Open Access
ARTICLE
Evaluation of Self-Healing Efficiency of Microcapsule-Based Self-Healing Cementitious Composites Based on Acoustic Emission
1 College of Mechanical Engineering, Yangzhou University, Yangzhou, 225127, China
2 Faculty of Civil Engineering and Mechanics, Jiangsu University, Zhenjiang, 212013, China
* Corresponding Authors: Wenfeng Hao. Email: ; Shiping Jiang. Email:
(This article belongs to the Special Issue: Recycled Concrete Towards a Sustainable Society)
Journal of Renewable Materials 2023, 11(4), 1687-1697. https://doi.org/10.32604/jrm.2022.023795
Received 16 May 2022; Accepted 20 June 2022; Issue published 01 December 2022
Abstract
Microcapsule self-healing technology is one of the effective methods to solve the durability problem of cement-based composites. The evaluation method of the self-healing efficiency of microcapsule self-healing cement-based composites is one of the difficulties that limits the self-healing technology. This paper attempts to characterize the self-healing efficiency of microcapsule self-healing cement-based composites by acoustic emission (AE) parameters, which provides a reference for the evaluation of microcapsule self-healing technology. Firstly, a kind of self-healing microcapsules were prepared, and the microcapsules were added into the cement-based composites to prepare the compression samples. Then, the specimen with certain pre damage was obtained by compression test. Secondly, the damaged samples were divided into two groups. One group was directly used for compression tests to obtain the damage failure process. The other group was put into water for healing for 30 days, and then compression tests were carried out to study the influence of self-healing on the compression failure process. During the experiments, the AE signals were collected and the AE characteristics were extracted for the evaluation of self-healing efficiency. The results show that the compression pre damage test can trigger the microcapsule, and the compression strength of the self-healing sample is improved. The failure mechanism of microcapsule self-healing cement-based composites can be revealed by the AE parameters during compression, and the self-healing efficiency can be quantitatively characterized by AE hits. The research results of this paper provide experimental reference and technical support for the mechanical property test and healing efficiency evaluation of microcapsule self-healing cement-based composites.Keywords
Cement-based composites are one of the most widely used materials in civil engineering. However, due to the long service time and changeable service environment, the durability of cement-based composites has attracted much attention. With the development of self-healing microcapsule technology, microcapsule self-healing cement-based composites have developed rapidly, making it a hot and difficult point in the field of new materials in civil engineering [1–6]. In particular, the lack of effective self-healing effect evaluation methods has greatly limited the design of self-healing cement-based materials.
Microcapsule self-healing cement-based composites have been preliminarily studied in engineering applications. A joint research team from Cardiff University, Cambridge University and Bath University conducted a series of field experiments using self-healing technology [7]. The joint team has made several concrete wallboards on a highway in the UK. Each wallboard contains different self-healing materials. The results show that different self-healing technologies are suitable for different applications. Therefore, it is necessary to clarify the damage mechanism to customize the technology required by the actual project. Al-Tabbaa et al. [8] conducted a large-scale field test of self-healing concrete, and self-healing microcapsules containing sodium silicate were used on site. After 60 days of self-healing, it was tested again. It was found that the width and depth of cracks on the self-healing wall decreased and the permeability recovered. The results confirmed the feasibility of self-healing of microcapsules. In 2019, microcapsule self-healing concrete was used in a tunnel project in Shenzhen, China. Wang et al. [9] conducted laboratory and field experimental research. The research shows that microcapsules have both positive and negative effects on the microstructure of self-healing concrete. The self-healing ability of concrete with 10% microcapsules increases gradually with time. Although scholars have conducted more field tests, they mainly focus on the traditional evaluation methods. Traditional evaluation methods mainly focus on the relationship between porosity, pore structure, strength and impermeability before and after microcapsule healing. The traditional evaluation method has some limitations, which affect the development and practical application of self-healing concrete technology. In order to realize the reasonable design and application of materials, it is not sufficient to consider their actual service performance only from these researches. We must further understand their deformation and failure mechanism. Looking for a method that can track and monitor the damage and healing effect in time and effectively is of great significance for the practical engineering application of microcapsule self-healing concrete.
