Open Access
ARTICLE
Chicken Eggshell as an Innovative Bioflocculant in Harvesting Biofloc for Aquaculture Wastewater Treatment
1
Faculty of Ocean Engineering Technology and Informatics, Universiti Malaysia Terengganu, Kuala Nerus, Terengganu, 21030,
Malaysia
2
Institute of Tropical Aquaculture and Fisheries (AKUATROP), Universiti Malaysia Terengganu, Kuala Nerus, Terengganu, 21030,
Malaysia
3
Faculty of Engineering, Universiti Putra Malaysia, UPM Serdang, Selangor Darul Ehsan, 43400, Malaysia
* Corresponding Authors: Nor Azman Kasan. Email: ; Ahmad Jusoh. Email:
Journal of Renewable Materials 2023, 11(5), 2321-2332. https://doi.org/10.32604/jrm.2023.026086
Received 15 August 2022; Accepted 17 November 2022; Issue published 13 February 2023
Abstract
Implementation of biofloc technology (BFT) system in aquaculture industry shows high productivity, low feed conversion ratio, and an optimum culture environment. This study was divided into two phases. The first phase involved maintaining the water quality using the optimum carbon-to-nitrogen ratio by manipulating pH in culture water. The second phase examined the performance of harvesting biofloc (remaining phytoplankton and suspended solids in the system) using chicken eggshell powder (CESP). This study showed that pH 7 to 8 were the best biofloc performance with high removal percentage of ammonia (>99%) with a remaining ammonia concentration of 0.016 mg L−1 and 0.018 mg L−1 , respectively. The second phase of this study was performed to determine the optimal formulation and conditions of using CESP as a bio-flocculant in harvesting excess biofloc. The use of eggshell showed a higher harvesting efficiency of more than 80% under the following treatment conditions: 0.25 g L−1 of eggshell dosage; with rapid and slow mixing rates of 150 and 30 rpm, respectively; 30 min of settling time; settling velocity of 0.39 mm s−1 and pH of 6 to 7. Therefore, the results indicated that biofloc would be the best green technology approach for sustainable aquaculture wastewater and the CESP is an organic matrix that environmental-friendly bio-coagulant for biofloc harvesting.Graphical Abstract
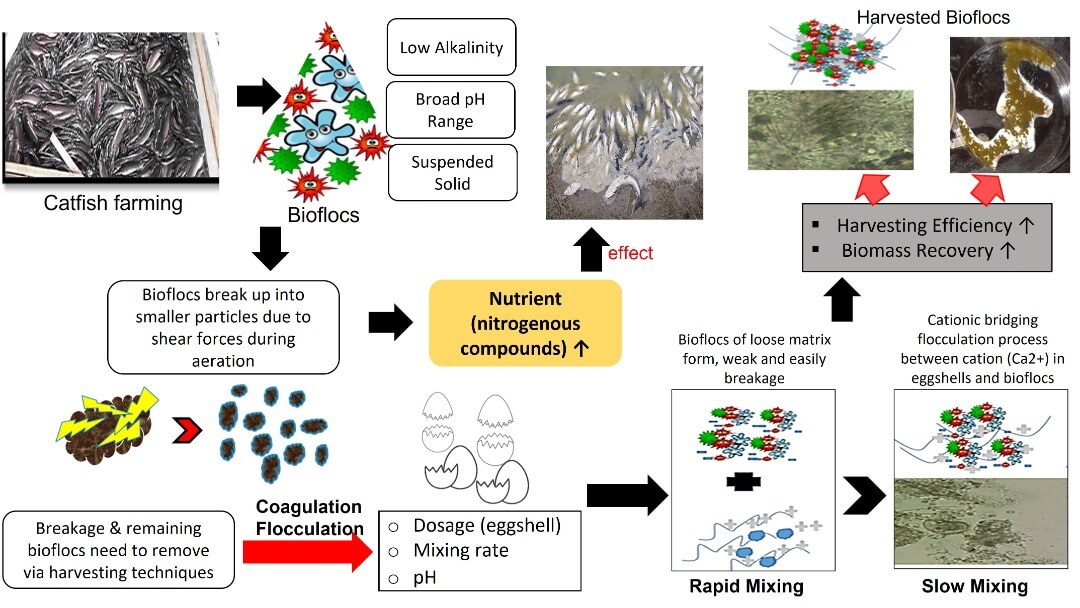
Keywords
As food consumption has increased with population growth, aquaculture has become a growing industry for sustaining food supplies [1]. Nevertheless, the expansion of the aquaculture industry is constrained due to the environmental pollution resulting from the effluent rich in nutrient compounds such as phosphorus, ammonia, nitrite, nitrate and pathogens [2]. Aquaculture systems adopting Bioflocs Technology (BFT) could solve this problem by effectively removing the nutrients while generating beneficial microbial biomass of high protein content [3]. Bioflocs are defined as an agglomeration of macroaggregates diatoms, macroalgae, microalgae, fungi, faecal pellets, exoskeletons and dead organisms, bacteria, and invertebrates [4].
