Open Access
ARTICLE
Rheological and Morphological Characterization of Modified Bitumen with Cup Lump Rubber
1
Department of Civil and Environmental Engineering, College of Engineering, Majmaah University, Al-Majmaah, 11952, Saudi Arabia
2
Department of Civil Engineering, Kebbi State University of Science and Technology, Aliero, Kebbi, Nigeria
3
School of Civil Engineering, Universiti Teknologi Malaysia, Johor Bahru, 81310, Malaysia
4
Department of Civil Engineering, College of Engineering, Jouf University, Sakaka, 72388, Saudi Arabia
5
Engineering Management Department, College of Engineering, Prince Sultan University, Riyadh, 11586, Saudi Arabia
6
Department of Civil Engineering, Bayero University, Kano, PMB 3011, Nigeria
* Corresponding Author: Hani Alanazi. Email:
(This article belongs to the Special Issue: Current Advances in Green Nanomaterials Applications)
Journal of Renewable Materials 2023, 11(5), 2433-2451. https://doi.org/10.32604/jrm.2023.026751
Received 23 September 2022; Accepted 24 November 2022; Issue published 13 February 2023
Abstract
Pure bitumen is not suitable for heavy traffic loads; hence modifiers are used to improve the bitumen performance. Recently, cup lump rubber (CLR) has become a preferred modifier due to its outstanding performance and less cost. However, little is known about the interactions between CLR and bitumen. Thus, this study investigates the behavior of bitumen with CLR. Four percentages of CLR (2.5%, 5.0%, 7.5%, and 10.0% by weight of bitumen) were used to modify conventional 60/70 penetration grade bitumen. The modified bitumen was evaluated through different laboratory testing such as dynamic shear rheometer, rotational viscosity, softening point, bending beam rheometer, ductility, and elastic recovery. The testing results show that the addition of CLR increased the bitumen’s rutting resistance by 3 PG grades at high temperatures. At low pavement temperatures, the cup lump rubber modified bitumen (CMB) can withstand up to −34°C. Fourier Transform Infrared (FTIR) analysis shows that the Aromaticity index at 1600 cm-1 rose as the CLR percentage increased, indicating the formation of a binder with a compact structure. This is expected to improve the elasticity of bitumen through π – π interactions. Atomic Force Microscopy (AFM) results showed the Catana phase increased in size and quantity at 5.0% and 7.5% CLR content. While contact angle measurement revealed that the binders are hydrophobic and tend to repel the dropped water on the bitumen surface.Keywords
To address the primary reasons for asphalt pavement degradation, the road construction industry employs polymer as a bitumen modifier. It is widely recognized that conventional bitumen cannot handle significant traffic loads, especially in harsh environmental conditions. As a result, pavements often collapse before reaching their designed life [1]. Consequently, typical pavement distress, which includes bottom-up fatigue cracking, rutting, cracking at low temperatures, and stripping failure, occurred. Utilizing polymer-modified bitumen (PMB) has several benefits, including less temperature sensitivity, a greater elastic recovery rate, a higher softening point value, and improved cohesive strength. Thus, PMB is a crucial component of the pavement construction process [2,3]. Polybutadiene, butadiene styrene (SBS), ethylene-vinyl acetate (EVA), polyvinyl acetate (PVA), styrene-butadiene rubber (SBR), crumb rubber, and natural rubber (NR) are examples of polymers being utilized to enhance bitumen performance [4,5].
NR is considered renewable and cost-effective compared to synthetic polymer, especially in countries with a high rate of rubber production [6,7]. The use of NR as a bitumen modifier increases the rutting resistance, fatigue life, dynamic stability, moisture damage, and low-temperature cracking resistance [8,9]. Furthermore, due to its structural regularity, NR can discharge high tire pressure to avoid formation of rutting or fatigue cracking on the pavement. NR latex, cup lump rubber (CLR), and ribbed smoked sheet (RSS) are common types of NR used in bitumen modification. The drawback of using NR (latex) is that it requires a special mixing plant due to the high-water content, which generates foam in large quantities. While the use of NR in dry form (such as CLR or RSS) leads to ineffective dispersion of the rubber in bitumen and the high tendency of the rubber particles to separate from the bitumen blend under storage. Also, adding high rubber content often leads to the production of a heterogenous blend with the rubber particles acting like elastic filler. But research has now overcome this drawback by adding toluene, xylene, nano-alumina or Polyphosphoric acid to aid in the effective dispersion of the particles and to produce a homogenous blend [10].
Cup lump rubber (CLR) is a freshly coagulated NR that underwent no manufacturing processes [11]. To boost the price of NR through pavement construction, the Malaysian road authority constructed some road sections with cup lump modified asphalt (CMA) in 2015. The pavement section was monitored for two years. The obtained results indicate improvement in resilience, durability, permanent deformation, and thermal and fatigue cracking failure resistance compared to the conventional mixture [12]. This led to the construction of an additional 1000 km of CMA roads in 2018 [13], and the project consumed about 42,000 tons of CLR [14].
Despite the huge success recorded in the project, information on the rheological and morphological properties of CLR-modified bitumen is not fully revealed. Hence, investigating the binder properties will aid the adoption of CLR in road construction globally. Also, because of the complicated interactions that exist between bitumen and CLR, characterizing the binders first is crucial before applying them at the mixed design level [15]. Notably, an attempt was made to produce and examine the binder properties of cup lump rubber-modified bitumen. However, only the physical properties of the binder were reported, which is insufficient to fully characterize the novel bitumen [16]. Similarly, Ansari et al. [17] extensively analyzed the physical, rheological, and chemical properties of CLR modified bitumen with 0.5% Polyphosphoric acid (PPA). However, the literature placed more emphasis on the effect of PPA in reducing the instability and phase separation of the modifier at high temperatures. Thus, the present study aims to examine bitumen’s physical, rheological, and morphological properties incorporating CLR through different laboratory testing at low, intermediate, and high temperatures. The outcome of the current research shall provide better knowledge about the effect of bitumen modification with a less costly material and promote CLR to the asphalt industry.