At present, the main nondestructive testing methods widely used to evaluate the self-healing efficiency include ultrasonic wave velocity method, acoustic emission (AE) test, coda interferometry, resonance frequency test, resistivity test, digital image correlation method (DIC), X-ray computed tomography (XCT), etc. In the ultrasonic wave velocity method, defects and discontinuities in the matrix can be detected by the change of ultrasonic wave velocity. Since the propagation speed of ultrasonic waves in liquid or gas phase is slower than that in cementitious matrix, the increase in propagation time means defects or cracks in the matrix. When the cracks are healed, the propagation time of ultrasonic wave is shortened, showing the healing behavior [10]. Coda interferometry is a non-destructive evaluation technology applied to monitor small velocity changes in multiple scattering media. Its principle is based on the characteristic that coda is very sensitive to small changes of scatters in media. Liu et al. [11] evaluated the self-healing process of micro cracks in mortar by coda interferometry. The results showed that the healing agent based on bacteria promoted the continuous precipitation of calcium carbonate and the wave velocity changed. The continuity of concrete material matrix has a significant impact on the resonance frequency, so it is often used as a supplementary test to detect the degree of matrix damage and healing efficiency [12]. Qiu et al. [13] evaluated the self-healing performance through crack width, resonance frequency recovery rate and microstructure analysis. Sahmaran et al. [14] used resonance frequency and rapid chloride penetration tests to evaluate the degree of damage and self-healing of cement-based materials. As a non-contact optical method used for full field strain measurement, DIC method uses the speckle on the surface of the object to move with the deformation of the specimen, and matches the speckle image before and after the deformation of the object through correlation calculation method to obtain the deformation information of the object, so as to determine the displacement field and strain field of the object [15–17]. At present, this method has been widely used in the study of crack propagation of materials and failure of members [18–20]. Al-Tabbaa et al. [8] conducted the first large-scale tests of microcapsule self-healing concrete in the UK, DIC technology was used to monitor the displacement and strain generated during loading and unloading of the self-healing wall and monitor the initiation and propagation of cracks. AE is a sound based technology [21–25], which has been widely used to monitor the crack generation and healing behavior of cement-based materials. Tittelboom et al. [26] evaluated the existence and triggering effect of microcapsules by using nondestructive testing technologies such as DIC, AE and XCT. Li et al. [27] synthesized polyurea formaldehyde (PUF) coated epoxy resin microcapsules, combined passive AE and active AE to detect the damage and healing process of microcapsule cement paste, studied the effects of microcapsule content and pre damage degree on the properties of cement paste, and revealed different crack mechanisms. Although AE has been used to evaluate the healing efficiency, not all self-healing methods are applicable. Whether AE can be used as the self-healing index depends on the mechanical properties of the used healing agent [28]. The current self-healing efficiency evaluation method is relatively single, mainly through the analysis of energy. The AE characteristics can distinguish the damage of microcapsules from other damage. For microcapsules with large damage energy, such as tubular glass microcapsules, the monitoring effect is obvious. Through the real-time processing of AE signal, the damage and healing of self-healing concrete can be judged and analyzed. AE characteristics have great potential for research. Characteristic signals such as peak frequency and amplitude can be applied to depth analysis.
In this paper, the damage state of microcapsule self-healing cement-based composites and the whole process of compression failure of healed microcapsule self-healing cement-based composites are monitored by AE technology. By analyzing the characteristics of AE signals in different stages, the failure mechanism of self-healing microcapsule cement-based composites and the influence of microcapsule contents on its failure mechanism are revealed. In addition, the AE characteristic parameters were used as the evaluation index of to quantitatively characterize the self-healing effect of microcapsule cement-based composites with different contents.
The core materials, such as sodium silicate hexahydrate and expanded Portland cement were mixed evenly, and then the core particles were produced by extrusion and rounding. The ethyl cellulose solution was sprayed on the capsule core material with a syringe and dried by blast to obtain the finished microcapsules. Parameters such as the mix ratio of microcapsule core materials and microcapsule wall materials can be found in [29,30]. Finally, microcapsules with particle size of 1–1.2 mm were selected, as shown in Fig. 1a.
Figure 1: Microcapsule-based self-healing cementitious composite samples
The manufacturing method of microcapsule self-healing cement-based composites mainly consults ISO 679-2009 (Test method for strength of cement mortar). The size of compression samples is 40 mm × 40 mm × 40 mm. The contents of microcapsules were 0%, 1%, 3%, 5% and 7%, respectively. Microcapsule content is defined as the percentage of microcapsule dosage and cement dosage. The compressive samples prepared in this paper are shown in Fig. 1b.