BFT enhances water quality by recycling nutrients and maintaining carbon and nitrogen ratio by introducing carbon sources to stimulate heterotrophic bacteria. The bacteria convert the nitrogenous waste from the uneaten feed and faeces into microbial biomass, making it possible for the protein (present in the feed and microorganism) to be eaten twice by the cultured fish and simultaneously reducing the waste generated in the aquaculture system [5]. This is due to the existence of microorganisms with ability to degrade ammonia, nitrite and chemical oxygen demand (COD) in aquaculture wastewater [6].
Although BFT shows high productivity, low feed conversion ratios, and provides a stable culture environment in the aquaculture industry, there are several challenges needed to be solved for the successful application of BFT, namely: determining the optimal C/N ratios, temperature fluctuations, low alkalinity and salinity, broad pH range, and high concentration of suspended solids. Solids concentration management in BFT systems is also a problem that should be highlighted. Excessive solids concentration has an adverse effect on the performance of BFT systems, including clogging gills of aquatic life that affect the productive system [7]. Therefore, this technology requires a comprehensive understanding of all microorganisms involved in every biological filter process as part of the BFT system [8]. In the activated sludge process, bioflocs can break up into smaller particles due to shear forces during aeration, pumping and dewatering process. This condition led to the breakage of weak bioflocs and could generate a large number of smaller particles that do not settle readily. This circumstance led to the increase of suspended solids and turbidity in the water bodies [9].
Therefore, the use of bioflocculant is needed to encounter the problems coagulation-flocculation method. In this process, flocculants are valuable agents for the agglomeration of colloids, cells and suspended particles. Flocculants can be categorized into three groups: synthetic organic flocculants, such as polyethyleneimine and polyacrylamide, inorganic flocculants, such as aluminium sulphate and poly aluminium chloride. And natural flocculants (bio-flocculants) such as chitosan [10] and various bioflocculants include chitosan, eggshell, Moringa oleifera, and microbial flocculants such as Aspergillus niger and Scenedesmus [11,12]. These types of flocculants have great potential for use in industrial applications. However, limitations, such as low flocculation efficiency and large dosage requirement, need to be dealt with [13].
Chicken eggshells are greatly recommended as bio-flocculant because they are non-toxic, non-corrosive and safe to handle [14]. Hence, the present study was proposed to determine the optimum condition of dosage, pH and settling time of eggshells as bio-flocculant to harvest the remaining or breakage bioflocs and maintain the alkalinity level. Besides, the reduction in alkalinity and pH occurs due to the consumption of inorganic carbon by bacteria present in the bioflocs. As the level of alkalinity becomes crucial in stabilizing the aquaculture system, the characteristics of eggshells that comprise an amorphous calcium carbonate matrix and the mineralized material contribute to the alkalinity supplementation [15]. Moreover, eggshells have a high cationic (Ca2+)charge density and can, thus, strongly adsorb and destabilize negative particles, such as negatively charged microbial cells, especially microalgae cells [16]. Calcium (principally precipitate) plays a significant role in the formation and growth of granules. In contrast, the location of calcium precipitates and microbial ecology of the granule appeared to have no additional effect on the shear strength [17].
The positive effect of Ca2+ on the aggregation of non-fed fine anaerobic granular sludge is likely to induce the formation of bigger particles and thus prevent their wash-out from anaerobic bioreactors due to Ca2+ addition significantly altered their limit viscosity values [18]. Besides, Ca2+ promotes cell-to-cell bridging formation, improving the biopolymer’s aggregation and stabilization, which could enhance bioflocculation and the overall granulation process [19]. Therefore, chicken eggshell, as a polymer with high cationic charge density has the potential to strongly agglomerate bioflocs. It can retain microbial cells on its surface through charge neutralization and polymer bridging. Consequently, it is crucial to surmount these problems and improve the flocculation processes to optimize its effective utilization. The harvested bioflocs can be used in ex-situ feeding applications with acceptable water quality performance [20].
