Open Access
ARTICLE
Seaweed Fiber Fabricated with Agar Alkali-Free Extracted from Gracilaria lemaneiformis
1 School of Chemistry and Chemical Engineering, State Key Laboratory of Bio-Fibers and Eco-Textiles, Marine Fiber New Material Institute, Qingdao University, Qingdao, 266071, China
2 Baoshan Hengfeng Textile Technology Co., Ltd., Baoshan, 678000, China
* Corresponding Authors: Cunzhen Geng. Email: ; Zhixin Xue. Email:
(This article belongs to the Special Issue: Natural Fibre Composites: Design, Materials Selection and Fabrication)
Journal of Renewable Materials 2023, 11(3), 1199-1208. https://doi.org/10.32604/jrm.2022.022976
Received 03 April 2022; Accepted 19 May 2022; Issue published 31 October 2022
Abstract
The sulfate groups in agar structure played a good role in the formation of fiber. However, commercially available agar is usually extracted from red algae by alkali treatment to decrease the content of sulfate group for the purpose of high gel strength. In this paper, an alkali-free method of agar extraction from Gracilaria lemaneiformis was proposed for the wet-spinning purpose. This method is environmentally friendly, reduces the extraction steps, saves energy, and reduces the production cost of agar fiber. The improved agar preparation process not only has higher agar yield, but also has higher molecular weight and sulfate group content, which is beneficial to the preparation and forming of fiber and makes the fiber have higher mechanical strength and elongation. Therefore, this extraction technology has broad application prospect in the textile field.Graphical Abstract
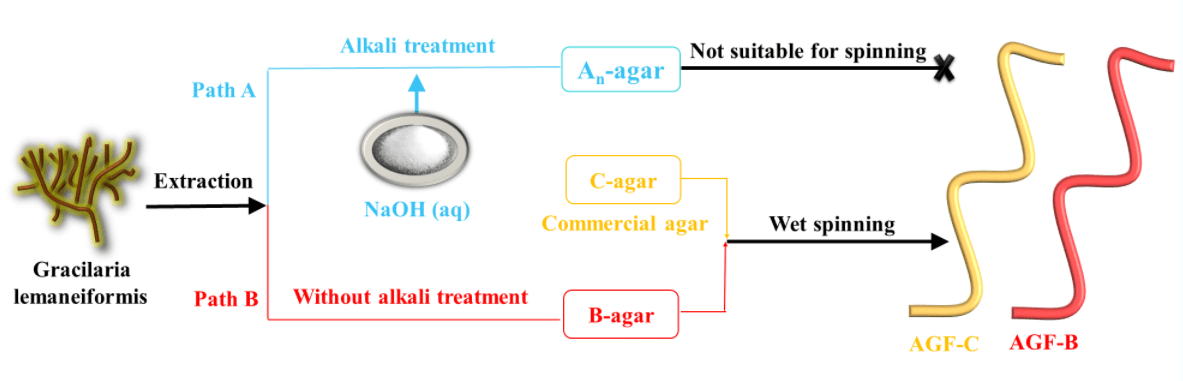
Keywords
Agar is a natural polysaccharide containing a small amount of sulfate groups extracted from red algae [1]. It consists of 3, 6-anhydro-L-galactopyranose and D-galactose alternately linked via α-1, 3 and β-1, 4 glycosidic bonds (Fig. 1 left) [2]. Agar can be fractionated into two components. The fraction with the greatest gelling capability is termed agarose, which is the neutral polysaccharide [3]. The remaining fraction is termed agaropectin and contains all the charged polysaccharide components [4]. In a previous report, Sousa et al. [5] found that in order to obtain high strength agar gel, the charged components, especially sulfate group content, should be reduced as much as possible. Meanwhile, Sousa et al. [6] reported that the high molecular weight and 3, 6-anhydro-L-galactopyranose content of the agar could reduce the loss of viscoelastic modulus and showed a compact and regular polymer network, which had a positive effect on the increase of gel strength.
Figure 1: The chemical structure of agar (left) and the schematic diagram of formation of agar fiber (right)
Agar is traditionally utilized in pharmaceutical, food and beverage industries, owing to its gelation properties and abundant bioactivities, such as hypolipidemic, immune regulation and so on [7,8]. In recent years, seaweed fibers have made great progress in textiles [9], sensors [10], wound dressing [11], etc. [12–14]. Agar has also been developed for wet spinning [15].
