Open Access
ARTICLE
Histological Assessment of Bone Regeneration in the Maxilla with Homologous Bone Graft: A Feasible Option for Maxillary Bone Reconstruction
1 Graduate Center of Faculdade Redentor, CLIVO, Rio de Janeiro, Brazil
2 Periodontics and Oral Medicine Department, University of Michigan School of Dentistry, Ann Arbor, MI, 48109, USA
3 Centre for Interdisciplinary Research in Health (CIIS), Universidade Católica Portuguesa, Viseu, 3504-505, Portugal
* Corresponding Author: Gustavo Vicentis Oliveira Fernandes. Email:
(This article belongs to the Special Issue: Compatible Biological Materials Applied to Regenerative Medicine: from the Bench to the Bedside )
Journal of Renewable Materials 2024, 12(1), 131-148. https://doi.org/10.32604/jrm.2023.043940
Received 17 July 2023; Accepted 18 October 2023; Issue published 23 January 2024
Abstract
Bone biomaterials have been increasingly used to reconstruct maxillary atrophic ridges. Thus, the aim of this study was to evaluate bone reconstruction in the maxilla using a homologous cortico-cancellous FFB (lyophilized) graft and verify its reliability. Eight individuals were included from 2014 to 2018. The first surgery was performed to install homologous bone blocks in the maxilla. The period of the second intervention varied between 5 months and 15 days to 11 months (≈7.93 months). The biopsies were taken from the central region of the matured graft during the surgery for implant placement. All patients presented clinical and radiographic conditions for the installation of dental implants. There was a 100% of survival rate. The histological assessment showed that the homologous block bone graft was an osteoconductive biomaterial, with connective tissue present, and newly formed bone juxtaposed on its surface. There were bone trabeculae with osteocytes and active osteoblasts with connective tissue in the mineralization process; the remodeling process can be found through the reverse lines. A limited focus of necrosis with fibrosis was detected, with small resorption and areas of inflammatory infiltrate, but without clinical significance. The homologous block bone graft can be considered a feasible option to substitute the autogenous bone graft (gold standard), with predictable clinical and favorable histological results. The patients had a shorter surgical period, low morbidity, and an unlimited amount of biomaterial available at an accessible cost.Keywords
The increased necessity of alveolar ridge augmentation for oral rehabilitation with dental implants has made bone grafting a cornerstone. The autogenous (autologous) bone graft is considered the “gold standard” biomaterial to reconstruct atrophic ridges due to its osteogenic, osteoinductive, and osteoconductive potential [1,2]. Nevertheless, it has unfavorable aspects such as high morbidity, greater discomfort at the donor site, and increased surgical time. Other postoperative complications may also occur, namely paresthesia, bleeding, and high-level pain [3]. Then, a possible substitute for this bone is the allograft, also named homologous or allogenous bone. It dates back more than 100 years of experience in orthopedics and more than 25 years in dentistry, with favorable results [4,5]. This fact makes it a reliable choice as an option biomaterial.
In the 1990s, the American Association of Tissue Bank (AABT) described the first protocol for the structuring, operation, selection rules, capture rules, processing, storage, and distribution of tissues for transplant purposes. This protocol was also accepted by the European Association of Tissue Bank (EATB), serving as the basis for the transplant process worldwide [6]. Thus, in less than ten years, data published in early 2000 showed that allogeneic bone grafts were Europe’s second most-used alternative approach, losing only the autogenous bone [7]. The first report of this bone graft in maxillofacial surgery is dated 1992, which was used alone or mixed with autologous bone graft. Four jaws and six atrophic mandibles were reconstructed, and after an average follow-up of 26.3 months, the results were considered clinically satisfactory, with a more than 98% success rate [8].
Allogeneic grafts are available as fresh, frozen, or lyophilized (frozen and dried; mineralized [inorganic bone matrix] or demineralized [organic bone matrix]), available in blocks or particles [9]. The bone to be used is available fresh (without processing), fresh-frozen (fresh-frozen, FFBA), or lyophilized (freeze-dry). Frozen allografts are stored in freezers at −70°C, decreasing antigenicity and trying to preserve their properties. Freeze-dried bone undergoes dehydration from a frozen allograft [10]. It can be classified as fresh-frozen bone (FFB), freeze-dried bone (FDB), or demineralized freeze-dried bone (DFDB). They can have only a cancellous portion (higher rate of revascularization, resorption, and creeping substitution of host cells), only a cortical part (slow remodeling rate but more structural strength), or then a mix of cortico-cancellous portions (fast integration due to rapid revascularization and have a thin outer cortical layer apt to securely lock the fastening screw, presenting better initial graft stability) [11]. Therefore, for the appropriate selection and use, the professional must be familiarized with the bone properties [12].
The impact of freezing the allograft bone on the biological immune response was evaluated. Homologous blocks were implanted in rats’ tibia defects and compared to the gold standard. The answer was analyzed on the first day and after 2 and 4 months. Antibodies were absent, and the data supported that, in the long term, there were no significant immunological responses compared with the fresh autogenous bone graft [13]. Even though it has questionable properties, the osteoinduction potential of allogenic bone blocks may increase cell proliferation, bone cell metabolism, and proliferation of the mineralization (histological nodules) [14].
There is a lack of studies regarding the histological aspects and demonstrating the clinical applicability and results of the homologous graft. It causes restrictions and reduced knowledge by professionals and patients. Thus, this case series aimed to histologically evaluate the bone reconstruction in the maxilla using a homologous cortico-cancellous FFB (lyophilized) graft and verify its reliability.