A simplified schematic interaction between CLR and bitumen is presented in Fig. 1. First, CLR particles are made into contact with bitumen under constant heat and stirring. The interaction begins when the CLR particles absorb light oil fractions from the bitumen and swell, forming a gel layer at the bitumen-CLR interface. The swelling continues, and chemical degradation of the CLR particles occurs by splitting into smaller fractions due to the destruction of the polymer chain structure. The CLR particles continue to degrade until they are fully dissolved into the bitumen matrix, producing a homogeneous binder as the final product.
Figure 1: Simplified schematic of interaction between CLR particles and base bitumen
The possibility of overlap between the various interaction stages discussed above is worth mentioning. The rubber network’s deterioration makes it easier for light bitumen components to be absorbed by the remaining rubber, causing swelling. In turn, the swelling also quickens the rate at which rubber deteriorates. There is a gradient of swelling rubber from the exterior to the interior. Numerous variables, including temperature, mixing duration, the bitumen’s chemical components, and the type and size of the rubber particles, were found to influence the rate of rubber swelling. A higher level of interaction between bitumen and rubber can be increased with enough heat and time to result in the depolymerization of the rubber particles. The rubber particles can swell up to three to five times their original size. Factors such as the temperature and time of rubber-bitumen contact, the chemical composition of bitumen, rubber type and size were all found to affect the rate of rubber swelling. In addition, small rubber particles can swell three to five times their original size on reacting with bitumen [18,19].
The materials used in this study are 60/70 penetration grade bitumen and Natural rubber in the form of cup lump. Cup lump rubber was obtained from a rubber factory in Johor Malaysia. The CLR has moisture content of 5.2%. Gas displacement pycnometer equipment (AccuPyc II 1340 V2.01) determined that the CLR has a specific gravity of 0.94. The CLR sheet as obtained from the factory is displayed in Appendix A.
First, CLR was cut into smaller sizes (passing 10 mm sieve) from the CLR sheet before soaking in Toluene solvent for one day using the ratio of 2:1 (Toluene:CLR). The soaking process changed the CLR into gel to aid in the rubber’s proper dispersion in the bitumen during blending. The CLR pre-treatment process is presented as Appendix B. A total of Five (5) types of bitumen were prepared and used in this study. Using a high shear mixer, bitumen is first mixed with 0, 2.5, 5.0, 7.5, and 10.0 percent by mass of bitumen at 4000 revolution per minute for two hours at 60°C ± 10°C [20]. A storage stability test was conducted to examine the likelihood of separation between the base bitumen (BB) and the CLR. The outcome shows that the bitumen with 10% CLR failed this test and was discarded from the study. Hence only the BB (0%) and the bitumen with 2.5%, 5.0%, 7.5% CLR were examined further.
In this study, physical, rheological, and morphological tests were carried out to examine the produced modified bitumen at various pavement temperatures (high, intermediate, and low). For each test conducted in this study, at least three replicants were prepared and tested. The average value is reported, the error bar is provided to display the disparity of the measured data.
The asphalt penetration test was performed according to ASTM D5 standard [21]. In line with ASTM D36, a softening point test was conducted to ascertain the temperature at which the binders transitioned from a semi-solid to a semi-liquid state [20]. The ductility test was conducted by drawing a bitumen sample from a briquette mold at the rate of 5 cm/min in the water bath at a temperature of 25°C ± 5°C as specified in ASTM D6084 standard [22]. The total length that the material extends before failing is recorded as the ductility value. The ductility test only covers the bitumen’s extension capacity without taking care of the bitumen’s recovery ability. As a result, an elastic recovery test was conducted to ascertain the binders’ ability to recover after deforming.
To make sure that the CLR modified binders can be easily mixed at production and construction temperatures, a viscosity test was performed. Test specimens were produced per ASTM 4402 standard, and Brookfield’s rotational viscometer (DV-II + Pro) was used to conduct the test. In this test, about 11g of liquid bitumen is poured into an aluminum mould (of about 20 mm diameter). The mould with the bitumen sample is then placed in a preheated chamber of the viscosity test apparatus and maintained at the desired testing temperature for 15–30 min to reach equilibrium. The motor rotation of the viscometer is started at 20 revolutions per minute using spindle number 27. The selected speed will develop a resisting torque between 10% to 98% of the full capacity of the (DV-II + Pro) viscometer apparatus. The viscosity of the bitumen displayed on the screen is then recorded at one-minute intervals for three minutes. The testing temperature is increased, and the above process is repeated [23].
At different pavement temperatures (46°C–88°C) and a loading frequency of 10 rad/s (1.59 Hz), the stiffness, viscoelastic properties, and resistance to deformation under the load of the binders were measured by conducting a dynamic shear rheometer (DSR) test. Bitumen sample is placed between two parallel plates of a HAAKE rheometer machine, and a sinusoidal strain is applied to it. The phase angle (δ) refers to the difference between the material’s response and the imposed strain, which causes corresponding sinusoidal stress to emerge as a result of the imposed strain being recorded. Other parameters obtained from this test include rutting and fatigue resistance of the binders [24].
Bitumen changes from a time-dependent ductile material to a brittle behavior when a pavement is exposed to cold temperatures, which raises the probability of crack formation [25]. As such, the creep stiffness behavior of bitumen at various low pavement temperatures is determined through the bending beam rheometer (BBR) test. The test was performed on the original binder in accordance with the ASTM6818 standard [26]. For specification purposes, the bitumen’s creep stiffness and m-value were established at a loading time of 60 s [27].