Gracilaria lemaneiformis is a kind of abundant, renewable and inexpensive red algae, mainly distributed in China’s Shandong, Fujian and other coastal provinces [16]. From the China Fishery Statistical Yearbook 2020, seaweed aquaculture in Chinese coastal regions had accumulated 2.5 million tones, in which Gracilaria lemaneiformis accounted for the second highest production. Gracilaria lemaneiformis contains a lot of carbohydrates, protein, dietary fiber and other active ingredients and have high economic benefits [17]. Gracilaria lemaneiformis is mainly used as abalone feed and main raw material for agar extraction [18]. In addition, the vast majority of agar is extracted from Gracilaria lemaneiformis [19].
As mentioned above, due to the pursuit of high gel strength, commercially available agar is usually extracted from red algae by alkali treatment to decrease the content of sulfate group [20,21]. Wang et al. [22] found that alkali treatment could increase the gel strength of agar and reduce the content of sulfate group and yield of agar, and the highest yield (23.14%) and gel strength (1068.15 g/cm2) were obtained when alkali concentration was 6% and treated at 80°C for 1.5 h. However, there are few studies on improving agar extraction processes for the preparation of wet-spun fiber. Meanwhile, the conventional extraction processes with alkali-treatment have several disadvantages, including consuming a lot of time, energy and low yield [23–25].
In our previous studies, we have successfully prepared agar fiber with commercial agar (i.e., C-agar in this study). Meanwhile, we speculated that the interplay between barium cation and sulfate anion could be exploited to improve the mechanical properties of agar fiber (Fig. 1 right) [15,26]. The sulfate groups in agar structure played a good role in the formation of fiber. Hence, in order to increase the content of sulfate in agar molecules, to benefit wet spinning and to reduce the economic cost, we came up with the idea of alkali-free treatment to extract agar from Gracilaria lemaneiformis for the wet-spinning of agar fiber.
In this paper, the molecular structure and solution properties of alkali-extracted and alkali-free agar from Gracilaria lemaneiformis were compared. The effect of alkali pretreatment on the molecular structure of agar was investigated. The properties of agar fibers fabricated by alkali-free agar extracted from Gracilaria lemaneiformis and commercial agar were examined.
Gracilaria lemaneiformis was harvested between September and October in 2021 at Putian, Fujian Province of China (25°25’ N 119°0’ E). Commercial agar (C-agar) was purchased from Lanji Technology Development Co., Ltd. (Shanghai, China). All the other reagents used in this article were of reagent grade and purchased from Sinopharm Chemical Reagent Co., Ltd. (Shanghai, China).
2.2 Agar Extraction and Agar Fiber Preparation
The traditional extraction method was depicted as path A in Fig. 2. The Gracilaria lemaneiformis was pretreated using NaOH with various concentrations (2 wt%, 4 wt%, 6 wt% and 8 wt%) at 80°C for 2 h. The treated Gracilaria lemaneiformis was washed to neutral pH, and then autoclaved (BXM-30R Steam Sterilizer, China) at 120°C for 120 min. Agar was obtained after immersing in ethanol, centrifugation at 5000 rpm for 10 min and freeze-drying to constant weight, and then weighed. The resulting products were labelled as An-agar, where n was the mass fraction of NaOH.
Figure 2: Steps of agar extracting (Path A. Traditional agar extracting process; Path B. Improved agar extracting process) and wet spinning process
The alkali treatment step was omitted in new extraction procedure (Fig. 2, path B). The other steps were the same as that of path A. The obtained agar via path B was labelled B-agar.
An-agar, B-agar and C-agar powders were dissolved in DMSO to obtain agar solution (12 wt%), and were referred to as An-agar-sol, B-agar-sol and C-agar-sol.
Agar fiber was spun via a wet-spinning device in Fig. 2. B-agar-sol and C-agar-sol were extruded under the pressure of 0.2 MPa and through a spinneret (60 holes, diameter = 0.08 mm × 60 mm) into the coagulation and stretch bath containing 7 wt% and 3 wt% BaCl2 solution, separately. The fibers fabrication with B-agar and C-agar were labelled agar fiber B (AGF-B) and agar fiber C (AGF-C).