This case series followed the Declaration of Helsinki (1975, updated 2013). It was approved by the Local Ethical Committee (RCL #130121). After the explanation, evaluation, and agreement, the Informed Consent was signed. The procedures were performed at the Graduate Center of Faculdade Redentor at the CLIVO clinic (Rio de Janeiro, Brazil). All blocks came from the Bone Bank—UNIOSS® (Brazil). Only one licensed author developed all block reconstruction surgeries (S.H.G.M.).
The homologous bone graft was donated from patients without any pathologies, such as osteoporosis, osteonecrosis, rheumatoid arthritis, lupus erythematosus, and sexually transmitted disease, with ages between 17 and 55 years. From the bone removed, it was collected for culture and then placed in simple envelopes in antibiotic solution (vancomycin and gentamicin), hermetically sealed, and sent under refrigeration (−4°C) to the Tissue Bank for immediate processing. Portable refrigerators are used in transportation. Once sent to the Tissue Bank, the pieces were processed in the operating room to remove their adventitious elements—periosteum, muscles, and fascia—and then packed in sterile casings. Cultures are taken throughout the process. The bone grafts were hermetically sealed and stored in a freezer at a temperature between −85°C and −110°C. Massive bone pieces go through this entire process and were even radiographed.
The null hypothesis is this type of bone did not permit adequate rehabilitation.
As inclusion criteria, (1) patients should need maxillary rehabilitation and reconstruction after evaluation of a tomography; (2) to accept receiving dental implants in the site grafted, to do a fixed rehabilitation; (3) no systemic disease; and (4) ≥18 years old. As exclusion, (1) patients cannot be currently using antibiotics, anti-inflammatories, or any medication/substance abuse that might influence the health or healing before starting the study; (2) any uncontrolled disease; (3) pregnant or nursing; (4) psychiatric problems or unrealistic expectations; (5) poor motivation or untreated periodontitis; (6) immunosuppressed or immunocompromised; and (7) smoker.
2.2 Surgical Protocol for Homologous Bone Graft Implantation
The treatment plan was based on the bone reconstruction for the pre-maxilla and later dental implant placement. The patients had a detailed anamnesis, laboratory tests, cone beam computed tomography (CBCT), and panoramic radiography done. All explanations and post-operative care were given before the treatment. Pre-surgical rinse with 0.2% chlorhexidine was used for 1 min. Then, bilateral anesthesia of the posterior, middle, and anterior-superior alveolar, nasopalatine, and greater palatine nerves was done using 3% prilocaine, 3% mepivacaine, and infiltration with 2% lidocaine. An incision with a 15C blade, running 45° on the crest of the ridge, slightly shifted towards the palate to avoid the incision border of the flap on the bone graft, which could cause exposure and contamination. Vertical relaxing incisions (made in the distal regions of 17 and 27) extended buccally beyond the mucogingival junction, and horizontal internal incisions were made to release and divide the soft tissue relaxing it, allowing greater mobility of the flap tissues and facilitating the closure by the first intention. The displacement of the full-thickness flap was performed, followed by careful horizontal incisions made in the periosteum of the basal portion of the flap, permitting stretching of the tissue, achieving higher mobility and posterior coaptation of the edges without tension. Then, decorticalization of the receptor bone area was done using small spherical burs (¼ and ½), producing small perforations in the cortical bone, promoting better blood flow to accelerate vascularization and incorporation of block bone grafts.
The blocks (from the tibia) were removed from the freezer and allowed to thaw in a sterile saline solution. A template was positioned in the region of the receptor site, permitting the obtention of the desired dimensions. They were achieved by cutting with diamond disks, modeled with Maxi-cut cutters, seeking a perfect and total adaptation. After, the bones were positioned with duckbill tweezers, and a perforation was performed larger than 1.6 mm in diameter in the blocks. A smaller perforation (1.3 mm ø) in the receptor bed was made with the purpose of fixation by the screws (2.0 mm ø) used. Two or three screws were used, transfixing the buccal and lingual cortical plates, to achieve the complete stability of the bone block. Areas not properly adapted were filled out with particulate synthetic bone grafts (Alobone® 0.5g, Osseocon Biomateriais, Brazil). All sites were covered with a resorbable collagen membrane. The flap was carefully repositioned, and simple interrupted sutures were made with 3-0 (Ethicon Suture, Silk Thread, Johnson & Johnson Health Care Systems Inc., Piscataway, USA).
The patient was instructed not to use the complete denture, reducing the possibility of suture dehiscence with consequent infection, necrosis, and bone graft loss. On the day of the surgery, two hours before the procedure, 4 mg of dexamethasone was taken and was continued until the 2nd day after surgery. Moreover, since day 0 post-operative, 600 mg of ibuprofen was administrated 12/12 h for 5 days, amoxicillin 500 mg thricely/day for 7 days, and paracetamol 750 mg 4/4 h only in case of pain on the first day was added to the regimen. Complementary was prescribed 1 g of vitamin C daily, starting one week before and continuing for one month, and one B-complex tablet after meals, starting one week before surgery and continuing for one month. Chlorhexidine 0.12% to gargle before surgery and bathe the region twice daily, up to 2 weeks after surgery. The suture was removed 15 days after surgery, and the total denture was relined with soft resin. The patient received strict orientation about the types of food and home care.
2.4 Implants Placement and Histological Procedure
Between 5 and 12 months, the patients were recalled following the second surgical procedure for implant placement. All blocks were gently trephined in the center of the bone block using a trephine bur drill 4.0 mm ø (inner diameter) (GTD Implants). One trephine was used per patient. The pieces were kept in a 10% buffered formaldehyde solution for 48 h and subjected to descaling with Morse’s solution (pH = 4.83) for 10 days. The samples were washed in running water, dehydrated in growing ethanol solutions, clarified in xylene, embedded in paraffin, cut in the Leica LM microtome to obtain a 5 µm thickness, and collected for histological preparation. The cuts were obtained and stained in hematoxylin-eosin (HE). The material was evaluated descriptively, considering the following parameters: inflammatory reaction, connective tissue, and bone. Histological sections were observed, discussed, and described (A.P.R.S. and J.C.H.F). Digital images were acquired. This was done using an image capture system (Sony Cyber-shot DSC-W300) coupled with the Feldmann Wild Leitz 1000 light microscope (BM 2000). Images with 20× magnification were obtained.