Bioremediation treatment utilizing BFT was carried out in batch culture with six different pH of 5, 6, 7, 8, 9 and 10 with an optimal C/N ratio of 15 based on our previous study stated that treatment of C/N 15 was determined as the optimum C/N ratio yielding the highest removal of ammonia (98.7%) [21]. Optimization of C/N ratio is specific to different types of aquaculture species selected, and it is crucial to effectively reduce the nutrient concentration in water. Biofloc was generated by adding organic carbon and continuous aeration, reducing dissolved nitrogen from 22% to 14%, sedimentary nitrogen from 49% to 5%, and increasing nitrogen accumulation in biofloc and fish biomass. Molasses acting as carbon sources were transferred to the treatment culture tank after being fermented for 24 h to enhance the breakdown process by the bacteria or microorganisms for bioflocs formation. The adjustment of C/N ratio by molasses was adopted from the experiment [21]. In order to observe the characteristics of bioflocs, 18 units of rectangular tanks were designed for the bioremediation treatment, and three other tanks served as controls (without the addition of a carbon source and pH adjustment). The procedure for determining the amount of molasses required for an effective treatment efficiency based on the amount of fish nitrogen excretion is shown in Fig. 1.
Figure 1: Carbon-Nitrogen procedure measurements
In order to ensure uniform wastewater characteristics, 20 individuals of catfish (Clarias gariepinus) with an average weight of 6 g/fish were maintained in each of the 8 L treatment tanks at a controlled temperature of about 28 ± 2°C. The aerator was set up in the middle section of the tank with continuous aeration. Feed (Dindings Super Keli Fish Feed 96000 with 35.43% crude protein), approximately 2% of the fish weight was given once daily. The substance of hydrochloric acid (HCL) or sodium hydroxide (NaOH) was added to the system for 30 days to maintain the pH set for the experiment. The accumulations of ammonia and nitrate concentration were observed after three days of culture period in freshwater [7]. After three days of fish rearing in each treatment tank, the water sample was collected for water quality testing to investigate the nitrogenous compound removal under the effect of pH conditions. Then, the optimal C/N ratio and pH condition for bioflocs formation was used to investigate the performance of chicken eggshells as a coagulant in harvesting the remaining bioflocs. Finally, the mechanism output was used to determine the optimal condition of the harvesting process based on the harvesting efficiency. The potential users of the harvested product produced from this research which consists of solid product (bioflocs biomass) and liquid product (settled water) were determined based on proximate analysis (AOAC Method) and water quality analysis.
The volume of bioflocs was produced to indicate the biofloc performance. A simple method to measure the in-situ bioflocs formation was to use Imhoff cone [7]. 1000 mL of water sample was collected and transferred into Imhoff cones. Then, the volume of the biofloc was allowed to settle for 15 min before the water quality analyses and harvesting process.
Water quality testing is an integral part of environmental monitoring in the aquaculture industry and is highlighted in this study. The water quality analyses were carried out with the collection of 50 mL of the water sample from each treatment and control tank at 3-day intervals until 30 days treatment period. The water sample was centrifuged at 6000 rpm for 10 min by Eppendorf 5702 Variable Speed Multi-Purpose Centrifuge to obtain a clear supernatant [2]. The supernatant was analyzed using a Dual-Beam UV-Vis spectrophotometer to monitor the ammonia, nitrite, and nitrate concentration. The percentage of ammonia removal was calculated using Eq. (1):
2.4 Preparation of Chicken Eggshell Solution
The collected chicken eggshell from cafeterias around Universiti Malaysia Terengganu (UMT) was thoroughly washed using warm distilled water. Then, the eggshell was dried in a conventional oven at 104°C for 24 h. The dried eggshell was ground and sieved using a 325-mesh sieve to obtain a fine powder. The chicken eggshell solution (bio-flocculant) was prepared based on the method reported by [14]. The stock solution was prepared by dissolving 100 mg of eggshell powder in 10 mL of 0.1 mol L−1 HCl solution with continuous stirring at 100 rpm for 30 min until a clear solution was achieved. The stock solution was diluted with 100 mL of deionized water to obtain a final concentration of 1000 mg L−1.