2.3 Fourier Transform Infrared Spectroscopy (FTIR)
The Infrared spectra of agar and agar fibers were recorded using a Nicolet iS50 FTIR spectrometer (Thermo Fisher Scientific Co, USA). The samples were scanned 32 times with a resolution of 4 cm−1 over the scanning range of 500 to 4000 cm−1.
Zeta potentials of the agar were recorded at 25°C using a Malver Mastersizer 3000 analyzer (Malvern Instruments, Ltd., UK). 20 mg agar was added to 50 ml distilled water, which was stirred for 24 h at 95°C to form a homogeneous solution.
2.5 Gel Permeation Chromatography (GPC)
The average molecular weight (Mw) of agar was determined using a GPC machine (Malvern Instruments, Ltd., UK). The standards and RPCs were injected on A6000 M GPC column and eluted in 0.1 mol/L NaNO3 with the temperature maintained at 40°C.
Elemental analyses (EA) were conducted in elemental analyzer (Elemental vario El cube, Germany) to determine sulfur content in nascent fibers. Before this, the samples were dried at 60°C for an hour by desiccator.
2.7 Dynamic Light Scattering (DLS)
The DLS of the agar solution was performed with a Malver Mastersizer 3000 analyzer (Malvern Instruments, Ltd., UK). An-agar-sol, B-agar-sol and C-agar-sol with the volume of 0.5 ml were added to 50 ml DMSO at 95°C under stirring for 24 h, respectively. Tests were performed at 25°C with a 173° scattering angle.
2.8 Scanning Electron Microscopy (SEM)
The surface morphology of the agar fiber was observed by SEM (Quanta 250 FEG, USA). The agar fiber was coated with gold and observed at 15 kV acceleration voltage.
The crystallization characteristics were characterized with an X-ray diffractometer (Bruker D8 Advance, Germany) with 2°/min scanning rate in a 2θ range from 5° to 60° at 40 kV and 30 Ma.
The mechanical properties of the agar fibers were examined using Single Fiber Strength instrument (Instron 5565 Mechanical Tester, USA) at a test speed of 20 mm/min. Test conditions: temperature: 25 ± 1°C, humidity: 60% ± 5%, gauge length: 20 mm.
The extraction experiment, zeta potential, GPC and DLS test were repeated five times. The mechanical properties of the fibers were obtained from an average of 30 samples. The data of agar yield, the zeta potential, the Mw of agar and mechanical strength of agar fibers were presented as means ± standard deviation (SD). One-way analysis of variance (ANOVA) and least significant difference (LSD) method tests were performed on the tensile data of agar fibers using the SPSS statistical analysis software to analyze the significant differences between the mean values of fiber properties, and values of p < 0.05 were considered significant.
3.1 Effect of Alkali Treatment on An-Agar and An-Agar-Sol
The FTIR spectra of An-agar, B-agar and C-agar are shown in Fig. 3. The characteristic peaks of An-agar, B-agar and C-agar were semblable. A stretching vibration band of O-H at about 3362 cm−1 could probably be attributed to isolated O-H moieties [27]. The bands at 2920 cm−1 corresponded to asymmetric stretching vibration band of CH2 [28], and it was the characteristic peak of carbohydrate. The weak peak at 1250 cm–1 was related to the sulfate group stretching vibration [29]. The signal of sulfate group stretching vibration for An-agar decreased with increasing alkali concentration, which indicated that alkali pretreatment affected the content of sulfate group.
Figure 3: FTIR spectra of An-agar, B-agar and C-agar
According to references [30], the yield of agar extracted from the Gracilaria lemaneiformis was defined as the mass of the extracted agar divided by the mass of dry Gracilaria lemaneiformis. As shown in Fig. 4a, the yield of B-agar was 37.5% ± 1.4%, which was much higher than that of An-agars. The yields were 20.4 ± 1.2%, 13.4 ± 1.0%, 11.1 ± 0.7% and 8.7 ± 0.9% for A2-agar, A4-agar, A6-agar and A8-agar, respectively. Furthermore, agar yields decreased with increasing alkali concentration. We preliminarily speculated that this result was due to the alkali pretreatment disrupting the molecular structure.