Using Attenuated Total Reflectance-Fourier Transform Infrared (ATR-FTIR) spectroscopy, the modified bitumen’s chemical properties were investigated. ATR sampling technique allows samples to be directly observed in a solid or liquid state without the need for heating, pressing into pellets or grinding before collecting the spectra as against the conventional FTIR. The bitumen sample to be tested is first positioned on the ATR prism before an infrared light of the wavelength of 4000 to 500 cm−1 is passed through it. A computer system attached to the ATR instrument records the energy absorbed or transmitted by the bitumen sample at various wavelengths, converts it into peaks, and displays it as charts of intensity vs. wavenumber [28]. The spectrum is used to analyze the oxidative aging of bitumen by measuring the amount of variation in carbonyl (C=O) and sulfoxide (S=O) absorption as well as carbon-carbon (C=C) stretching vibrations of the aromatic ring [29,30]. TQ Analyst program was used to analyze and quantify the IR spectra by computing the region bordered by each peak [31]. They include aliphatic index ((A1452 + A1373)/ΣA), aromaticity index (A1600/ΣA), carbonyl oxidation (A1700/ΣA), the presence of sulfoxide group (A1030/ΣA), aromatic heteroatoms ((A874 + A809 + A747)/ΣA), and long chains A720/(A1452+ A1373). The sum of the areas is calculated using Eq. (1).
The forces produced between the end of a cantilever beam and bitumen are quantified using atomic force microscopy (AFM) as a function of the distance between the two surfaces [32]. Appendix C presents a schematic of AFM test procedure. In this AFM test, samples were prepared by heating bitumen to a fluid state at 135°C. A bitumen of around 2 mm in diameter is poured on a microscope glass slide. The glass slide is placed in the oven at 135°C for 5 min to produce a smooth and flat testing surface [33]. During testing, the tiny cantilever tip oscillates just above its resonance frequency and analyzes the bitumen surface (non-contact mode of operation), and the system creates surface profiles of the specimen in two dimensions (2D) and three dimensions (3D). The average distance between the tip-to the sample surface is used to scan the surface. When the tip approaches the bitumen surface and feels the forces generated by the material, the frequency decreases, and a lower scan rate (0.5–1 Hz) was adopted in this study in order to give the response additional time to determine the proper height for the probe at each spot on the bitumen sample. Also, the tracking was done with a large setpoint of 6V (less force) to avoid causing damage to the bitumen sample or dulling the probe. The 3D image offers detail on the comparative height of the several topographies present in the specimen, while the 2D image illustrates the numerous phase matrices contained in the tested bitumen [34].
Bitumen modification with CLR can alter its adhesion properties. As such, investigating the CMB’s propensity to attract moisture at the bitumen-aggregate interface, which in turn lowers the adhesion bond, becomes necessary [35,36]. In order to prepare samples for this test, bitumen was heated in an oven until it was fluid enough to pour. The bitumen is applied to a 3 cm by 5 cm region of a microscope glass slide. At room temperature, a tiny syringe needle and a sessile drop of distilled water are discharged from a height of 5 mm. A picture of the sessile drop was recorded with a video contact angle measuring device (VCA optima), and the left, and right contact angles were calculated. The contact angle test set-up is depicted in Appendix D. For each type of bitumen produced in this study. Five replicate samples were prepared and examined. The repeatability of the measurement was estimated using a single test method. The results show that the variability ranges from 1.38–2.59, which is within the recommended limit of ±5 degrees [37,38].
Field electron scanning microscopy (FESEM) was conducted to investigate the morphology of the CLR, and the result is presented in Fig. 2. CLR has a porous surface with the agglomeration of rubber globules classified as short fibres. The fiber comes from impurities present in the NR especially sand (SiO2) and other remnants from the NR tree during tapping [39].
Figure 2: Field electron scanning microscopy image of cup lump rubber at 50 μm
3.1 Penetration and Softening Point
The results of the penetration and softening point tests are shown in Fig. 3. It demonstrates that CLR modification significantly increased the hardness of the BB (0% CLR), as observed in the decreasing penetration values with increasing rubber content. This increasing hardness was equally noticed in the rising softening point temperature of the binders. This is explained by swelling the rubber particles in the BB, which causes a tougher matrix to be formed. A similar effect was reported in the following literature [5,7] and Azahar et al. [39] on modified bitumen with other types of NR. The outcome of these consistency tests agrees with the concept that CLR addition causes binder stiffening. When CLR and BB are blended together, the rubber absorbs small amounts of the bitumen’s light oils, causing the rubber particles to swell and create a gel-like structure around the bitumen-rubber interface. When the volume of the binder increases due to the swelling action, the softening temperature also rises until an equilibrium is established [40].
Figure 3: Penetration values and softening point for bitumen modified with cup lump rubber
3.2 Ductility and Elastic Recovery
The results of the ductility and elastic recovery tests are shown in Fig. 4. It indicates that adding 2.5% and 5.0% CLR marginally reduced the ductility value, whereas 7.5% CLR led to a severe reduction of the ductility value of the bitumen. At 7.5% CLR content, it was observed that the bitumen did not form a homogenous thin thread before breaking at 43 cm, indicating the heterogeneity of the bitumen sample caused by the undissolved rubber particles in suspension. NR can enhance the stiffness of bitumen, thereby improving its performance at high levels of temperature but making it more brittle at intermediate temperatures (25°C) [41]. This finding suggests that adding a higher percentage of CLR may negatively affect the cohesion of bitumen or that the ductility test is unsuitable for measuring the rubberized bitumen’s stretching ability. Contrarily, it was discovered that the addition of rubber had a considerable favorable impact on the elastic recovery of bitumen as seen in the chart, where elastic recovery increased as CLR content increased. The addition of CLR by percentages of 5.0 and 7.5 boosted and improved the elastic recovery of the BB by more than 100%. Though none of the evaluated binders met the recommendation that a minimum of 60% elastic recovery should be present in a binder [42].