2.5 Harvesting of Bioflocs Using Chicken Eggshell Powder (CESP)
Excess bioflocs commonly occur in the culture ponds that use BFT. The excess bioflocs that were not settled at the bottom tank and released as waste from BFT were harvested using eggshells as a coagulant. It can be used as a feed for the aquaculture industry while minimizing environmental problems. The floc jar test was used to determine the optimal condition in terms of dosages of eggshell and mixing rate of eggshell. Various dosages of (0, 0.1, 0.15, 0.2, 0.25, 0.3, 0.35, 0.40, 0.45 and 0.5 g L−1) and pH ranges (5–10) were run at fixed rapid and slow mixing rate at 150 and 30 rpm, respectively. After determining the optimal dosage and pH, this study examined the flocculation condition by varying the slow mixing rate from 10 to 50 rpm. The optical density of the supernatant was measured at 600 nm using Dual-Beam UV-Vis Spectrophotometer (Shimadzu UV-1800, Japan) to determine the flocculation by determination of harvesting efficiency and biomass recovery. The harvesting efficiency (%) and biomass recovery (%) of nutrients were determined by Eqs. (2) and (3):
where ODinitial is optical density 600 nm of initial culture and ODfinal is optical density 600 nm at clarified zone.
Statistical analyses of this study were performed through IBM SPSS ver. 23.0. Normality and homogeneity of variances of the data were satisfied via Shapiro-Wilk test and Levene’s test, respectively. The removal efficiency of ammonia (%) in different pH conditions (pH 5, pH 6, pH 7, pH 8, pH 9, and pH 10) in BFT culture system was analyzed by One-Way Analysis of Variance (ANOVA), followed by Tukey HSD test. Results were considered as statistically significant at p < 0.05 in this experiment.
3.1 Effect of pH on Biofloc Formation
The study on the effect of pH was carried out at an optimal C/N ratio of 15 for 30 days treatment period to evaluate the performance of biofloc (biofloc volume) at six different pH (5, 6, 7, 8, 9 and 10). Based on Fig. 2, the acclimatization period observed for biofloc at the beginning of treatment period for about one week. The biofloc formation was observed from the Day 0 to Day 6 onwards. The volume of biofloc at pH 7 and 8 showed a significantly high trend or pattern as compared to other pH. Whereas the biofloc volume at other pH (5, 6, 9 and 10) were relatively low. During the treatment period, pH 7 and 8 produced the highest biofloc volume of more than 90.0 mL L−1.
Figure 2: Volume of C/N 15 bioflocs at different pH for 30 days treatment period
This result is in accordance with Luo et al. [22], which reported that pH at neutral to weakly alkaline conditions resulted in high growth of bacteria and enhanced the formation of biofloc. An optimum pH concentration must be maintained to produce high biofloc formation since the optimum pH can increase microbial activity. This finding was supported by Martins et al. [23], who stated that the abundance of microorganisms depended on the pH of the environment (pH 6.5 to 7). Nasir et al. also reported a similar finding [24] that pH from 7 and above is suitable for the bacteria to enhance biofloc formation.
3.2 Bioflocs Performance in Reducing Nitrogenous Compound
The changing of pH might exhibit a low rate of microbial nitrogen assimilation and lead to increased inorganic nitrogen such as ammonia, nitrite, and nitrate in the culture system. This circumstance explains that the difference in pH could affect the ammonia concentration between the treatments. Table 1 shows the removal of the nitrogenous compound (ammonia) and the remaining ammonia concentration at various pH values ranging from 5 to 10. This study showed that the pH condition ranging from pH 7 to 8 showed the best performance of biofloc due to the capability of maintaining an effective nutrient reduction. This condition is due to the high biofloc volume recorded in this pH range (7 to 8) that promoted the nitrogen accumulation process by the abundance of microbial cells [4]. There are no data recorded for ammonia removal at Day 0 up to Day 6 for all pH. This is because autotrophic nitrification and heterotrophic nitrification were delayed during the lag period, so it has a long generation time to acclimate to a new environment [25]. Moreover, during this stage (Day 0 to 6), there was no biofloc formation which led to the low density of autotrophic and heterotrophic microorganisms [21]. This condition was parallel to the Navada et al. [26], which stated that the availability of nitrogen and organic carbon in water treatment resulted in a high density of autotrophic and heterotrophic microorganisms.
At pH close to neutral (7–8), a significantly high ammonia removal efficiency of more than 90% was achieved, with remaining ammonia concentration recorded at 0.016 and 0.018 mg L−1, respectively. In contrast, ammonia removal efficiency recorded at the other pH 5, 6, 9 and 10 were lower than that recorded at pH 7 and 8. This condition indicates the occurrence of incomplete decomposition processes performed by the probiotic bacteria; thus, nutrient removal, especially ammonia tended to decrease [27]. Furthermore, the ammonia concentration recorded on day 30 for both pH 7 and 8 showed compliance with the water quality standard of specified water sources in fish farms less than 0.3 mg L−1 and no statistically significant differences (p > 0.05 in HSD-Tukeys test) were observed [22]. However, at a pH of strong alkaline (pH 10), the trend for the R (remaining concentration of ammonia) presented from 9th day–30th day showed fluctuation. This circumstance is because ammonia depends on the pH of the culture, and the concentration of free ammonia increases with pH. Increased pH encourages the production of more toxic unionized forms (NH3). At the daytime, with the source of solar energy, the photosynthesis process is in active mode, and this will contribute to the high dissolved oxygen (DO) level. The DO concentration affects the concentration of ammonia. This is because the heterotrophic and autotrophic bacteria can break up organic compounds and ammonia with an adequate DO concentration [28]. Whereby during the nighttime (very low), so DO become lower with no occurrence of the photosynthesis process. Therefore, the cycle process of day and night might influence the reading of ammonia remaining concentration in the treatment tank.