Figure 4: The yield (a) Zeta potential (b) and the Mw (c) of An-agar, B-agar and C-agar
As shown in Fig. 4b, the Zeta potential of B-agar was -22.6 mV, while that of An-agar was smaller in value than that of B-agar, and decreased with the increase of alkali concentration. Namely, B-agar had more sulfate group than An-agar and C-agar.
As shown in Fig. 4c, the Mw of B-agar was 7.5 × 104 Da, which was larger than that of An-agar (5.5 × 104 Da, 4.6 × 104 Da, 3.4 × 104 Da, 2.2 × 104 Da) and C-agar (4.1 × 104 Da). The result further confirmed that the alkali pretreatment could destroy the molecular structure of agar with low yield and low Mw [31].
The sulfur content of An-agar, B-agar and C-agar is shown in Table 1. In a sense, the sulfur content in agar reflected the content of sulfate group because only sulfate group contained sulfur element. The results showed that the sulfur content of B-agar was the highest, much higher than that of C-agar, and the sulfur content gradually decreased with the increase of alkali pretreatment concentration, which was consistent with the above FTIR spectra and Zeta potential test results.
The particle size distributions of An-agar-sol, B-agar-sol and C-agar-sol were shown in Figs. 5a and 5b. The average particle sizes were 547.3 nm, 384.6 nm, 340.0 nm, 259.2 nm, 192.5 nm and 432.9 nm for B-agar, A2-agar, A4-agar, A6-agar, A8-agar and C-agar, respectively. The mean particle size of the An-agar-sol decreased with increasing alkali concentration, which indicated the degradation of the An-agar molecular chains. The three peaks of An-agar-sol and only two peaks of B-agar-sol in the size distribution by intensity indicated that the particle size of An-agar-sol was not uniform. Meanwhile, in the size distribution by volume, the size of the agar solution decreased gradually with increasing alkali concentration and the particle size of An-agar-sol was too small to make the solution viscous. The results further confirmed that An-agar molecular chains were degraded and B-agar had larger molecular chains with good solution properties for the preparation of fiber than An-agar and C-agar.
Figure 5: Size distribution by volume of agar solution
Due to the low yield, low molecule weight, low viscosity of agar alkali-extracted from Gracilaria lemaneiformis, it proved that An-agars were not suitable for spinning. Therefore, in the following studies, we only compared the spinning properties of B-agar and C-agar.
As we all know, the properties of polysaccharide fibers depend largely on the molecular weight and structure of polysaccharides, but at the same time, spinning parameters such as temperature, drawing ratio and spinning process also affect the properties of the fibers [32,33]. In this paper, the environmental temperature is 25°C, the tensile ratio is 1:1.2, and the parameters are controlled at the same level. Only the influence of polymer molecules on the fiber is studied.
Fig. 6a–6d showed the good morphology of AGF-B and AGF-C. The shape and structure of the fibers were determined by the gelation process of the spinning solution and the interaction between Ba2+ and sulfate group played an important role in it [34]. Moreover, AGF-B was more regular and softer than AGF-C.
Figure 6: Morphology and structure of agar fibers: a. Optical image of AGF-C; b. SEM of surface of AGF-C; c. Optical image of AGF-B; d. SEM of surface of AGF-B; e. The FTIR spectra of agar fiber; f. The XRD spectra of agar fiber
FTIR spectra of AGF-C and AGF-B were presented in Fig. 6e. The result showed that the peak of the sulfate group at 1250 cm−1 of AGF-B was slightly stronger than that of AGF-C, indicating that there were more sulfate groups in AGF-B and AGF-B was easier to bind to Ba2+.
As shown in Fig. 6f, XRD patterns of agar fiber displayed a broad and higher intensity diffraction region at 2θ-13.5° beside the typical amorphous peak at 2θ-18.4°, which indicated an orderly structure and semi-crystalline nature of agar (as a copolymer of galactose and 3, 6-anhydro-galactose) [35]. Similar results were reported by Arfat et al. [36]. However, the diffraction pattern of AGF-C exhibited higher crystallinity than AGF-B, which may be the reason why AGF-B felt softer and had higher elongation at break (Table 2) compared to AGF-C.
As shown in Table 2, AGF-B not only exhibited slight improvement of the linear intensity but also significantly increased elongation at break. The breaking elongation of the fibers increased from 18.43 ± 12.93% to 38.89 ± 13.90%. Obviously, the molecular structure of the agar seemed to directly affect the mechanical properties of the fiber, which was also proved by the tensile test results.