There are nine compressive samples of each content, of which three are used to test the strength of this batch of samples. The remaining six are divided into two groups. One group is unloaded after preloading to obtain the damaged specimens, and the other group is immersed in water for healing for 30 days after preloading and unloading to obtain the healed specimens. The pre damage method is to use 70% of the maximum compressive load as the load and maintain the load for 5 min. The purpose of this pre damage is to control the compression failure process and make the specimen reach the stage of stable propagation of microcracks. At this stage, microcapsules can be triggered with less damage, and microcapsules can give full play to their role.
In this experiment, formula (1) was used to evaluate the healing effect.
where η represents the healing efficiency of mechanical strength and reflects the self-healing effect of microcapsule self-healing cement-based materials. Fhealed is the maximum load in the healing state of self-healing cement-based composite sample. Fdamaged is the maximum load in the damaged state of the self-healing cement-based composite sample.
The test device is shown in Fig. 2, and the loading rate was set to 0.05 mm/min. The Ruandao DS 2-8A full information AE signal analyzer was used for data collection and analysis. The sensor is 16 mm in diameter and was connected to the device using a 60 dB smart AE preamplifier. The silicone oil was used as the insulating material to reduce the AE loss of the sensor. In the test, the data acquisition accuracy was 16 bit, the sampling rate was 3 M, and the sampling time interval was 0.3333 us. The threshold trigger mode was adopted. In order to ensure high signal-to-noise ratio, the threshold value was set to 250 mV.
Figure 2: Experimental setups
3.1 Compression Failure Process after Pre Damage
According to the AE characteristics of the specimens in the pre damage process and the failure process after pre damage in Fig. 3, it can be seen that the AE events occur in the whole process of compression test. It can be seen from the distribution of AE hits that the pre damage method used in this paper achieves the purpose of causing damage and triggering microcapsules to a certain extent. The general form of damage process is that the AE hits curve increases with the increase of load. The higher the slope of the curve, the more internal damage. When the load is maintained at 70% of the maximum load, the AE events increase slowly. During the destruction process after pre damage, the AE hits of compression specimens with different microcapsule contents also increase first and then decrease. The AE hits of specimens with 5% microcapsule content are the most, and the AE hits of specimens with 7% microcapsule content are the least. The increase of AE hits is mainly due to the events of microcapsule crushing and internal defect extrusion.
Figure 3: AE characteristics of specimen in pre damage process and failure process after pre damage
After preloading and unloading, the damaged specimen was obtained. Comparing the failure process of the original state specimen and the damaged state specimen, it can be found that the AE hits curve of the damaged state specimen increases slowly in the early stage, because when the load reaches 70% of the maximum load, the specimen has gone through the first stage and the second stage, and the specimen has been compacted at this time. Although the characteristic curve has changed, the law of AE hits is still similar to that of the original state specimen. The AE hits first increase and then decrease, with the largest amount of 3% and the least amount of 7%. The reason is that the damage under 70% of the maximum load is small, slight damage has occurred in the specimen, the specimen still has well service performance, and the basic properties have not changed greatly in the damage process.
3.2 Compression Failure Process after Healing of the Pre Damage
Another group of samples were pre damaged and put into water for healing for 30 days to obtain the healed samples. It can be seen from Fig. 4 that not all microcapsules will normally trigger and bond holes after expansion as shown in Fig. 4a, but some microcapsules escape as shown in Fig. 4b, leaving only an empty shell. After the microcapsule is damaged, it contacts with water, and the healing material of the capsule core flows out. After the surface microcapsule is triggered, the capsule core flows into the water and does not react with the healing agent. For the microcapsules triggered internally, the capsule core flows into the interior, expands and adheres to the healing agent, and the interior becomes denser.