Figure 4: Effect of CLR addition on ductility and elastic recovery property of bitumen
Fig. 5 presents the viscosity test results. It shows that adding 2.5 percent, CLR substantially increased the viscosity of the BB. At CLR content levels of 5.0 and 7.5 percent, similar stiffness increase was seen. Despite the increase, the CMB’s viscosity is within the ASTM specification limit of less than 3 Pa.s at 135°C. Upon increasing the testing temperature, the binders’ viscosity reduced in direct proportion to a rise in testing temperature to 165°C. This is because bitumen has a viscoelastic property, where temperature and shear rate both have a substantial impact on its viscosity [43]. The bitumen’s rheology typically changes when the temperature rises from 135°C to 165°C, and it begins to flow, but the CLR acts like a polymer chain and prevents the binders from flowing, thereby increasing the bitumen’s shear resistance [7]. Additionally, as NR has a melting temperature of 180°C, which is higher than that of the BB, hence the addition of CLR is anticipated to slightly raise the melting point of the BB. Also, CLR addition shall improve the rheology of bitumen by reducing its sensitivity to temperature consequently improving its rutting resistance.
Figure 5: Significance of CLR addition on bitumen viscosity at 135°C and 165°C
Although the qualities of the asphalt mixture have the greatest impact on rutting, the bitumen behavior also plays a significant role. Bitumen is a viscoelastic substance whose rheological characteristics are affected by temperature and time. Unmodified bitumen shows Newtonian behavior at temperatures above 60°C, while polymer-modified bitumen exhibit shear susceptibility at the same temperature. In this study, the non-recoverable deformation of the modified binders was measured using isochronal plots of complex modulus at 0.1 s loading time (equal to 1.59 Hz), simulating a truck tire traveling at 80 km/h on the pavement. To determine the binder’s resistance to rutting, a limiting value of |G*|/sin(δ) = 1 kPa was utilized, and the result is shown in Fig. 6. It demonstrates that the BB has the lowest resistance to rutting (70°C) when compared to the CLR-modified bitumen. The rutting resistance of the 2.5 and 5.0 percent CLR-modified bitumen is 82°C, whereas the 7.5 percent CLR-modified bitumen has a rutting resistance of 88°C. Thus, CLR significantly improved BB’s rutting resistance by 3 PG grades at a CLR level of 7.5 percent.
Figure 6: Isochronal of phase angle and rutting resistance at a fixed frequency of 1.59 Hz
The phase angle curves show that at low CLR content (2.5%), the performance of the CMB is comparable to the BB. While the polymer network in the 7.5 percent CMB caused the phase angle (δ) readings to decrease even more at temperatures below 60°C due to the high rubber content, signifying an enhanced elastic response. Additionally, the phase angle of the BB reaches 90° at temperatures above 70°C, indicating mostly viscous behavior, whereas the phase angle of the CMB is around 75°. Also, the development of a plateau region proves the presence of a dominating polymer network and confirms that the inclusion of CLR improved the modified binders’ elastic response at high temperatures. The improvement is ascribed to the BB’s low viscosity, which allowed the modified bitumen’s characteristics to be affected by the elastic network of the polymer.
Moreover, the equivalence of time and temperature dependence of bitumen using master curves is typically related using the time-temperature superposition principle (TTSP). This is accomplished by gathering rheological data at various frequencies and temperatures, selecting a standard reference temperature, then shifting the data at other temperatures andime until a smooth curve is generated [30]. TTSP is expressed as Eq. (2).
where G* is the modulus, ω is the loading frequency, T is the temperature, and Tr is the reference temperature.
It should be noted that the master curves are only accurate at the selected standard reference temperature used in plotting the curves, thus limiting the use of TTSP to generate rheological data at another temperature. In addition, when interpreting the master curve and assessing the rheological characteristics of polymer-modified bitumen, one must consider both the time and temperature dependency as indicated by the shift factors. As such, isochronal plots provide more information and can be used to interpret the rheological characteristics of bitumen [44] easily.
Fig. 7 presents the BBR test results. Creep stiffness (S) measures how stiff the bitumen is at low temperatures, while m-value measures the binder’s capacity to release applied stress by plastic flow swiftly. The bitumen should be less stiff (poses a lower creep stiffness value) and have a higher m-value (fast rate of stress release) for better low-temperature performance [44]. The creep stiffness of the BB and the CLR modified bitumen is noticeably different at −12°C testing temperature, according to a visual assessment of the bar chart. It demonstrates that the BB has a larger beam deflection value and a lower flexural rigidity. When bitumen was modified with 2.5 and 5.0 percent CLR, the BB’s creep stiffness increased by 13 and 17 percent, respectively, whereas the creep stiffness decreased at 7.5 percent CLR content. Improvement in the elasticity of bitumen is indicated by a decrease in creep stiffness and is expected to resist the formation of microcracks at low pavement temperatures. It also implies that a small percentage of CLR replacement is inadequate for reducing the stiffness of the bitumen at low testing temperatures. Wen obtained a similar result, where only bitumen modified with more than 9% NR latex showed improvement in thermal cracking resistance. Given the fact when bitumen is exposed to freezing temperatures, it becomes too stiff and tends to crack under stress, NR acts as an elastic band by holding the bitumen together and helps in dissipating the stresses as they develop [9]. At a temperature of −12°C, all of the tested binders met the AASHTO criteria of S ≤ 300 MPa and m-value ≥ 0.3, indicating the necessity for more investigation at considerably lower temperatures. All the binders show greater creep stiffness when the testing temperature is raised to −18°C, but the BB has a higher rate of stiffness increase when compared with the CLR modified bitumen, hence becoming extra brittle. This was supported by the deflection data, which showed a severe 53 percent reduction within a −6°C temperature increase, the m-value values showed a similar tendency. At −24°C, the BB and the 2.5 percent CLR both display the same creep stiffness value. However, the 7.5 percent CLR displayed a considerably lower creep stiffness value than the BB. The m-value of the CLR modified bitumen has reached the 0.3 limits at −24°C, even though the stiffness value of all the tested binders is still below the 300 MPa limit. This shows that the binder’s maximum capacity for reducing stress has been reached. As a result, the binder is graded as PGXX−34°C in line with the AASHTO M320 specification.