All images were evaluated to achieve the description. The following parameters were analyzed: 1. compact (cortical) bone without osteocytes; 2. connective tissue; 3. medullar (cancellous) area; 4. osteocytes; 5. new bone formed; 6. native bone (receptor site); 7. osteoblasts; 8. trabecular bone; 9. osteoclasts; 10. osteoid matrix; 11. non-vital mineralized tissue; 12. presence of necrosis; and 13. blood vessel.
This histological study was carried out on eight individuals (8 maxillae) from January 2014 to January 2018, 7 females and 1 male, aged between 53 and 63 (mean age 58 ± 5.3 years old), who needed surgery for bone volume augmentation, to improve the esthetic, and for later rehabilitation with dental implants. The first surgery was performed to install homologous bone blocks in the maxilla (Figs. 1–8), and the period of the second intervention varied between 5 months and 15 days to 11 months (mean average of 7.93 months). The biopsies were taken from the central region of the matured graft at the moment of the reopening for the implant placement procedure. All patients presented clinical and radiographic conditions for the installation of dental implants. There was a 100% of survival rate.
Figure 1: Case 1. Sequential images for the treatment: (A) initial X-ray; (B) surgical approach for block bone graft installation; (C) new panoramic after 6 months; and (D, E) a small sample was collected for histological analysis
Figure 2: Case 2. Sequential images for the treatment: (A–C) initial clinical presentation and panoramic exam (with and without the old prosthesis); (D) bone block prepared; (E) surgical placement of the block bone graft; (F) surgical approach for block bone graft installation; (G–H) flap raised to expose the block after 5 months and 15 days. Sample collected and implant placement (observed the presence of a small fracture in the block); (I) new panoramic after implants placed; and (J) final rehabilitation after 6 months of the implant placement
Figure 3: Case 3. Sequential images for the treatment: (A) surgical guide proof before surgery; (B) flap raised and blocks bone grafts placed (immediate thickness presented); (C–E) after around 6 months, sequential images of the trephine collecting material and material after harvested; (F–G) seven implants were placed to rehabilitate the patient
Figure 4: Case 4. Sequential images for the treatment: (A) flap raised after 6 months to fill the gap present in the mesial of tooth #23; (B–C) Block integrated; (D) the flap was repositioned, and the suture was done
Figure 5: Case 5. Sequential images for the treatment: (A) the initial smile of the patient complaining about the esthetic; (B) the implants were kept, and a block bone graft was placed to improve the esthetic. (C) Final aspect after suture
Figure 6: Clinical case 6. The flap reopened, showing the integration
Figure 7: Case 7. Sequential images for the treatment: (A–B) homologous bone graft adjusted and in position, with perforations (decorticalization) to improve the vascularization of the block; (C) occlusal image showing the bone block adaptation on the bedside
Figure 8: Case 8. Sequential images for the treatment: (A) surgical guide proof; (B–D) blocks exposed and integrated with ~1.0 mm of horizontal loss (periodontal probe); (E) screws removed; (F–G) sample collected with trephine; (H) guide in position for the implant placement
The cases were performed following the same sequence, varying the quantity and size of bone used to reconstruct but always keeping the same type of donor region (tibia), e.g., Fig. 1 shows 2 block bone grafts, and Fig. 3 with three blocks. Figs. 1–3 show the procedures done in all of them. Initially, a panoramic X-ray was requested for the primary assessment and overview (Figs. 1 and 2). CBCT was sequentially assessed to verify the necessary dimensions and volume of the bone graft. The surgery was scheduled after receiving the bone graft, and the surgical guide for the implant placement was prepared (Figs. 3 and 8).
The flap was raised according to the needed extension, and the receptor bedside was prepared to receive the homologous bone graft from the tibia. The block graft had an adequate cortical width (≥5 mm), length, height, and medullar area. After adjustments, the homologous bone was fixed using at least 2 screws, transfixing both cortical (buccal and lingual) of the bedside receptor. The immediate osseous volume obtained during the surgery was adequate for posterior rehabilitation (Figs. 1–8); therefore, after the healing period (between 5 and 12 months), a volume loss was expected and found due to tissue remodeling (Fig. 8). After the healing period, the patients were recalled for a new evaluation and panoramic/CBCT analysis (Figs. 1 and 2). Afterward, the surgical procedure for implant placement was scheduled, where the block was trephined to obtain the samples for histological processing (Figs. 1–3 and 8; macroscopic aspects of the histological piece [4 ø mm × 4 mm height]). The implant placement occurred without a significative event, except in one case, in which a small fracture in the block graft was observed (Fig. 2) during the implant installation (after healing); this fact did not harm the procedure and rehabilitation.