At pH 7 and 8, the concentration of ammonia, nitrite and nitrate was low from Day 21 onwards. This elucidated that the bioflocs underwent formation and breakage by utilizing the available nutrient in the treated water. Based on Fig. 2, a significant maximum bioflocs growth and surplus likely occurred on Day 21. Since the catfish was more endurance than the tilapia, this statement was in line with the research by Kanu et al. [29], which found that catfish had a significantly higher survival probability than tilapia, so the surplus bioflocs should be more than 50 mg L−1. Therefore, surplus bioflocs should be harvested.
3.3 Performance of Chicken Eggshell as Bio-Flocculant in Harvesting Bioflocs
Harvesting technology consists of various methods such as centrifugation, gravity filtration, ultrafiltration, flotation and flocculation [30]. However, this study focused on flocculation using chicken eggshells as bio-flocculant for harvesting excess bioflocs. The important characteristics of eggshells are non-toxic, non-corrosive and easy or safe to handle. Thus, this bio-waste is potentially suitable for use as a coagulant [14].
3.3.1 Effect of Dosage Eggshell on Harvesting Process
The floc jar test results showed optimal flocculation process performance with the appropriate bio-flocculant dosage, pH and mixing agitation condition. The effect of different eggshell dosages (0.10, 0.15, 0.20, 0.25, 0.30, 0.35, 0.40, 0.45 and 0.5 g L−1) on the efficiency of harvesting bioflocs was monitored. Based on Fig. 3, the maximum harvesting efficiency and biomass recoveries were 81% and 80%, respectively, with the eggshell dosage recorded at 0.25 g L−1. However, Choi [14] reported that the dosage of eggshell 0.08 g L−1 was encouraging dosage for harvesting single species, Chlorella sp. On the contrary, this study focused on harvesting a complex of species/microorganisms contained in bioflocs. The encouraging harvesting efficiency achieved using the same eggshell bio-flocculant found that the dosage of eggshell 0.08 g L−1 was used for harvesting only one species (Chlorella sp.) [14]. However, this study focused on harvesting a complex of species/microorganisms contained in bioflocs.
Figure 3: Effect of eggshell dosage on harvesting efficiency and biomass recovery
3.3.2 Effect of Mixing Rate on Harvesting Process
High efficiency of biomass recovery of up to 80% was achieved at a slow mixing rate between 30 and 50 rpm, as shown in Fig. 3. 30 rpm was found to show the best harvesting efficiency and biomass recovery of 81% and 80%, respectively (Fig. 4). This slow flocculation mixing rate was appropriate for bioflocs cells to be agglomerated with the eggshells. The results indicated that the mixing rate had a significant impact on flocculation efficiency, including harvesting efficiency. This is in line with the finding from Bakar et al. [21] and Li et al. [31], which stated that the mixing regime or mixing rate becomes a critical factor that controls the flocculation process towards the perspective of harvesting efficiency and energy saving.
Figure 4: Effect of mixing rate on harvesting and biomass recovery
3.3.3 Effect of pH on Harvesting Bioflocs
The optimum growth pH refers to the most favorable pH for the growth of an organism. The lowest pH value an organism can tolerate is called the minimum growth pH, and the highest is called the maximum growth pH [32]. The selected optimal dosage 0.25 gL−1 and mixing rate 30 rpm that produced the highest harvesting efficiency of more than 80% was used to study the effect of pH (Fig. 5). The results showed that pH at 6–7 was appropriate since it produced the highest harvesting efficiency of more than 80%. A similar group of harvesting efficiency and removal efficiency was discovered between pH 6 and pH 7 (v/v) via Post hoc Tukey’s HSD test (p > 0.05). However, a slight decrease in the harvesting efficiency was observed (65%) when the pH was increased to 8–10. The effect of pH could be explained by the physical properties of eggshells and physicochemical interactions between eggshells and microorganisms in bioflocs cells [33]. At neutral pH, coagulants retained a coil-like structure that fascinated the other microorganisms in bioflocs to bind and form a large molecule, resulting in high harvesting efficiency.