Figure 7: Low-temperature cracking resistance of CLR modified bitumen
3.6 Fourier Transform Infrared (FTIR)
The variations in the infrared spectra of the CLR modified bitumen are depicted in (Appendix E(a)) and Table 1. Additionally, the FTIR of CLR alone and the BB is presented (Appendix E(b)) to show specific footprint and clarity. For the CLR alone, substantial out-of-plane absorption from the toluene group was found at 619–775 cm−1, and cis 1.4–polyisoprene absorption associated with =C–H out of plane bending was identified at 842 cm–1. Significant changes were seen in the CLR modified bitumen from 600 to 2000 cm−1 wavenumbers. First, the lack of a peak at 1700 cm−1 indicates that the CLR addition did not affect carbonyl absorption, indicating that no oxidation took place throughout the blending process. The addition of 2.5% CLR marginally increased the absorption of single bonds from 910 to 1300 cm−1, but the absorption at reduces as the CLR content increased to 5.0 and 7.5 percent, respectively. The aromaticity index at 1600 cm−1 (A1600/ΣA) increased with increasing CLR content, indicating the formation of a binder with a compact structure. This is expected to provide strong interactions and improve the elasticity of the binder through π – π interactions [29]. This is because unsaturated bonds are present in the CLR, favoring the increase in the aromatic ring with significant delocalized π-electron [45]. Furthermore, it is confirmed that the structure becomes more compact by the presence of long-chain aliphatic chemicals at 720 cm−1 and the declining aliphatic index (A1456 + A1378/A) with increasing CLR concentration [46]. The decrease in absorbance with increasing CLR content at 810, 745 and at the region between 2900–3100 cm−1 wavenumbers, which characterize C-H aromatic bonds, further supports this finding.
Fig. 8a illustrates a 50 × 50 µm scan of the bitumen that reveals a network structure with two unique phases: the Catana phase (bee-structure) and a matrix that combines the Peri and Para phases [47]. Peri and Catana phases are the high-stiffness matrices with low cohesion, whereas Para-phase has a low-stiffness matrix (with strong cohesion) [48]. In the instance of the BB, it is easy to see the Catana phase, but it is difficult to tell the difference between the Peri and Para phases. This is because bitumen source and origin determine its morphology at the microscale level. The addition of CLR begins by increasing the number and decreasing the size of the bee structure at 2.5% CLR content. Changes in the shape and size of the bee-like structure indicate changes in the molecular structure of the bitumen [49]. At CLR contents of 5.0 and 7.5 percent, a rise in quantity and size was seen. This occurs when aromatic compounds that contain bee-structure mix with asphaltenes, and the bee structure disintegrates into smaller elements, producing a conglomerate of bee structures [32]. Surprisingly, the FTIR results confirm this finding that the aromatic index increased as the CLR proportion increased. Additionally, the interaction of the polar bee structure with the more polar maltene components (aromatic and resin) results in an increase in stiffness as the CLR content increases [50]. Furthermore, the modified bitumen sample was at re-scanned at 10 × 10 µm magnification to possibly differentiate between Peri and Para phases and presented as Fig. 8b. However, only the Catana phase is distinctively observed while Peri and Para phases still acts as a combined matrix.
Figure 8: Atomic force microscope scan showing changes in Catana, Peri and Para phases at various CLR content (a) at 50 × 50 µm scan (b) at 10 × 10 µm scan
Fig. 9 presents the contact angle measurement results carried out to examine the affinity of the binders to water. Bitumen can be categorized as hydrophilic (loving water) or hydrophobic, which tends to repel water and is less susceptible to stripping [51]. The dropped distilled water forms an average angle of 93° on the BB, while the contact angles for the CMB are 103°, 104°, and 106° at 2.5, 5.0 and 7.5 percent CLR, respectively. It can be argued that all binders are hydrophobic since the measured contact angles are greater than 90°, and they tend to resist water from the bitumen surface. Additionally, the modified binders have a more non-water absorbing surface than the BB, as seen by the rise in contact angle with increasing CLR concentration. In a similar vein, the difference in polarity makes non-polar substances like bitumen and oils not allow water (polar) to spread over their surface [52,53]. It is anticipated that this lack of water wettability will strengthen the link between the bitumen and the aggregate and increase resistance to induced stripping failure. When water is allowed to wet the bitumen surface, then fewer points of contact will exist between the bitumen and the aggregate. Therefore, compared to BB, the CLR modified binders shall be more resistant to moisture degradation.
Figure 9: Contact angle measurement showing the affinity of the binders to water at various cup lump rubber content: (a) BB (b) 2.5% (c) 5.0% (d) 7.5%
This investigation assesses the characteristics of bitumen containing CLR. Including natural rubber in the form of a cup lump increased the bitumen’s viscosity and softening point temperature while the penetration value was significantly reduced. DSR test result shows significant improvement in the rutting resistance of the base bitumen by three performance grades due to the addition of CLR. Similarly, the BBR result demonstrates that CLR addition enhanced the base bitumen’s low-temperature performance. Thus, this modification has extended the base bitumen’s low and high-temperature range (plasticity interval). Furthermore, FTIR spectra confirm that oxidation does not occur during the CLR modification, but the increase in aromatic ring enriched the elasticity of the modified binders. The bee structure is dispersed in the AFM scan, while contact angle measurements showed that the addition of CLR improved the bitumen’s ability to repel water. The outcome of this study shows that 5% CLR is the optimum percentage for cup lump rubber modified bitumen production. Lastly, CLR modified bitumen can be used at the mix design level to create a durable asphalt road with less maintenance.