All images collected are presented in Fig. 9. The overview analysis shows that the homologous block bone graft was an osteoconductive biomaterial (Figs. 9F, 9G), with connective tissue present and newly formed bone juxtaposed on its surface. Cortical and cancellous bone, remodeling tissue, and new blood vessels were observed in all samples. The presence of vessels was the main characteristic present, which permitted the blood flow and supply for the repair/remodeling and new tissue. Newly formed bone found in Figs. 9H, 9I. Bone trabeculae with osteocytes, surrounded by connective tissue, can be observed in Fig. 9L. Active osteoblasts with connective tissue in the mineralization process and new bone were observed in Figs. 9G, 9M. The remodeling process can be found through the reverse lines, clearly seen in Fig. 9N. Foci of necrosis with fibrosis detected in Fig. 9O. Moreover, it was possible to describe areas of connective tissue with inflammatory infiltrate, but the greatest part did not have any signal of inflammation; fragments of loosely arranged connective tissue, with areas of myxoid appearance and intermingled bone trabeculae showing osteocytes, reversal lines, areas of osteogenic matrix deposition, osteoblastic paving, with few osteoclasts; peripherical and in continuity, devitalized mineralized tissue can be observed (the appearance suggests its replacement by compact bone); devitalized compact bone, with an absence of osteocytes and osteoblasts; and evidence of reabsorption.
Figure 9: (A–O) Histological analysis obtained. It is possible to observe neoangiogenesis and bone remodeling involving the bone graft used. Presence of osteoblasts and osteocytes present in the analysis. New bone formed on juxtaposition to the native bone, showing the osteoconduction principle. Number from 1 to 13 are showing specific parts found in each image (1.compact [cortical] bone without osteocytes; 2. connective tissue; 3. medullar [cancellous] area; 4. osteocytes; 5. new bone formed; 6. native bone [receptor site]; 7. osteoblasts; 8. trabecular bone; 9. osteoclasts; 10. osteoid matrix; 11. nonvital mineralized tissue; 12. presence of necrosis; and 13. blood vessel).
The ideal biomaterials [15] for bone graft procedures should possess the following characteristics: provide osteogenic, osteoinductive, and osteoconductive properties; stimulate the neo-angiogenesis process; absence of antigenic, teratogenic, or carcinogenic reactions; avoid systemic toxicity complications; assure satisfactory support and stability from mechanical properties point of view; hydrophilic nature of the surface properties and good interface with human bone; good handling in clinical condition and ability to be easy sterilized; and able to be supplied in sufficient quantities with reduced costs [16]. Therefore, there is not yet an ideal biomaterial with all those characteristics.
According to the literature [17], the anatomical origin of the bone graft is the factor that most influences postoperative bone resorption and remodeling. Therefore, a graft of iliac or tibia origin, with a greater volume of cancellous bone and also the presence of cortical, tends to resorb more than a graft of cranial or intraoral origin. In addition, bone from the mandibular ramus has an absorption rate between 5% and 28% [18], which can be higher, as demonstrated in another study, with resorption between 23% and 42% after a healing period of 4 months [19]. For parietal bone grafts, it showed a resorption rate of 16.2% after 6 months [20] and 19% after 1 year [21]. Then, the proximal tibia offers an excellent source of cancellous and cortical bone, and several authors have advocated tibial bone [19,21,22].
This study aimed to histologically evaluate the bone reconstruction in the maxilla using a homologous cortico-cancellous FFB (lyophilized) graft. This type of bone graft has some of the features abovementioned, such as (i) absence of antigenic effects, (ii) osteoconductive property, (iii) it is accepted by the body as not recognized as a foreign body by the host [23]. A recent study showed there is a high risk of malignancies in hematopoietic stem cell transplantation recipients later in life [24]. Still, there are not many reports about cancer associated with the mineral part of the homologous bone, which depends on the treatment done.
The tibia bone graft was selected in this study due to its adequate corticocancellous bony volume and availability. Even though iliac and tibial bone grafts are passive osteoconductive scaffolds, there is no reason to expect differences in fusion rates after remodeling [25]. Cancellous tissue offers a higher rate of revascularization and bone substitution, while cortical grafts have a slow remodeling rate but more structural strength. Corticocancellous blocks offer fast integration in the recipient site due to rapid revascularization. They have a thin layer on their outer cortical portion that permits securely locking the fastening screw, stabilizing the graft [11,26]. Whereas dental implants can be placed after 4 to 6 months when using block-type autogenous bone grafts, the healing period should be longer for bone graft materials from allogenic, xenogeneic, and alloplastic bone substitutes. The authors advised clinicians to provide an adequate healing period of at least 12 months [27]. This period of healing was not followed in our study.
The results obtained from the eight samples using homologous bone graft support the viability of the homologous bone block application to prepare the ground for future rehabilitation with dental implants. Similar results for allogenic bone showing its efficacy were presented through the other clinical studies, in which the authors reported its feasibility alternative to the autogenous bone, mainly if considering the tissue limitations during the treatment planning [28,29]. Otherwise, the bone substitution during the remodeling process can be regarded as partial and slower than the gold standard [29]. Depending on the tissue volume used for reconstruction, the entire healing process will last months. Moreover, it can be slower in homologous than in autologous bone grafts, likely due to a low residual immunological discrepancy or cell viability [30] and lack of osteoinduction and osteogenesis capacity. Furthermore, it is important to highlight that block-type autogenous bone is still considered the gold standard biomaterial for reconstruction. A study [31] presented the results of reconstructive procedures and implant placement of eleven horizontal bone deficiencies. The authors obtained CT measurements before surgery (T0) and after 6 months (T1) and 5 years (T2), showing an adequate volume reconstruction. The mean total thickness of the ridge was 4.7 mm (T0), 7.6 mm (T1), and 6.9 mm (T2); the average increase in horizontal thickness was 2.9 mm at T1 and 2.2 mm at T2. These thickness gains were similar to our results, but we used the homologous bone graft.