Figure 4: Surface healing of samples
Fig. 5 shows the AE characteristics of the specimen during compression failure after healing. Comparing the damaged state and healed state, it can be found that the AE hits curve becomes active in the early stage of healed state, which is due to the healing effect of microcapsules. The healing effect of microcapsules depends not only on the number of microcapsules, but also on the triggering efficiency of microcapsules. The reason is that the complete microcapsules are protected by the shell and can exist in water for a long time without damage. The compressive strength of the specimens with each content is enhanced after 30 days of immersion healing, as shown in Fig. 6. The first thing to consider is that the cement-based material itself has the ability of self-healing. The experimental results of 0% microcapsule content specimens show that the self-healing effect in 30 days is limited. Comparing the specimens with 0% microcapsule content, it can be found that the strength enhancement effect of cement-based composites is obvious after adding microcapsules, especially the specimens with 3% microcapsule content have the highest healing efficiency, and the strength increases by nearly 45%. The specimens with 1% content increased slightly, and the specimens with 5% and 7% content had obvious enhancement effect. The change of AE parameters can well explain the healing mechanism. The AE characteristic curves of the 0% content specimen in the healed state and the original state are also very similar, but the AE events in the early stage are reduced after the compaction period. The healing efficiency of microencapsulation is related to the triggering effect. Microencapsulation in the matrix requires a certain amount of load to be triggered. The damage loads of 5% and 7% doped specimens are small, and it can be seen from the damage process that there are fewer AE events with 5% and 7% doping. It is very likely that the damage load is small and many microcapsules have not been triggered. When healed by immersion, the microencapsulation does not work due to the protection of the shell. The 3% content specimens suffered high damage loads and triggered more microencapsulation. The specimens with 1% additive have sufficient damage load, but the self-healing effect is poor due to the small amount of additive.
Figure 5: AE characteristics of compression failure process after healing
Figure 6: Comparison of healing effect
Compared with the damage state, a large number of AE events occurred in the early stage of failure of specimens with 1%, 3% and 5% content. The reason may be that the internal self-healing produces restoration materials, it may also be that the load is removed and the pores develop again, or it may be caused by the expansion and healing of microcapsules. The expanded microcapsules can not only block the bonding micro cracks, but also flow into the interior to block the micro pores, so that the internal structure becomes compact. Some of the AE events in the early stage may be caused by the extrusion and collision of the expanded microcapsules during the compression of the specimen. The AE hits of 1%–7% samples first increase and then decrease, the AE hits of 3% samples are the most, and the AE hits of 7% samples are the least. The difference from 0% content specimen is that the whole process AE hits of other content specimens becomes more. The increase is mainly caused by the enhancement of compressive strength and the extrusion of expanded microcapsules and matrix (see Fig. 6).
In this paper, the self-healing effect of microcapsule-based self-healing cementitious composites with different contents of microcapsules was evaluated using AE technique. The results show that the compression pre damage test can trigger the microcapsule, and the compressive strength of the self-healing samples is improved. The strength enhancement effect of cement-based composites is obvious after adding microcapsules, especially the specimens with 3% microcapsule content have the highest healing efficiency, and the strength increases by nearly 45%. The specimens with 1% content increase slightly, and the specimens with 5% and 7% content have an obvious enhancement effect. Comparing the damage state and healed state, it can be found that the AE hits curve becomes active in the early stage of healing state, which is due to the healing effect of microcapsules. The healing effect of microcapsules depends not only on the number of microcapsules, but also on the triggering efficiency of microcapsules. The failure mechanism of microcapsule self-healing cement-based composites can be revealed by the AE parameters during compression, and the self-healing effect can be quantitatively characterized by AE hits. The research results of this paper provide experimental reference and technical support for the mechanical property test and healing effect evaluation of microcapsule self-healing cement-based composites.
Funding Statement: The authors are grateful for the financial support provided by the National Natural Science Foundation of China (Grant No. 11872025) and the Six Talent Peaks Project in Jiangsu Province (Grant No. 2019-KTHY-059).
Conflicts of Interest: The authors declare that they have no conflicts of interest to report regarding the present study.