Acknowledgement: The authors extend their appreciation to the deputyship for Research & Innovation, Ministry of Education in Saudi Arabia for funding this research work through the Project No. (IFP-2020-89).
Funding Statement: The authors received funding for this research work through the Project No. (IFP-2020-89) from the Deputyship for Research & Innovation, Ministry of Education in Saudi Arabia.
Conflicts of Interest: The authors declare that they have no conflicts of interest to report regarding the present study.
References
1. Rodríguez-Fernández, I., Cavalli, M. C., Poulikakos, L., Bueno, M. (2020). Recyclability of asphalt mixtures with crumb rubber incorporated by dry process: A laboratory investigation. Materials, 13(12), 2870. DOI 10.3390/ma13122870. [Google Scholar] [CrossRef]
2. Shaffie, E., Ahmad, J., Arshada, A. K., Kamarun, D., Awang, H. (2016). Investigation on rutting performance of nanopolyacrylate and natural rubber latex polymer modified asphalt binder mixes. Jurnal Teknologi, 78(7–3), 10–14. DOI 10.11113/jt.v78.9469. [Google Scholar] [CrossRef]
3. Buczyński, P., Iwański, M. (2019). The influence of a polymer powder on the properties of a cold-recycled mixture with foamed bitumen. Materials, 12(24), 4244. DOI 10.3390/ma12244244. [Google Scholar] [CrossRef]
4. Škoc, M. S., Pezelj, E. (2012). Abrasion resistance of high performance fabrics. In: Abrasion resistance of materials rijeka, pp. 35–52. Croatia: InTech. [Google Scholar]
5. Shafii, M. A., Veng, C. L. Y., Rais, N. M., Ab Latif, A. (2017). Effect of blending temperature and blending time on physical properties of NRL-modified bitumen. International Journal of Applied Engineering Research, 12(13), 3844–3849. [Google Scholar]
6. Allison, K. (1967). Those amazing rubber roads. Rubber World, 78(3–4), 47–52. [Google Scholar]
7. Saowapark, W., Jubsilp, C., Rimdusit, S. (2019). Natural rubber latex-modified asphalts for pavement application: Effects of phosphoric acid and sulphur addition. Road Materials and Pavement Design, 20(1), 211–224. DOI 10.1080/14680629.2017.1378117. [Google Scholar] [CrossRef]
8. Shaffie, E., Ahmad, J., Arshad, A., Kamarun, D., Kamaruddin, F. (2015). Stripping performance and volumetric properties evaluation of hot mix asphalt (HMA) mix design using natural rubber latex polymer modified binder (NRMB). Proceedings of the International Civil and Infrastructure Enginerring, pp. 873–884. Singapore. [Google Scholar]
9. Siswanto, H. (2017). The effect of latex on permanent deformation of asphalt concrete wearing course. Procedia Engineering, 171(10), 1390–1394. DOI 10.1016/j.proeng.2017.01.452. [Google Scholar] [CrossRef]
10. Abdulrahman, S., Hainin, M. R., Satar, M. K. I. M., Hassan, N. A., Al Saffar, Z. H. (2020). Review on the potentials of natural rubber in bitumen modification. Proceedings of the 2nd International Conference on Civil & Environmental Engineering, IOP Conference Series: Earth and Environmental Science, vol. 476, 012067. Langkawi, Malaysia. [Google Scholar]
11. Tuntiworawit, N., Lavansiri, D., Phromsorn, C., Engineer, C. (2005). The modification of asphalt with natural rubber latex. Proceedings of the Eastern Asia Society for Transportation Studies, 5, 679–694. [Google Scholar]
12. Abdulrahman, S., Hainin, M. R., Satar, M. K. I. M., Hassan, N. A., Usman, A. (2021). Mechanical performance and global warming potential of unaged warm cup lump modified asphalt. Journal of Cleaner Production, 297, 126653. DOI 10.1016/j.jclepro.2021.126653. [Google Scholar] [CrossRef]
13. Huibin, O., Mohamed, S., Thierry, B., Jean-claude, G. (2016). Determination of the activation energy of silicone rubbers using different kinetic analysis methods. Proceedings of the 12th International Conference on Numerical Methods in Industrial Forming Processes, MATEC Web of Conferences, vol. 80, 16007. Troyes, France. [Google Scholar]
14. Othman, Z., Hainin, M. R., Warid, M. N. M., Idham, M. K., Kamarudin, S. N. N. (2018). Cup lump modified asphalt mixture along jalan Kuala Lumpur-Kuantan, daerah Temerloh, Pahang. The 3rd International Symposium on Expertise of Engineering Design (SEPKA-ISEEDMATEC Web of Conferences, vol. 250, 2007. Johor, Malaysia. [Google Scholar]
15. Wang, H., Liu, X., Zhang, H., Apostolidis, P., Scarpas, T. et al. (2020). Asphalt-rubber interaction and performance evaluation of rubberised asphalt binders containing non-foaming warm-mix additives. Road Materials and Pavement Design, 21(6), 1612–1633. DOI 10.1080/14680629.2018.1561380. [Google Scholar] [CrossRef]
16. Abdulrahman, S., Hainin, M., Idham, M., Hassan, N. A., Warid, M. M. et al. (2019). Physical properties of warm cup lump modified bitumen. Proceedings of the 11th International Conference on Geotechnical Engineering in Tropical Regions (GEOTROPIKAIOP Conference Series: Materials Science and Engineering, vol. 527, 12048. Kuala Lumpur, Malaysia. [Google Scholar]