Figure 5: Effect of eggshell pH on harvesting efficiency and biomass recovery
Besides, the divalent metal ion, such as ion calcium (Ca2+) that was present in bio-coagulant (eggshell), could influence the harvesting efficiency by coagulating the negatively charged microorganisms in bioflocs to form a large and robust molecule [34]. At low pH, the hydrogen ion concentration was high. Thus, ion Ca2+ had to compete with the ion hydrogen for binding sites on microorganisms in bioflocs, and this led to reduced harvesting efficiency [35]. On the contrary, at pH 6–7 with a low concentration of hydrogen ion, the presence of Ca2+ promotes the binding process with microorganisms in bioflocs that produce higher harvesting efficiency and contribute to higher biomass recovery. For example, the optimal pH between 6–7 produced a higher biomass recovery of >70%. In addition, the promotion of Ca2+ through eggshells could maintain water quality in BFT by the maintenance of alkalinity levels in order to minimize the daily fluctuation of pH [36].
The results obtained from this study demonstrated the best condition of effective bioflocs formation at pH close to neutral with recorded the encouraging of ammonia removal at day-30 of more than 98% percentage removal efficiency. However, a little bit high concentration of ammonia (0.75 mg L−1) was recorded on day-18 due to the optimum growth of bacteria at this stage. In addition, the utilization of eggshell as an organic matrix showing potential to be used as a coagulant to control the nutrient in aquaculture wastewater with more than 80% harvesting efficiency under condition of 0.25 g L−1 of eggshell dosage; rapid and slow mixing rate of 150 rpm and 30 rpm; and pH of 6 to 7. The use of eggshells indicated good performance in harvesting bioflocs and polishing aquaculture wastewater and is considered a sustainable practice for the aquaculture industry towards an environmentally friendly approach.
Acknowledgement: This work was funded by the Ministry of Higher Education (MOHE), Malaysia under Higher Institution Centre of Excellence (HICoE), Institute of Tropical Aquaculture and Fisheries (AKUATROP) Program [Vot. No. 63933, JPT.S(BPKI) 2000/016/018/ 015 Jld.3 (23) and Vot. No. 56050, UMT/PPPI/2-2/5 Jld.2 (24)]. All authors would like to dedicate special gratitude to whoever involves and participated in this research project.
Funding Statement: The authors received no specific funding for this study.
Author Contributions: Hajjar Hartini Wan Jusoh: draft the original manuscript, Nor Azman Kasan: Design and supervise the research project, also comments on the critical part of manuscript, Hidayah Manan: formatting, conduct on final revision of manuscript & submission of manuscript, Nurfarahana Mohd Nasir: comments on the critical manuscript writing, Fareza Hanis Mohd Yunos: comments on the critical manuscript writing, Sofiah Hamzah: comments on the critical manuscript writing, Ahmad Jusoh: supervise of the research project and comments on the critical manuscript writing.
Availability of Data and Materials: Data are available upon reasonable request from the corresponding author.
Ethics Approval: All applicable international, national, and/or institutional guidelines for the care and use of animals or chemicals handling were fully utilized and followed.
Conflicts of Interest: The authors declare that they have no conflicts of interest to report regarding the present study.