References
1. Xue, C. (2022). Performance and mechanisms of stimulated self-healing in cement-based composites exposed to saline environments. Cement and Concrete Composites, 129, 104470. DOI 10.1016/j.cemconcomp.2022.104470. [Google Scholar] [CrossRef]
2. Han, T., Wang, X., Li, D., Li, D., Xing, F. et al. (2020). Influence of strain rate on mechanical characteristic and pore structure of self-healing cementitious composites with epoxy/urea-formaldehyde microcapsules. Construction and Building Materials, 268, 121138. DOI 10.1016/j.conbuildmat.2020.121138. [Google Scholar] [CrossRef]
3. Han, T., Wang, X., Li, D., Li, D., Xing, F. et al. (2021). Uniaxial deformation characteristics and mechanical model of microcapsule-based self-healing cementitious composite. Construction and Building Materials, 274, 121227. DOI 10.1016/j.conbuildmat.2020.121227. [Google Scholar] [CrossRef]
4. Zheng, T., Su, Y., Qian, C., Zhou, H. (2020). Low alkali sulpho-aluminate cement encapsulated microbial spores for self-healing cement-based materials. Biochemical Engineering Journal, 163, 107756. DOI 10.1016/j.bej.2020.107756. [Google Scholar] [CrossRef]
5. Han, T., Wang, X., Li, D., Li, D., Xing, F. et al. (2020). Impermeability characteristics of cementitious materials with self-healing based on epoxy/urea-formaldehyde microcapsules using an immersion test. Construction and Building Materials, 259, 119782. DOI 10.1016/j.conbuildmat.2020.119782. [Google Scholar] [CrossRef]
6. Cao, B., Zhang, Y., Xu, J., Al-Tabbaa, A. (2022). Use of superabsorbent polymer in soil-cement subsurface barriers for enhanced heavy metal sorption and self-healing. Science of the Total Environment, 831, 154708. DOI 10.1016/j.scitotenv.2022.154708. [Google Scholar] [CrossRef]
7. Davies, R., Teall, O., Pilegis, M., Kanellopoulos, A., Lark, R. (2018). Large scale application of self-healing concrete: Design, construction, and testing. Frontiers in Materials, 5, 51. DOI 10.3389/fmats.2018.00051. [Google Scholar] [CrossRef]
8. Al-Tabbaa, A., Litina, C., Giannaros, P., Kanellopoulos, A., Souza, L. (2019). First UK field application and performance of microcapsule-based self-healing concrete. Construction and Building Materials, 208, 669–685. DOI 10.1016/j.conbuildmat.2019.02.178. [Google Scholar] [CrossRef]
9. Wang, X., Huang, Y., Huang, Y., Zhang, J., Fang, C. et al. (2019). Laboratory and field study on the performance of microcapsule-based self-healing concrete in tunnel engineering. Construction and Building Materials, 220, 90–101. DOI 10.1016/j.conbuildmat.2019.06.017. [Google Scholar] [CrossRef]
10. Eunjong, A., Hyunjun, K., Sung-Han, S., Sung, S., Myoungsu, S. (2017). Principles and applications of ultrasonic-based nondestructive methods for self-healing in cementitious materials. Materials, 10(3), 278. DOI 10.3390/ma10030278. [Google Scholar] [CrossRef]
11. Liu, S., Bundur, Z. B., Zhu, J., Ferron, R. D. (2016). Evaluation of self-healing of internal cracks in biomimetic mortar using coda wave interferometry. Cement & Concrete Research, 83, 70–78. DOI 10.1016/j.cemconres.2016.01.006. [Google Scholar] [CrossRef]
12. Wang, X. F., Yang, Z. H., Fang, C., Han, N. X., Zhu, G. M. et al. (2019). Evaluation of the mechanical performance recovery of self-healing cementitious materials-its methods and future development: A review. Construction and Building Materials, 212, 400–421. DOI 10.1016/j.conbuildmat.2019.03.117. [Google Scholar] [CrossRef]
13. Qiu, J., Han, S. T., Yang, E. H. (2016). Coupled effects of crack width, slag content, and conditioning alkalinity on autogenous healing of engineered cementitious composites. Cement & Concrete Composites, 73, 203–212. DOI 10.1016/j.cemconcomp.2016.07.013. [Google Scholar] [CrossRef]
14. Sahmaran, M., Yildirim, G., Noori, R., Ozbay, E., Lachemi, M. (2015). Repeatability and pervasiveness of self-healing in engineered cementitious composites. ACI Materials Journal, 112(4), 513–522. DOI 10.14359/5168730. [Google Scholar] [CrossRef]
15. Hao, W., Liu, Y., Wang, T., Guo, G., Chen, H. et al. (2019). Failure analysis of 3D printed glass fiber/PA12 composite lattice structures using DIC. Composite Structures, 225, 111192. DOI 10.1016/j.compstruct.2019.111192. [Google Scholar] [CrossRef]