A systematic study also analyzed the efficiency and predictability of allogenic bone [32] in 110 patients. The surgeries were made in the anterior regions with a short-term follow-up of less than 3 years. The authors obtained ≥90% of the clinical graft incorporation and 99% to 100% of the implant survival rate. Otherwise, they considered limited scientific evidence available to establish the efficacy of the treatment (block bone integration) for an increase of the ridge alveolar function and long-term implant survival. Our study found 100% for incorporation of the blocks. Therefore, for optimal healing (good vascularization and no necrosis), the graft must be immobilized and well-stabilized without any physical trauma during the healing period [33]. Micromovements around 20 µm are enough to harm bone remodeling and may result in reduced/no bone integration or encapsulation by fibrous tissue. Our study had a histologically limited presence of fibrosis and necrosis. These facts indicate that, in a specific area, there was a lack of ideal adaptation between the homologous block and the receptor bed, micromovements, or possible mucosal pressure on the fixation screws during the healing [33]. Therefore, those observations did not impair implant placement and rehabilitation.
Other authors [34] assessed the bone changes through tomographic, histologic, and histomorphometric analysis, comparing three cortico-cancellous FFB graft sources (proximal tibia, iliac crest, and femur bones). There was progressive graft resorption, which was significantly different among the three groups. After 4 months, the presence of graft was 50.78% (±10.43); at 6 months, it was 32.77% (±7.84); and after 8 months, it was 13.02% (±3.86), suggesting that 4 months could be sufficient time for integration, maturation, and adequate vascularization quality; and 8 months with the largest rate of graft resorption, which could render the grafts unfavorable for implant placement. Moreover, the authors reported the presence of newly formed bone with osteocytes next to the grafted bone in all groups, but significantly lower at 4 months, which they considered the most favorable for implant placement. Another study recommended that dental implants should predictably be positioned primarily within the original host bone [29]. Our study kept at least 5 months of integration (healing), and we agree there was minimal resorption without any commitment to the implant placement and presence of osteocytes and osteoblast cells; furthermore, significant resorption was not observed in the cases treated after 6 months, but one fracture was verified and could be explained by the drilling done in the grafted bone.
In the cases treated in this study, no significant event was observed. If complications were found, they could lead to the partial or complete loss of the bone graft used. Thus, the professionals must always comply with the following precautions in case of bone graft block reconstruction [35]: (1) adequate blood supply to the graft; (2) adequate modeling and fixation of the block; (3) covering of the bone block and filling the gaps with slowly resorbable particulate bone graft; (4) releasing incisions for a tension-free flap; (5) avoidance of load or compression on the reconstructed area with removable prostheses; (6) wait for the sufficient healing period to allow for the successful integration of the grafts without simultaneous dental implant placement; (7) avoidance of over-contouring with block bone grafts that could cause wound dehiscence.
Moreover, with the evolution of biomaterials and technological tools applied to the medical area, new possibilities have emerged in bone reconstruction [36–38], even substituting autogenous and homologous bone grafts. Plenum® Oss-3Dβ [39] employs a superior level of technology, using a DICOM file for manufacturing by an additive technique using a 3D ceramic printer. This equipment will make a personalized alloplastic graft through lithography-based Ceramic Manufacturing technology. This process involves printing the virtual model with β-TCP slurry. Applying this process reduces the trans-operative time due to enhanced graft adaptation on the bedside, resulting in lower discomfort and better details of anatomy with high design fidelity [40]. Moreover, β-TCP has been highly used as a bone graft biomaterial due to its higher degradability and rapid replacement by new bone [41,42]. Hence, it can be considered one of the best current options.
The histological evidence of new bone formation from homologous bone in humans has been verified. Osteocytes trapped in the bone matrix and medullary spaces suggested vital tissue and new bone formation [43]. In this study, osteocytes were present in the samples within the new bone formed due to the homologous block’s osteoconduction processes. This property was observed in the histological images, where only the autogenous bone graft carries vital cells to the recipient site. In addition, investigators have chronologically established that angiogenesis precedes osteogenesis [44]. A histomorphometry study [45] assessed 10 patients who underwent alveolar ridge reconstruction with FFB. Six cases were grafted with an iliac crest and 4 with a femoral head. After 6 months, the femoral group had faster angiogenesis, with a significantly higher percentage of vessels and intense osteoblast activity close to the vascularized and non-mineralized tissue. On the other hand, this group had more bone resorption than the iliac group.
In a study by Sendyk et al. [46], allografts were vascularized and showed partial cell repopulation after 12 weeks. Similarly, our study also presented vital cells, proving the cellular repopulation of the homologous graft, but within a greater period of healing. Upon clinical and histological comparison between allogenic and autogenous bone grafts used in the same patient, however, in the maxillary sinus lifting, there were no differences in bone neoformation after 7 months, demonstrating that the osteogenic potential of the receptor bed was similar in the presence of both materials [47]. In another study comparing contralaterally autogenous and homologous bone blocks, greater resorption was clinically observed in the homologous, with lower density or resistance to drilling at the time of implant insertion, without compromising the primary stability (both with 40 N.cm). On the other hand, histological findings were similar after 6 and 8 months, showing vital tissue [48].
This study had some limitations. It was a case series with a few patients that evaluated the histological behavior of the homologous bone block graft, in the short term, in the maxilla. Assessing the histology in another period is unreasonable because we took advantage of the moment to place implants to rehabilitate the patients. Furthermore, there was no standard for the blocks’ quantity, volume, or size because we intended to evaluate their integration in the maxilla and not the reconstructions themselves.
According to the findings and respecting the limitation, the homologous block bone graft can be considered a viable and feasible option to substitute the autogenous bone graft (gold standard), with predictable clinical and favorable histological results. The patients had a shorter surgical period, low morbidity, and an unlimited amount of biomaterial available at an accessible cost. The null hypothesis was rejected because the homologous block bone permitted adequate oral rehabilitation. More studies should be carried out to evaluate the results in the long term and also use and compare results with other technologies, such as those presented by Plenum® Oss-3Dβ.