References
1. Cai, J., Zhou, X. (2019). Contribution of aquaculture to total fishery production: The 50-percent mark. FAO Aquaculture Newsletter, 60, 43–45. [Google Scholar]
2. Hamzah, S., Yatim, N. I., Alias, M., Ali, A., Rasit, N. et al. (2019). Extraction of hydroxyapatite from fish scales and its integration with rice husk for ammonia removal in aquaculture wastewater. Indonesian Journal of Chemistry, 19(4), 1019–1030. DOI 10.22146/ijc.40907. [Google Scholar] [CrossRef]
3. Avnimelech, Y. (2009). Biofloc technology: A practical guidebook. Baton Rouge, Louisiana, USA: The World Aquaculture Society. [Google Scholar]
4. Jamal, M. T., Broom, M., Al-Mur, B. A., Al Harbi, M., Ghandourah, M. et al. (2020). Biofloc technology: Emerging microbial biotechnology for the improvement of aquaculture productivity. Polish Journal of Microbiology, 69(4), 401–409. DOI 10.33073/pjm-2020-049. [Google Scholar] [CrossRef]
5. Sujjat, A. A., Al-Najib, B. B., Mohammad, T. B. M. L. (2020). Bioconversion of fish hatchery waste as feed in the production of live feed. Advances in Bioscience and Biotechnology, 11(7), 289–300. [Google Scholar]
6. Xu, H., You, S. H. (2016). A review on treatment of aquaculture wastes. 2nd International Conference on Education Technology, Management and Humanities Science, pp. 748–751. https://www.atlantis-press.com/proceedings/etmhs-16/25849374. [Google Scholar]
7. Hargreaves, J. A. (2013). Biofloc production systems for aquaculture, vol. 4503, pp. 1–11. Stoneville, MS: Southern Regional Aquaculture Center. [Google Scholar]
8. Faizullah, M., Rajagopalsamy, C. B. T., Ahilan, B., Daniel, N. (2019). Application of biofloc technology (BFT) in the aquaculture system. Journal of Entomology and Zoology Studies, 7(4), 204–212. [Google Scholar]
9. Wilen, B. M., Lumley, D., Mattsson, A., Mino, T. (2008). Relationship between floc composition and flocculation and settling properties studied at a full scale activated sludge plant. Water Research, 42(16), 4404–4418. [Google Scholar]
10. Yunos, F. H. M., Nasir, N. M., Jusoh, H. H. W., Khatoon, H., Lam, S. S. et al. (2017). Harvesting of microalgae (Chlorella sp.) from aquaculture bioflocs using an environmental-friendly chitosan-based bio-coagulant. International Biodeterioration & Biodegradation, 124, 243–249. [Google Scholar]
11. Hamid, S. H. A., Lananan, F., Din, W. N. S., Lam, S. S., Khatoon, H. et al. (2014). Harvesting microalgae, Chlorella sp. by bio-flocculation of Moringa oleifera seed derivatives from aquaculture wastewater phytoremediation. International Biodeterioration & Biodegradation, 95, 270–275. [Google Scholar]
12. Jusoh, H. H. W., Yunos, F. H. M., Mohd, N., Nasir, S. H., Jusoh, A. (2019). Utilization of auto-flocculate of microalgae, Scededesmus sp. for harvesting of freshwater microalgae, Chlorella sp. biomass. Journal of Sustainability Science and Management, 14(2), 83–89. [Google Scholar]
13. Czemierska, M., Szcześ, A., Hołysz, L., Wiater, A., Jarosz-Wilkołazka, A. (2017). Characterisation of exopolymer R-202 isolated from Rhodococcus rhodochrous and its flocculating properties. European Polymer Journal, 88, 21–33. [Google Scholar]
14. Choi, H. J. (2015). Effect of eggshells for the harvesting of microalgae species. Biotechnology & Biotechnological Equipment, 29(4), 666–672. [Google Scholar]
15. Ketta, M., Tumova, E. (2016). Eggshell structure, measurements, and quality-affecting factors in laying hens: A review. Czech Journal of Animal Science, 61(7), 299–309. [Google Scholar]
16. Musa, M., Ward, A., Ayoko, G. A., Rösch, C., Brown, R. et al. (2020). Single-step dynamic dewatering of microalgae from dilute suspensions using flocculant assisted filtration. Microbial Cell Factories, 19(1), 222. [Google Scholar]
17. Cunha, J. R., Schott, C., van der Weijden, R. D., Leal, L. H., Zeeman, G. et al. (2018). Calcium addition to increase the production of phosphate granules in anaerobic treatment of black water. Water Research, 130, 333–342. [Google Scholar]
18. Wang, Z., Zhu, C., Yi, M., Yang, Z., Feng, Q. et al. (2018). Effect of the Ca2+ concentration on anaerobic digestion and microbial communities of granular sludge. BioResource, 13(3), 6062–6076. [Google Scholar]
19. Cunha, J. R., Morais, S., Silva, J. C., van der Weijden, R. D.,Hernández Leal, L. et al. (2019). Bulk pH and carbon source are key factors for calcium phosphate granulation. Environmental Science Technology, 53(3), 1334–1343. [Google Scholar]