16. Chen, X. W., Zhang, T. J., Liu, S. H. (2009). Accelerated ageing behavior of PMMA under hygrothermal air and water conditions. Failure Analysis and Prevention, 4, 193–195. DOI 10.3969/j.issn.1673-6214.2009.04.001. [Google Scholar] [CrossRef]
17. Liu, J., Kanwal, H., Tang, C., Hao, W. (2022). Study on flexural properties of 3D printed lattice-reinforced concrete structures using acoustic emission and digital image correlation. Construction and Building Materials, 333, 127418. DOI 10.1016/j.conbuildmat.2022.127418. [Google Scholar] [CrossRef]
18. Gui, T., Cai, S., Wang, Z., Zhou, J. (2020). Influence of aspect ratio on rolling shear properties of fast-grown small diameter eucalyptus lumber. Journal of Renewable Materials, 8(9),1053–1066. DOI 10.32604/jrm.2020.011645. [Google Scholar] [CrossRef]
19. Chen, X. W., Pei, G. L., Jin, Y. S. (2009). Study on accelerated ageing of aeronautical perspex (PMMA) in ultraviolet. Journal of Aeronautical Materials, 29, 107–112. DOI 10.3969/j.issn.1005-5053.2009.6.022. [Google Scholar] [CrossRef]
20. Saeedifar, M., Zarouchas, D. (2020). Damage characterization of laminated composites using acoustic emission: A review. Composites Part B: Engineering, 195, 108039. DOI 10.1016/j.compositesb.2020.108039. [Google Scholar] [CrossRef]
21. Guo, J., Wang, J., Chen, B. (2021). Cyclic tensile behavior of high strain hardening UHPC analyzed by acoustic emission techniques. Construction and Building Materials, 267, 121797. DOI 10.1016/j.conbuildmat.2020.121797. [Google Scholar] [CrossRef]
22. Wang, T., Wang, Z., Yang, Y., Zhou, J. (2022). Acoustic emission characteristics of different bamboo and wood materials in bending failure process. Journal of Renewable Materials, 10(2), 527–540. DOI 10.32604/jrm.2022.017955. [Google Scholar] [CrossRef]
23. Sun, Y., Wang, G., Li, Y. (2022). Study on acoustic emission characteristics of self-compacting concrete under uniaxial compression test. Journal of Renewable Materials, 10(8), 2287–2302. DOI 10.32604/jrm.2022.019660. [Google Scholar] [CrossRef]
24. Burud, N. B., Kishen, J. M. C. (2021). Response based damage assessment using acoustic emission energy for plain concrete. Construction and Building Materials, 269, 121241. DOI 10.1016/j.conbuildmat.2020.121241. [Google Scholar] [CrossRef]
25. Zhao, G., Zhang, L., Tang, C., Hao, W., Luo, Y. (2019). Clustering of AE signals collected during torsional tests of 3D braiding composite shafts using PCA and FCM. Composites Part B: Engineering, 161, 547–554. DOI 10.1016/j.compositesb.2018.12.145. [Google Scholar] [CrossRef]
26. Tittelboom, K. V., Tsangouri, E., Hemelrijck, D. V., Belie, N. D. (2015). The efficiency of self-healing concrete using alternative manufacturing procedures and more realistic crack patterns. Cement & Concrete Composites, 57, 142–152. DOI 10.1016/j.cemconcomp.2014.12.002. [Google Scholar] [CrossRef]
27. Li, W., Jiang, Z., Yang, Z. (2017). Acoustic characterization of damage and healing of microencapsulation-based self-healing cement matrices. Cement and Concrete Composites, 84, 48–61. DOI 10.1016/j.cemconcomp.2017.08.013. [Google Scholar] [CrossRef]
28. van Tittelboom, K., de Belie, N., Lehmann, F., Grosse, C. U. (2013). Use of acoustic emission analysis to evaluate the self-healing capability of concrete. In: Güneş, O., Akkaya, Y. (Eds.) Nondestructive Testing of materials and structures. (RILEM Bookseries), vol. 6. Springer, Dordrecht. DOI 10.1007/978-94-007-0723-8_7. [Google Scholar] [CrossRef]
29. Jiang, S., Lin, Z., Tang, C., Hao, W. (2021). Preparation and mechanical properties of microcapsule-based self-healing cementitious composites. Materials, 14(17), 4866. DOI 10.3390/ma14174866. [Google Scholar] [CrossRef]
30. Wang, Y., Lin, Z., Tang, C., Hao, W. (2021). Influencing factors on the healing performance of microcapsule self-healing concrete. Materials, 14(15), 4139. DOI 10.3390/ma14154139. [Google Scholar] [CrossRef]
Cite This Article
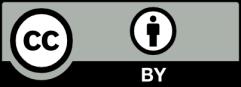
This work is licensed under a Creative Commons Attribution 4.0 International License , which permits unrestricted use, distribution, and reproduction in any medium, provided the original work is properly cited.