1080/02773813.2022.2056612. [Google Scholar] [CrossRef]
18. Brocco VF, Paes JB, Da Costa LG, Kirker GT, Brazolin S. Wood color changes and termiticidal properties of teak heartwood extract used as a wood preservative. Holzforschung. 2020;74:233–45. doi:10.1515/hf-2019-0138. [Google Scholar] [CrossRef]
19. Rowell RM. Chemical modification of wood: a short review. Wood Mater Sci Eng. 2006;1:29–33. doi:10.1080/17480270600670923. [Google Scholar] [CrossRef]
20. Andrade JKB, Paes JB, Batista DC, Medeiros JR, Trianoski R. Resistance to weathering and color stability of thermally modified eucalyptus wood for deck. J Build Eng. 2024;82:1–12. doi:10.1016/j.jobe.2023.108143. [Google Scholar] [CrossRef]
21. Navi P, Sandberg D. Chapter 9: heat treatment. In: Fenter F, editor. Thermo-hydro- mechanical processing of wood. Boca Raton: Taylor & Francis Group; 2011. p. 373. [Google Scholar]
22. Del Menezzi CHS, de Souza RQ, Thompson RM, Teixeira ED, Okino EYA, da Costa AF. Properties after weathering and decay resistance of a thermally modified wood structural board. Int Biodeterior Biodegr. 2008;62:448–54. doi:10.1016/j.ibiod.2007.11.010. [Google Scholar] [CrossRef]
23. Sugahara ES, Arroyo FN, de Campos CI, Botelho EC, Christoforo AL, Costa ML, et al. Thermal treatment of OSB panels: a systematic and bibliometric overview. Eur J Wood Wood Prod. 2022;80:1293–307. doi:10.1007/s00107-022-01849-9. [Google Scholar] [CrossRef]
24. Aro MD, Brashaw BK, Donahue PK. Mechanical and physical properties of thermally modified plywood and oriented strand board panels. For Prod J. 2014;64:281–9. doi:10.13073/FPJ-D-14-00037. [Google Scholar] [CrossRef]
25. Gonçalves FG, Paes JB, Lopez YM, de Alcântara Segundinho PG, de Oliveira RGE, Fassarella MV, et al. Resistance of particleboards produced with ligno-cellulosic agro-industrial wastes to fungi and termites. Int Biodeterior Biodegr. 2021;157:1–7. doi:10.1016/j.ibiod.2020.105159. [Google Scholar] [CrossRef]
26. Ünsal Ö, Nami Kartal S, Candan Z, Arango R, Clausen CA, Green F. Preliminary investigation of biological resistance, water absorption and swelling of thermally compressed pine wood panels. Stockholm, Sweden: IRG Secretariat; 2008. [Google Scholar]
27. Hassan B, Morrell JJ. Termite testing methods: a global review. J Test Eval. 2021;49:4607–36. doi:10.1520/JTE20200455. [Google Scholar] [CrossRef]
28. Karlinasari L, Sejati PS, Adzkia U, Arinana A, Hiziroglu S. Some of the physical and mechanical properties of particleboard made from betung bamboo (Dendrocalamus asper). Appl Sci. 2021;11:1–13. doi:10.3390/app11083682. [Google Scholar] [CrossRef]
29. Gonçalves FG, de Alcântara Segundinho PG, Fassarella MV, Minini D, Tinti VP, Lopez YM, et al. Relationship between elastic and biological properties in eucalypt wood waste particleboards. Rev Mater. 2021;26:1–14. doi:10.1590/S1517-707620210003.13048. [Google Scholar] [CrossRef]
30. Han G, Cheng W, Manning M, Eloy P. Performance of zinc borate-treated oriented structural straw board against mold fungi, decay fungi, and termites–a preliminary trial. BioResources. 2012;7:2986–95. doi:10.15376/biores.7.3.2986-2995. [Google Scholar] [CrossRef]
31. Marcon B, Viguier J, Candelier K, Thevenon M, Butaud J, Pignolet L, et al. Heat treatment of poplar plywood: modifications in physical, mechanical and durability properties. iForest. 2023;16:1–9. doi:10.3832/ifor4159-015. [Google Scholar] [CrossRef]
32. Nunes AM, Regazzi AJ, Gonçalves FG, Paes JB, Almeida ATS, Batista DC. Resistance to biodeterioration of plywood manufactured with veneers treated with boron compounds. J Indian Acad Wood Sci. 2023;20:138–48. doi:10.1007/s13196-023-00322-1. [Google Scholar] [CrossRef]
33. Cahyono TD, Yanti H, Massijaya MY, Iswanto AH, Uluputty MR. The durability of OSB composite plywood with avocado, mahogany, and pine wood veneers against drywood and subterranean termites. IOP Conf Ser Earth Environ Sci. 2019;260. doi:10.1088/1755-1315/260/1/012067. [Google Scholar] [CrossRef]
34. Malik J, Aini EN, Trisatya DR, Suhartana S, Wahyudi I, Augustina S. Dimensional stability and durability of hybrid sandwich panel made from oil palm lumber, sengon and gmelina with boron-alum impregnation. Wood Res. 2022;67:1017–31. doi:10.37763/wr.1336-4561/. [Google Scholar] [CrossRef]
35. Febrianto F, Sahroni, Hidayat W, Bakar ES, Kwon GJ, Kwon JH, et al. Properties of oriented strand board made from Betung bamboo (Dendrocalamus asper (Schultes.f) Backer ex Heyne). Wood Sci Technol. 2012;46:53–62. doi:10.1007/s00226-010-0385-8. [Google Scholar] [CrossRef]
36. Ferro F, Hendrigo de Almeida T, Icimoto FH, Antonio F, Lahr R, Dos Santos L, et al. Performance of OSB with different preservative treatments to dry wood termites attack. In: WCTE 2016–World Conference on Timber Engineering, 2016; Vienna; p. 1–8. [Google Scholar]