In general, high crystallinity results in high strength. But the high molecular weight of AFG-B made up for its weakness in strength. The relatively high sulfate group content in B-agar also improved the strength of AGF-B by combining sulfate group with barium ions. At the same time, the high molecular weight of agar ensured the integrity of the molecular chain and the amorphous structure of AGF-B provided more space for the rotation of its molecular chain, so the elongation at break increased significantly.
In this study, agar extracted from Gracilaria lemaneiformis without alkali pretreatment was adopted to prepare agar fiber. The new extraction procedure by eliminating the step of alkali treatment displayed higher yield (37.5% ± 1.4%) and molecular weight (75, 567 Da) and the size distribution of spinning solution was homogeneous and suitable for wet spinning. Furthermore, the morphology of agar fiber was improved and the elongation at the break of agar fiber was increased to 38.89% ± 13.90%. Therefore, the developed extraction procedure has promising application prospect in textile fields.
Funding Statement: This work was financially supported by the Program of National Natural Science Foundation of China (52173037), Natural Science Foundation of Shandong Province (ZR2020ME061), Changjiang Scholars and Innovative Research Team in University of Ministry of Education of China (IRT14R30), State Key Laboratory of Bio-Fibers and Eco-Textiles of Qingdao University (ZFT201810, ZKT17), Yunnan Expert Workstation Project (202105AF150061).
Conflicts of Interest: The authors declare that they have no conflicts of interest to report regarding the present study.
References
1. Li, Y., Zhao, M., Gomez, L. P., Senthamaraikannan, R., Padamati, R. B. et al. (2021). Investigation of enzyme-assisted methods combined with ultrasonication under a controlled alkali pretreatment for agar extraction from Gelidium sesquipedale. Food Hydrocolloids, 120, 106905. DOI 10.1016/j.foodhyd.2021.106905. [Google Scholar] [CrossRef]
2. Chen, X., Fu, X., Huang, L., Xu, J., Gao, X. (2021). Agar oligosaccharides: A review of preparation, structures, bioactivities and application. Carbohydrate Polymers, 265, 118087. DOI 10.1016/j.carbpol.2021.118076. [Google Scholar] [CrossRef]
3. Tabani, H., Alexovic, M., Sabo, J., Payan, M. R. (2021). An overview on the recent applications of agarose as a green biopolymer in micro-extraction-based sample preparation techniques. Talanta, 224, 121892. DOI 10.1016/j.talanta.2020.121892. [Google Scholar] [CrossRef]
4. Mostafavi, F. S., Zaeim, D. (2020). Agar-based edible films for food packaging applications–A review. International Journal of Biological Macromolecules, 159, 1165–1176. DOI 10.1016/j.ijbiomac.2020.05.123. [Google Scholar] [CrossRef]
5. Sousa, A. M. M., Gonçalves, M. P. (2015). The influence of locust bean gum on native and alkali-modified agar gels. Food Hydrocolloids, 44, 461–470. DOI 10.1016/j.foodhyd.2014.10.020. [Google Scholar] [CrossRef]
6. Sousa, A. M. M., Borges, J., Silva, A. F., Goncalves, M. P. (2013). Influence of the extraction process on the rheological and structural properties of agars. Carbohydrate Polymers, 96, 163–171. DOI 10.1016/j.carbpol.2013.03.070. [Google Scholar] [CrossRef]
7. Kang, J., Jia, X., Wang, N., Xiao, M., Song, S. et al. (2022). Insights into the structure-bioactivity relationships of marine sulfated polysaccharides: A review. Food Hydrocolloids, 123, 107049. DOI 10.1016/j.foodhyd.2021.107049. [Google Scholar] [CrossRef]