17. Ansari, A. H., Jakarni, F. M., Muniandy, R., Hassim, S., Elahi, Z. et al. (2022). Effect of cup lump rubber as a sustainable bio-modifier on the properties of bitumen incorporating polyphosphoric acid. Construction and Building Materials, 323(6), 126505. DOI 10.1016/j.conbuildmat.2022.126505. [Google Scholar] [CrossRef]
18. Wang, H., Apostolidis, P., Zhu, J., Liu, X., Skarpas, A. et al. (2021). The role of thermodynamics and kinetics in rubber-bitumen systems: A theoretical overview. International Journal of Pavement Engineering, 22(14), 1785–1800. DOI 10.1080/10298436.2020.1724289. [Google Scholar] [CrossRef]
19. Peralta, E. J. F. (2009). Study of the interaction between bitumen and rubber (Ph.D. Thesis). Universidade do Minho, Portugal. [Google Scholar]
20. ASTM, D (2014). Standard test method for softening point of bitumen (ring-and-ball apparatus). West Conshohocken, PA, USA: ASTM International. [Google Scholar]
21. ASTM, D5 (2013). Standard test method for penetration of bituminous materials. USA: ASTM International. [Google Scholar]
22. ASTM, D6084 (2014). Standard test method for elastic recovery of asphalt materials by ductilometer. USA: ASTM International. [Google Scholar]
23. ASTM, D4402 (2011). Standard test method for viscosity determination using a rotational viscometer. USA: ASTM International. [Google Scholar]
24. ASTM, D7175 (2015). Standard test method for determining the rheological properties of asphalt binder using a dynamic shear rheometer. USA: ASTM International. [Google Scholar]
25. Cavalli, M. C., Zaumanis, M., Mazza, E., Partl, M. N., Poulikakos, L. D. (2018). Aging effect on rheology and cracking behaviour of reclaimed binder with bio-based rejuvenators. Journal of Cleaner Production, 189, 88–97. DOI 10.1016/j.jclepro.2018.03.305. [Google Scholar] [CrossRef]
26. ASTM, D6816 (2015). Standard practice for determining low-temperature performance grade (PG) of Asphalt binders. USA: ASTM International. [Google Scholar]
27. Hunter, R. N., Self, A., Read, J., Hobson, E. (2015). The shell bitumen handbook. London, UK: Ice Publishing. [Google Scholar]
28. Liao, D., Li, D., Zhou, S., Zhang, X., Fang, Y. (2023). Study on the hydration and physical properties of cement by M18 polycarboxylate superplasticizer modified graphene oxide. Journal of Renewable Materials, 11(2), 625–641. DOI 10.32604/jrm.2022.022501. [Google Scholar] [CrossRef]
29. Soenen, H., Redelius, P. (2014). The effect of aromatic interactions on the elasticity of bituminous binders. Rheologica Acta, 53(9), 741–754. DOI 10.1007/s00397-014-0792-0. [Google Scholar] [CrossRef]
30. Sun, Z., Yi, J., Chen, Z., Xie, S., Xu, M. et al. (2019). Chemical and rheological properties of polymer modified bitumen incorporating bio-oil derived from waste cooking oil. Materials and Structures, 52(5), 1–11. DOI 10.1617/s11527-019-1400-7. [Google Scholar] [CrossRef]
31. Lamontagne, J., Dumas, P., Mouillet, V., Kister, J. (2001). Comparison by Fourier transform infrared (FTIR) spectroscopy of different ageing techniques: Application to road bitumens. Fuel, 80(4), 483–488. DOI 10.1016/S0016-2361(00)00121-6. [Google Scholar] [CrossRef]
32. Aguiar-Moya, J. P., Salazar-Delgado, J., Bonilla-Mora, V., Rodríguez-Castro, E., Leiva-Villacorta, F. et al. (2015). Morphological analysis of bitumen phases using atomic force microscopy. Road Materials and Pavement Design, 16(sup1), 138–152. DOI 10.1080/14680629.2015.1029672. [Google Scholar] [CrossRef]
33. Al-Rawashdeh, A. S., Sargand, S. (2014). Performance assessment of a warm asphalt binder in the presence of water by using surface free energy concepts and nanoscale techniques. Journal of Materials in Civil Engineering, 26(5), 803–811. DOI 10.1061/(ASCE)MT.1943-5533.0000866. [Google Scholar] [CrossRef]
34. Pauli, A., Grimes, R., Beemer, A., Turner, T., Branthaver, J. (2011). Morphology of asphalts, asphalt fractions and model wax-doped asphalts studied by atomic force microscopy. International Journal of Pavement Engineering, 12(4), 291–309. DOI 10.1080/10298436.2011.575942. [Google Scholar] [CrossRef]
35. Bhasin, A., Little, D. N. (2009). Application of microcalorimeter to characterize adhesion between asphalt binders and aggregates. Journal of Materials in Civil Engineering, 21(6), 235–243. DOI 10.1061/(ASCE)0899-1561(2009)21:6(235). [Google Scholar] [CrossRef]
36. Borges, L. G. A., Savi, A., Teixeira, C., de Oliveira, R. P., de Camillis, M. L. F. et al. (2017). Mechanical ventilation weaning protocol improves medical adherence and results. Journal of Critical Care, 41(6 Suppl), 296–302. DOI 10.1016/j.jcrc.2017.07.014. [Google Scholar] [CrossRef]
37. Al-Assi, M., Kassem, E. (2017). Evaluation of adhesion and hysteresis friction of rubber-pavement system. Applied Sciences, 7(10), 1029. DOI 10.3390/app7101029. [Google Scholar] [CrossRef]
38. National Academies of Sciences, Engineering, and Medicine (2007). Using surface energy measurements to select materials for asphalt pavements. Washington DC, USA: National Academies Press. [Google Scholar]