37. Cheng Q, Tang JD, Zhou C, Jiang W, Hu L, Nicholas DD, et al. Performance of oriented strand board made with soy substituted resin in termite choice tests with southern yellow pine. For Prod J. 2021;71:199–208. doi:10.13073/FPJ-D-21-00011. [Google Scholar] [CrossRef]
38. Lee M, Kang E-C, Lee S-M. Decay and termite resistance performance of structural particleboard. In: 5th International Conference on Bio-Based Building Materials, 2023; Vienna, Austria; p. 1–5. [Google Scholar]
39. Tascioglu C, Yoshimura T, Tsunoda K. Biological decay and termite resistance of post-treated wood-based composites under protected above-ground conditions: a preliminary study after 36 months of exposure. BioResources. 2013;8:833–43. [Google Scholar]
40. Filho LEC, Steigenberger AC, Martins RHB, Junior WEL, Favarim HR, de Jesus Agnolon Pallone EM, et al. Oriented strand board panels of residual reforestation wood with Al2O3 nanoparticles. Ind Crops Prod. 2023;200:1–7. doi:10.1016/j.indcrop.2023.116777. [Google Scholar] [CrossRef]
41. Kumar C, Leggate W. An overview of bio-adhesives for engineered wood products. Int J Adhes Adhes. 2022;118:1–15. doi:10.1016/j.ijadhadh.2022.103187. [Google Scholar] [CrossRef]
42. Laatiri Y, Sammouda H, Aloulou F. Bio-PCM Panels composed of renewable materials interact with solar heating systems for building thermal insulation. J Renew Mater. 2024;12:771–98. doi:10.32604/jrm.2024.047022. [Google Scholar] [CrossRef]
43. Sugahara E, Dias A, Arroyo F, Christoforo A, Costa ML, Botelho EC, et al. Study of the influence of heat treatment on OSB panels produced with eucalyptus wood in different layer compositions. Forests. 2022;1–18. doi:10.3390/f13122083. [Google Scholar] [CrossRef]
44. BSI ES. BS EN 300—Oriented strand boards (OSB). Definitions, classification and specifications. Brussels: CEN-European Committee for Standardization; 2006. p. 1–24. [Google Scholar]
45. ABNT AB de NT. ABNT NBR 16936—edificações em light wood frame (In Portuguese). Rio de Janeiro: Associação Brasileira de Normas Técnicas; 2023. p. 1–57. [Google Scholar]
46. AWPA. AWPA E1-22 Laboratory Methods for evaluating the termite resistance of wood-based materials: Choice and no-choice tests. Clermont: American Wood Protection Association, Inc.; 2022. p. 1–6. [Google Scholar]
47. AWPA. E30-22 Standard method for evaluating natural decay resistance of woods using laboratory decay tests. Clermont: American Wood Protection Association, Inc.; 2022. p. 1–4. [Google Scholar]
48. Brito JP. Ação de cupins de madeira seca (Cryptotermes brevis) e de solo (Nasutitermes corniger) nas madeiras termorretificadas de Pinus taeda e de Corymbia citriodora (Thesis). Universidade de São Paulo Escola Superior de Agricultura Luiz de Queiroz: Piracicaba; 2015. p. 1–104 (In Portuguese). [Google Scholar]
49. IPT. IPT/DIMAD D-2: ensaio acelerado de laboratório da resistência natural ou madeira preservada ao ataque de térmitas do gênero Cryptotermes (Fam. Kalotermitidae). São Paulo: Instituto de Pesquisas Tecnológicas/Divisão de Madeiras; 1980 (In Portuguese). [Google Scholar]
50. Maistrello L. Termites and standard norms in wood protection: a proposal targeting drywood termite. In: Khan A, Ahmad W, editors. Termites and sustainable management. Cham, Switzerland: Springer International Publishing; 2018. p. 261–87. doi:10.1007/978-3-319-68726-1_12 [Google Scholar] [CrossRef]
51. Rocha Medeiros J. Efeito da modificação térmica na resistência biológica da madeira de eucalipto (Master Dissertation). Universidade Federal do Espírito Santo: Jerônimo Monteiro; 2021 (In Portuguese). [Google Scholar]
52. Mubofu EB. Castor oil as a potential renewable resource for the production of functional materials. Sustain Chem Process. 2016;4. doi:10.1186/s40508-016-0055-8. [Google Scholar] [CrossRef]
53. Nelson PM, Nascimento EM, Nisgoski S, Magalhães WLE, Neto C, Azevedo S, et al. Caracterização física e térmica de compósito de poliuretano derivado de óleo de mamona associado com partículas de bambu. Polímeros. 2013;23:201–5 (In Portuguese). doi:10.1590/s0104-14282013005000007. [Google Scholar] [CrossRef]
54. Lee M, Kang EC, Lee SM, Yoon SM. Mechanical properties of structural particleboard and termite and decay resistance. BioResources. 2023;18(3):6169–82. doi:10.15376/biores.18.3.6169-6182. [Google Scholar] [CrossRef]
Cite This Article
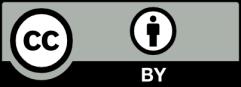
This work is licensed under a Creative Commons Attribution 4.0 International License , which permits unrestricted use, distribution, and reproduction in any medium, provided the original work is properly cited.