20. Khatoon, H., Banerjee, S., Yuan, G. T. G., Haris, N., Ikhwanuddin, M. et al. (2016). Biofloc as a potential natural feed for shrimp post larvae. International Biodeterioration & Biodegradation, 113, 304–309. [Google Scholar]
21. Bakar, N. S. A., Nasir, N. M., Lananan, F., Hamid, S. H. A., Lam, S. S. et al. (2015). Optimization of C/N ratios for nutrient removal in aquaculture system culturing African catfish (Clarias gariepinus) utilizing bioflocs technology. International Biodeterioration & Biodegradation, 102, 100–106. [Google Scholar]
22. Luo, L., Zhao, Z., Huang, X., Du, X., Wang, C. et al. (2016). Isolation, identification, and optimization of culture conditions of a bioflocculant-producing bacterium Bacillus megaterium SP1 and its application in aquaculture wastewater treatment. BioMed Research International, 2016, 2758168. DOI 10.1155/2016/2758168. [Google Scholar] [CrossRef]
23. Martins, G. B., da Rosa, C. E., Tarouco, F. D. M., Robaldo, R. B. (2019). Growth, water quality and oxidative stress of Nile tilapia Oreochromis niloticus (L.) in biofloc technology system at different pH. Aquaculture Research, 50(4), 1030–1039. [Google Scholar]
24. Nasir, N. M., Bakar, N. S., Lananan, F., Abdul Hamid, S. H., Lam, S. S. et al. (2015). Treatment of African catfish, Clarias gariepinus wastewater utilizing phytoremediation of microalgae, Chlorella sp. with Aspergillus niger bio-harvesting. Bioresource Technology, 190, 492–498. [Google Scholar]
25. Delgadillo-Mirquez, L., Lopes, F., Taidi, B., Pareau, D. (2016). Nitrogen and phosphate removal from wastewater with a mixed microalgae and bacteria culture. Biotechnology Reports, 11, 18–26. [Google Scholar]
26. Navada, S., Knutsen, M. F., Bakke, I., Vadstein, O. (2020). Nitrifying biofilms deprived of organic carbon show higher functional resilience to increases in carbon supply. Scientific Reports, 10(1), 7121. [Google Scholar]
27. Ridvan, K., Nevzat, S., Demet, T., Aysel, V., Tayfun, A. et al. (2015). Isolation and identification of bacterial strains from decomposing hazelnut husk. Compost Science & Utilization, 23(3), 174–184. [Google Scholar]
28. Purwono, Rezagama, A., Hibbaan, M., Budihardjo, M. A. (2017). Ammonia-nitrogen (NH3-N) and ammonium-nitrogen (NH4+-N) equilibrium on the process of removing nitrogen by using tubular plastic media. Journal of Materials and Environmental Sciences, 8, 4915–4922. [Google Scholar]
29. Kanu, K. C., Otitoloju, A. A., Amaeze, N. H. (2022). Survival analysis of African catfish and Nile tilapia briefly exposed to complex pesticide mixtures. The Journal of Basic and Applied Zoology, 83(1), 1–15. [Google Scholar]
30. Muylaert, K., Bastiaens, L., Vandamme, D., Gouveia, L. (2017). Harvesting of microalgae: Overview of process options and their strengths and drawbacks. Microalgae-Based Biofuels and Bioproducts, 2017, 113–132. [Google Scholar]
31. Li, S., Hu, T., Xu, Y., Wang, J., Chu, R. et al. (2020). A review on flocculation as an efficient method to harvest energy microalgae: Mechanisms, performances, influencing factors and perspectives. Renewable and Sustainable Energy Reviews, 131(80), 110005. [Google Scholar]
32. Jin, Q., Kirk, M. (2018). pH as a primary control in environmental microbiology: 1. Thermodynamic perspective. Frontiers in Environmental Science, 6, 1–15. [Google Scholar]
33. LiuZhu, J. Y., Tao, Y., Zhang, Y., Li, A., Li, T. et al. (2013). Freshwater microalgae harvested via flocculation induced by pH decrease. Biotechnology for Biofuels, 6(1), 98. [Google Scholar]
34. Vandamme, D., Foubert, I., Fraeye, I., Meesschaert, B., Muylaert, K. (2012). Flocculation of Chlorella vulgaris induced by high pH: Role of magnesium and calcium and practical implications. Bioresource Technology, 105, 114–119. [Google Scholar]
35. Brandt, M. J., Johnson, K. M., Elphinston, A. J., Ratnayaka, D. D. (2017). Chemistry, microbiology and biology of water. In: Brandt, M. J., Johnson, K. M., Elphinston, A. J., Ratnayaka, D. D. (Eds.Twort’s water supply (Seventh Editionpp. 235–321. Butterworth-Heinemann, Elsevier, Ltd. [Google Scholar]
36. Wang, S., McDonnell, E. A., Sedor, F., Toffaletti, J. (2002). pH effects on measurements of ionized calcium and ionized magnesium in blood. Archives of Pathology & Laboratory Medicine, 126(8), 947–950. DOI 10.5858/2002-126-0947-PEOMOI. [Google Scholar] [CrossRef]
Cite This Article
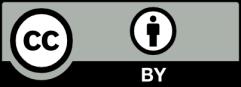