39. Azahar, N., Hassan, N., Jaya, R., Hainin, M., Yusoff, N. et al. (2021). Properties of cup lump rubber modified asphalt binder. Road Materials and Pavement Design, 22(6), 1329–1349. DOI 10.1080/14680629.2019.1687007. [Google Scholar] [CrossRef]
40. Wang, H., Liu, X., Apostolidis, P., Erkens, S., Scarpas, T. (2019). Numerical investigation of rubber swelling in bitumen. Construction and Building Materials, 214(10), 506–515. DOI 10.1016/j.conbuildmat.2019.04.144. [Google Scholar] [CrossRef]
41. Yousefi, A. A. (2004). Rubber-polyethylene modified bitumens. Iranian Polymer Journal, 13(2), 101–112. [Google Scholar]
42. Al-Shamsi, K., Hassan, H. F., Mohammed, L. N. (2017). Effect of low VMA in hot mix asphalt on load-related cracking resistance. Construction and Building Materials, 149(1), 386–394. DOI 10.1016/j.conbuildmat.2017.05.120. [Google Scholar] [CrossRef]
43. Partl, M. (2018). Towards improved testing of modern asphalt pavements. Materials and Structures, 51(6), 1–12. DOI 10.1617/s11527-018-1286-9. [Google Scholar] [CrossRef]
44. ASTM, D6648 (2014). Standard test method for determining the flexural creep stiffness of asphalt binder using the Bending Beam Rheometer (BBR). USA: ASTM International. [Google Scholar]
45. Mouazen, M., Poulesquen, A., Bart, F., Masson, J., Charlot, M. et al. (2013). Rheological, structural and chemical evolution of bitumen under gamma irradiation. Fuel Processing Technology, 114, 144–1453. DOI 10.1016/j.fuproc.2013.03.039. [Google Scholar] [CrossRef]
46. Mouazen, M., Poulesquen, A., Vergnes, B. (2011). Influence of thermomechanical history on chemical and rheological behavior of bitumen. Energy & Fuels, 25(10), 4614–4621. DOI 10.1021/ef201000j. [Google Scholar] [CrossRef]
47. De Moraes, M., Pereira, R., Simão, R., Leite, L. (2010). High temperature AFM study of CAP 30/45 pen grade bitumen. Journal of Microscopy, 239(1), 46–53. DOI 10.1111/j.1365-2818.2009.03354.x. [Google Scholar] [CrossRef]
48. Arshad, A. K., Samsudin, M. S., Ahmad, J., Masri, K. A. (2016). Microstructure of nanosilica modified binder by atomic force microscopy. Jurnal Teknologi, 78(7–3), 37–44. DOI 10.11113/jt.v78.9480. [Google Scholar] [CrossRef]
49. Magonov, S., Alexander, J., Surtchev, M., Hung, A. M., Fini, E. H. (2017). Compositional mapping of bitumen using local electrostatic force interactions in atomic force microscopy. Journal of Microscopy, 265(2), 196–206. DOI 10.1111/jmi.12475. [Google Scholar] [CrossRef]
50. Aguiar-Moya, J. P., Salazar-Delgado, J., García, A., Baldi-Sevilla, A., Bonilla-Mora, V. et al. (2017). Effect of ageing on micromechanical properties of bitumen by means of atomic force microscopy. Road Materials and Pavement Design, 18(sup2), 203–215. DOI 10.1080/14680629.2017.1304249. [Google Scholar] [CrossRef]
51. Kakar, M. R., Hamzah, M. O., Akhtar, M. N., Woodward, D. (2016). Surface free energy and moisture susceptibility evaluation of asphalt binders modified with surfactant-based chemical additive. Journal of Cleaner Production, 112(1), 2342–2353. DOI 10.1016/j.jclepro.2015.10.101. [Google Scholar] [CrossRef]
52. Hussein, A. A., Jaya, R. P., Hassan, N. A., Yaacob, H., Huseien, G. F. et al. (2017). Performance of nanoceramic powder on the chemical and physical properties of bitumen. Construction and Building Materials, 156(4), 496–505. DOI 10.1016/j.conbuildmat.2017.09.014. [Google Scholar] [CrossRef]
53. Hussein, A. A., Putra Jaya, R., Yaacob, H., Abdul Hassan, N., Oleiwi Aletba, S. R. et al. (2021). Physical, chemical and morphology characterisation of nano ceramic powder as bitumen modification. International Journal of Pavement Engineering, 22(7), 858–871. DOI 10.1080/10298436.2019.1650277. [Google Scholar] [CrossRef]
54. Barron, A. R. (2015). Atomic Force Microscopy (AFM). Physical methods in chemistry and nano science, 1st edition. Rice University, Texas, USA: LibreTexts Chemistry. [Google Scholar]
Appendix
Appendix A: A sample of the Cup lump rubber sheet used in the study
Appendix B: Cup lump rubber pre-treatment process (a) Sliced cup lump rubber (b) Cup lump rubber + Toluene (c) Cup lump rubber gel ready for blending with bitumen
Appendix C: Atomic force microscopy (AFM) schematic and modes of operation (a) Simple schematic of AFM apparatus (b) Contact mode schematic interaction. Where (F) is the force, (k) is the spring constant, and (x) is the cantilever deflection in equation (c) Tapping mode schematic interaction (d) Non-contact mode schematic interaction [54]
Appendix D: Contact angle test set-up
Appendix E: Fourier Transform Infrared spectra of (a) combined graph for all the tested binders in the study (b) base bitumen and cup lump rubber alone
Cite This Article
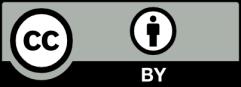
This work is licensed under a Creative Commons Attribution 4.0 International License , which permits unrestricted use, distribution, and reproduction in any medium, provided the original work is properly cited.