Acknowledgement: Faculdade Redentor—Clivo, Rio de Janeiro, Brazil.
Funding Statement: The authors received no specific funding for this study.
Author Contributions: The authors confirm contribution to the paper as follows: study conception and design: SHGM, APRS, GVOF; data collection: SHGM, APRS; analysis and interpretation of results: SHGM, APRS, JCHF, GVOF; draft manuscript preparation: SHGM, APRS, JCHF, GVOF. All authors reviewed the results and approved the final version of the manuscript.
Availability of Data and Materials: All data obtained was inserted in this article.
Ethics Approval: All patients gave their informed consent for inclusion before they participated in the study. It was approved by the Local Ethical Committee Faculdade Redentor—Clivo, Rio de Janeiro, Brazil (RCL #130121).
Conflicts of Interest: The authors declare that they have no conflicts of interest to report regarding the present study.
References
1. Wang, W., Yeung, K. W. K. (2017). Bone grafts and biomaterials substitutes for bone defect repair: A review. Bioactive Materials, 2, 224–247. [Google Scholar] [PubMed]
2. Fernandes, G. V. O., Calasans-Maia, M., Mitri, F. F., Bernardo, V. G., Rossi, A. et al. (2009). Histomorphometric analysis of bone repair in critical size defect in rats calvaria treated with hydroxyapatite and zinc-containing hydroxyapatite 5%. Key Engineering Materials, 396–398, 15–18. https://doi.org/10.4028/www.scientific.net/KEM.396-398.15 [Google Scholar] [CrossRef]
3. Tonk, G., Yadav, P. K. R., Agarwal, S., Jamoh, K. (2022). Donor site morbidity in autologous bone grafting—A comparison between different techniques of anterior iliac crest bone harvesting: A prospective study. Journal of Orthopaedics, Trauma and Rehabilitation, 57(5), 490–492. [Google Scholar]
4. Peleg, M., Sawatari, Y., Marx, R. N., Santoro, J., Cohen, J. et al. (2010). Use of cortical cancellous allogeneic bone blocks for augmentation of alveolar bone defects. The International Journal of Oral and Maxillofacial Implants, 25, 153–162. [Google Scholar] [PubMed]
5. Lima, A. M. H., Dantas, E. M., Abreu, T. C., Filho, A. A. L., Sendyk, W. (2012). Avaliação do ganho ósseo em espessura nas reconstruções de pré-maxila com transplantes homógenos (Brazilian Edition). ImplantNews, 9(1), 21–27. [Google Scholar]
6. Pelegrine, A. A., Macedo, L. G. S., Cosmo, L. A. M. (2008). Transplantes Ósseos na Odontologia (Brazilian Edition)Nova Odessa, São Paulo, Brazil: Napoleão. [Google Scholar]
7. Delloye, C., Cornu, O., Druez, V., Barbier, O. (2007). Bone allografts: What they can offer what they cannot. The Journal of Bone Joint Surgery, 89, 574–579. [Google Scholar] [PubMed]
8. Perrott, D. H., Smith, R. A., Kaban, L. B. (1992). The use of fresh frozen allogenic bone for maxillary and mandibular reconstruction. The International Journal of Oral and Maxillofacial Surgery, 21(5), 260–265. https://doi.org/10.1016/S0901-5027(05)80732-5 [Google Scholar] [PubMed] [CrossRef]
9. Tournier, P., Guicheux, J., Paré, A., Veziers, J., Barbeito, A. et al. (2021). An extrudable partially demineralized allogeneic bone paste exhibits a similar bone healing capacity as the gold standard bone graft. Frontiers in Bioengineering and Biotechnology, 9, 658853. https://doi.org/10.3389/fbioe.2021.658853 [Google Scholar] [PubMed] [CrossRef]
10. Campana, V., Milano, G., Pagano, E., Barba, M., Cicione, C. et al. (2014). Bone substitutes in orthopaedic surgery: From basic science to clinical practice. Journal of Materials Science. Materials in Medicine, 25(10), 2445–2461. https://doi.org/10.1007/s10856-014-5240-2 [Google Scholar] [PubMed] [CrossRef]
11. Giannoudis, P. V., Dinopoulos, H., Tsiridis, E. (2005). Bone substitutes: An update. Injury-International Journal of the Care of the Injured, 36(Suppl 3), S20–S27. https://doi.org/10.1016/j.injury.2005.07.029 [Google Scholar] [PubMed] [CrossRef]
12. Beer, A. J., Tauro, T. M., Redondo, M. L., Christian, D. R., Cole, B. J. et al. (2019). Use of allografts in orthopaedic surgery: Safety, procurement, storage, and outcomes. Orthopaedic Journal of Sports Medicine, 7(12), 2325967119891435. https://doi.org/10.1177/2325967119891435 [Google Scholar] [PubMed] [CrossRef]
13. Coutinho, L. F., Amaral, J. B., Santos, E. B., Martinez, E. F., Montalli, V. A. M. et al. (2017). Presence of cells in fresh-frozen allogeneic bone grafts from different tissue banks. Brazilian Dental Journal, 28(2), 1–6. https://doi.org/10.1590/0103-6440201701206 [Google Scholar] [PubMed] [CrossRef]
14. Sousa, A. P. S., Martinez, E. F., Napimoga, M. H. (2014). Osteoinductive potential of allogeneic human bone blocks in vitro. Revista da Associação Paulista de Cirurgiões Dentistas, 69, 75–78. [Google Scholar]
15. Haugen, H. J., Lyngstadaas, S. P., Rossi, F., Perale, G. (2019). Bone grafts: Which is the ideal biomaterial? Journal of Clinical Periodontology, 46(Suppl 21), 92–102. https://doi.org/10.1111/jcpe.13058 [Google Scholar] [PubMed] [CrossRef]
16. Georgeanu, V. A., Gingu, O., Antoniac, I. V., Manolea, H. O. (2023). Current options and future perspectives on bone graft and biomaterials substitutes for bone repair, from clinical needs to advanced biomaterials research. Applied Sciences, 13, 8471. https://doi.org/10.3390/app13148471 [Google Scholar] [CrossRef]
17. Chiapasco, M., Zaniboni, M., Boisco, M. (2006). Augmentation procedures for the rehabilitation of deficient edentulous ridges with oral implants. Clinical Oral Implants Research, 17, 136–159. [Google Scholar] [PubMed]
18. Gultekin, B. A., Bedeloglu, E., Kose, T. E., Mijiritsky, E. (2016). Comparison of bone resorption rates after intraoral block bone and guided bone regeneration augmentation for the reconstruction of horizontally deficient maxillary alveolar ridges. BioMed Research International, 2016, 4987437. [Google Scholar] [PubMed]
19. Cordaro, L., Amadé, D. S., Cordaro, M. (2002). Clinical results of alveolar ridge augmentation with mandibular block bone grafts in partially edentulous patients prior to implant placement. Clinical Oral Implants Research, 13, 103–111. [Google Scholar] [PubMed]
20. Iizuka, T., Smolka, W., Hallermann, W., Mericske-Stern, R. (2004). Extensive augmentation of the alveolar ridge using autogenous calvarial split bone grafts for dental rehabilitation. Clinical Oral Implants Research, 15, 607–615. [Google Scholar] [PubMed]
21. Smolka, W., Eggensperger, N., Carollo, V., Ozdoba, C., Iizuka, T. (2006). Changes in the volume and density of calvarial split bone grafts after alveolar ridge augmentation. Clinical Oral Implants Research, 17, 149–155. [Google Scholar] [PubMed]
22. Malara, P., von Krockow, N., Niedzielska, I., Malara, B. (2022). Evaluation of the proximal tibia as a donor site of cancellous bone for intraoral grafting procedures—A retrospective study. Journal of Clinical Medicine, 11(6), 1493. https://doi.org/10.3390/jcm11061493 [Google Scholar] [PubMed] [CrossRef]
23. Yao, F., Seed, C., Farrugia, A., Morgan, D., Cordner, S. et al. (2022). Supercritical CO2 processed bone allografts in implantology treatment. Biomedical Journal of Scientific & Technical Research, 44(2), 35428–35434. [Google Scholar]
24. Liu, H. L., Chen, Y. H., Chung, C. H., Wu, G. J., Tsao, C. H. et al. (2023). Risk of secondary malignancies in hematopoietic stem cell transplantation recipients: A nationwide population-based study in Taiwan. Balkan Medical Journal, 40, 131–138. https://doi.org/10.4274/balkanmedj.galenos.2023.2022-10-56 [Google Scholar] [PubMed] [CrossRef]
25. Chiodo, C. P., Hahne, J., Wilson, M. G., Glowacki, J. (2010). Histological differences in iliac and tibial bone graft. Foot & Ankle International, 31, 418–422. https://doi.org/10.3113/FAI.2010.0418 [Google Scholar] [PubMed] [CrossRef]
26. Mahato, N. K. (2011). Characterization of cortico-cancellous bone along the iliac crest: Focus on graft harvesting. Surg Radiol Anat, 33(5), 433–437. https://doi.org/10.1007/s00276-010-0752-z [Google Scholar] [PubMed] [CrossRef]
27. Oh, J. K., Choi, B. J., Lee, B. S. (2009). The histologic study of bone healing after horizontal ridge augmentation using auto block bone graft. Journal of Korean Association of Maxillofacial and Plastic Reconstructive Surgery, 31, 207–215. [Google Scholar]
28. Borgonovo, A. E., Tommasi, F., Panigalli, A., Bianchi, A. C., Boninsegna, R. et al. (2023). The clinical efficacy of the allogenic demineralized dentin matrix graft for implant placement: A systematic review. Oral Maxillofacial Surgery. https://doi.org/10.1007/s10006-023-01170-6 [Google Scholar] [PubMed] [CrossRef]
29. Procopio, O., Trojan, D., Frigo, A. C., Paolin, A. (2019). Use of homologous bone for alveolar crest reconstruction in 483 patients with 5 years’ outcomes post implantation. Oral and Maxillofacial Surgery, 23(3), 353–363. https://doi.org/10.1007/s10006-019-00781-2 [Google Scholar] [PubMed] [CrossRef]
30. Gaudet, C., Solecki, L., Mathéaud, B., Odet, S., Meyer, C. et al. (2022). Questions about residual cell viability in cryopreserved human amniotic membrane and its impact on clinical applications. Biomedicines, 10(10), 2456. https://doi.org/10.3390/biomedicines10102456 [Google Scholar] [PubMed] [CrossRef]
31. Gulinelli, J. L., Dutra, R. A., Marão, H. F., Simeão, S. F. P., Klein, G. B. G. et al. (2017). Maxilla reconstruction with autogenous bone block grafts: Computed tomography evaluation and implant survival in a 5-year retrospective study. International Journal of Oral and Maxillofacial Surgery, 46, 1045–1051. [Google Scholar] [PubMed]
32. Waasdorp, J., Reynolds, M. A. (2010). Allogeneic bone onlay grafts for alveolar ridge augmentation: A systematic review. International Journal of Oral and Maxillofacial Implants, 25, 525–531. [Google Scholar] [PubMed]
33. Nguyen, T. T. H., Eo, M. Y., Kuk, T. S., Myoung, H., Kim, S. M. (2019). Rehabilitation of atrophic jaw using iliac onlay bone graft combined with dental implants. International Journal of Implant Dentistry, 5, 11. https://doi.org/10.1186/s40729-019-0163-9 [Google Scholar] [PubMed] [CrossRef]
34. Deluiz, D., Oliveira, L. S., Pires, F. R., Tinoco, E. M. (2015). Time-dependent changes in fresh-frozen bone block grafts: Tomographic, histologic, and histomorphometric findings. Clinical Implant Dental Related Research, 17(2), 296–306. https://doi.org/10.1111/cid.12108 [Google Scholar] [PubMed] [CrossRef]
35. Kloss, F. R., Offermanns, V., Kloss-Brandstätter, A. (2018). Comparison of allogeneic and autogenous bone grafts for augmentation of alveolar ridge defects—A 12-month retrospective radiographic evaluation. Clinical Oral Implants Research, 29, 1163–1175. [Google Scholar] [PubMed]
36. Nilius, M., Nilius, M. H., Mueller, C., Weiland, B., Haim, D. et al. (2022). Customized allogeneic bone augmentation improves esthetic outcome in anteromaxillary dental implantation. Case Report in Dentistry, 2022, 6943930. https://doi.org/10.1155/2022/6943930 [Google Scholar] [PubMed] [CrossRef]
37. Tallarico, M., Park, C. J., Lumbau, A. I., Annucci, M., Baldoni, E. et al. (2020). Customized 3D-printed titanium mesh developed to regenerate a complex bone defect in the aesthetic zone: A case report approached with a fully digital workflow. Materials, 13(17), 3874. https://doi.org/10.3390/ma13173874 [Google Scholar] [CrossRef]
38. Nilius, M., Mueller, C., Nilius, M. H., Haim, D., Weiland, B. et al. (2021). Advanced backward planning with custom-milled individual allogeneic block augmentation for maxillary full-arch osteoplasty and dental implantation: A 3-year-follow-up. Cell and Tissue Banking, 23(3). https://doi.org/10.1007/s10561-021-09947-3 [Google Scholar] [PubMed] [CrossRef]
39. de Almeida Malzoni, C. M., Gonçalves, V., Possari, J., Marcantonio, E. (2022). The use of 3D-ceramic block graft compared with autogenous block graft for rehabilitation of the atrophic maxilla: A randomized controlled clinical trial. Research Square. https://doi.org/10.21203/rs.3.rs-1768533/v1 [Google Scholar] [CrossRef]
40. Osman, R. B., Swain, M. V. (2015). A critical review of dental implant materials with an emphasis on titanium versus zirconia. Materials, 8, 932–958. https://doi.org/10.3390/ma8030932 [Google Scholar] [PubMed] [CrossRef]
41. Bohner, M., Baroud, G., Bernstein, A., Döbelin, N., Galea, L. et al. (2017). Characterization and distribution of mechanically competent mineralized tissue in micropores of β-tricalcium phosphate bone substitutes. Materials Today, 20, 106–115. https://doi.org/10.1016/j.mattod.2017.02.002 [Google Scholar] [CrossRef]
42. Ghassemi, T., Shahroodi, A., Ebrahimzadeh, M. H., Mousavian, A., Movaffagh, J. et al. (2018). Current concepts in scaffolding for bone tissue engineering. The Archives of Bone and Joint Surgery, 6(2), 90–99. [Google Scholar] [PubMed]
43. Yu, Q., DiFeo Jacquet, R., Landis, W. J. (2020). Characterization of tissue-engineered human periosteum and allograft bone constructs: The potential of periosteum in bone regenerative medicine. Cells Tissues Organs, 209(2–3), 128–143. https://doi.org/10.1159/000509036 [Google Scholar] [PubMed] [CrossRef]
44. Genova, T., Petrillo, S., Zicola, E., Roato, I., Ferracini, R. et al. (2019). The crosstalk between osteodifferentiating stem cells and endothelial cells promotes angiogenesis and bone formation. Frontiers Physiology, 10, 1291. https://doi.org/10.3389/fphys.2019.01291 [Google Scholar] [PubMed] [CrossRef]
45. Buffoli, B., Boninsegna, R., Rezzani, R., Poli, P. P., Santoro, F. et al. (2013). Histomorphometrical evaluation of fresh frozen bone allografts for alveolar bone reconstruction: Preliminary cases comparing femoral head with iliac crest grafts. Clinical Implant Dental Related Research, 15(6), 791–798. https://doi.org/10.1111/cid.12028 [Google Scholar] [PubMed] [CrossRef]
46. Sendyk, W. R., Querido, M. R. M., Silva, C. E. X. S. (2010). Avaliação histológica da reparação de enxertos ósseos autógenos e alógenos frescos congelados em coelhos (Brazilian Edition). ImplantNews, 7, 27–34. [Google Scholar]
47. Caregnato, L., Ponzoni, D., Matsumoto, M. A., Carneiro, G. P., Bertonha, M. (2010). Levantamento de seio maxilar usando substitutos ósseos. Relato de caso e análise histológica após sete meses (Brazilian Edition). ImplantNews, 7(4), 473–480. [Google Scholar]
48. Mallmann, F., Manfro, R., Bortoluzzi, M. C., Ruchell, G. H., Weiter, J. F. et al. (2012). Estudo com desenho split-mouth com enxertos onlay de bloco alógeno na maxila para futura colocação de implantes osseointegrados; resultados tomográficos e histológicos (Brazilian Edition). ImplantNews, 9(2), 233–239. [Google Scholar]
Cite This Article
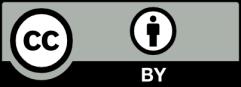
This work is licensed under a Creative Commons Attribution 4.0 International License , which permits unrestricted use, distribution, and reproduction in any medium, provided the original work is properly cited.