8. Kalita, P., Ahmed, A. B., Sen, S., Chakraborty, R. (2022). A comprehensive review on polysaccharides with hypolipidemic activity: Occurrence, chemistry and molecular mechanism. International Journal of Biological Macromolecules, 206, 681–698. DOI 10.1016/j.ijbiomac.2022.02.189. [Google Scholar] [CrossRef]
9. Bao, X., Hayashi, K., Li, Y., Teramoto, A., Abe, K. (2010). Novel agarose and agar fibers: Fabrication and characterization. Materials Letters, 64(22), 2435–2437. DOI 10.1016/j.matlet.2010.08.008. [Google Scholar] [CrossRef]
10. Zhang, M., Zhao, Z., Hui, B., Sun, J., Sun, J. et al. (2021). Carbonized polymer dots activated hierarchical tungsten oxide for efficient and stable triethylamine sensor. Journal of Hazardous Materials, 416, 126161. DOI 10.1016/j.jhazmat.2021.126161. [Google Scholar] [CrossRef]
11. Han, J., Han, X., Xue, Z., Wang, Q., Xia, Y. et al. (2021). An eco-friendly procedure for achieving high-yield carrageenan from Hypnea cervicornis suitable for wet spinning. Journal of Applied Polymer Science, 138, 50833. DOI 10.1002/app.50833. [Google Scholar] [CrossRef]
12. Liu, Y., Tao, Y., Wang, B., Li, P., Xu, Y. et al. (2021). Fully bio-based fire-safety viscose/alginate blended nonwoven fabrics: Thermal degradation behavior, flammability, and smoke suppression. Cellulose, 27, 6037–6053. DOI 10.1007/s10570-020-03162-1. [Google Scholar] [CrossRef]
13. Forget, A., Arya, N., Randriantsilefisoa, R., Miessmer, F., Buck, M. et al. (2016). Nonwoven carboxylated agarose-based fiber meshes with antimicrobial properties. Biomacromolecules, 17(12), 4021–4026. DOI 10.1021/acs.biomac.6b01401. [Google Scholar] [CrossRef]
14. Wang, P., Du, X., Wang, X., Zhang, K., Sun, J. et al. (2021). Integrated fiber electrodes based on marine polysaccharide for ultrahigh-energy-density flexible supercapacitors. Journal of Power Sources, 506, 230130. DOI 10.1016/j.jpowsour.2021.230130. [Google Scholar] [CrossRef]
15. Liu, J., Xue, Z., Zhang, W., Yan, M., Xia, Y. (2018). Preparation and properties of wet-spun agar fibers. Carbohydrate Polymers, 181, 760–767. DOI 10.1016/j.carbpol.2017.11.081. [Google Scholar] [CrossRef]
16. Long, X., Hu, X., Liu, S., Pan, C., Chen, S. et al. (2021). Insights on preparation, structure and activities of Gracilaria lemaneiformis polysaccharide. Food Chemistry:X, 12, 100153. DOI 10.1016/j.fochx.2021.100153. [Google Scholar] [CrossRef]
17. Han, T., Qi, Z., Huang, H., Fu, G. (2017). Biochemical and uptake responses of the macroalga Gracilaria lemaneiformis under urea enrichment conditions. Aquatic Botany, 136, 197–204. DOI 10.1016/j.aquabot.2016.09.012. [Google Scholar] [CrossRef]
18. Luo, H., Wang, Q., Zhang, C., Zhang, L., Yang, Y. (2021). Bioaccumulation and release of heavy metals during growth and decomposition of cultivated Gracilaria lemaneiformis. Marine Pollution Bulletin, 173, 113130. DOI 10.1016/j.marpolbul.2021.113130. [Google Scholar] [CrossRef]
19. Porse, H., Rudolph, B. (2017). The seaweed hydrocolloid industry: 2016 updates, requirements, and outlook. Journal of Applied Phycology, 29(5), 2187–2200. DOI 10.1007/s10811-017-1144-0. [Google Scholar] [CrossRef]
20. Xiao, Q., Wang, X., Zhang, J., Zhang, Y., Chen, J. et al. (2021). Pretreatment techniques and green extraction technologies for agar from Gracilaria lemaneiformis. Marine Drugs, 19(11), 617. DOI 10.3390/md19110617. [Google Scholar] [CrossRef]
21. Li, H., Yu, X., Jin, Y., Zhang, W., Liu, Y. (2008). Development of an eco-friendly agar extraction technique from the red seaweed Gracilaria lemaneiformis. Bioresource Technology, 99, 3301–3305. DOI 10.1016/j.biortech.2007.07.002. [Google Scholar] [CrossRef]
22. Wang, L., Shen, Z., Mu, H., Lin, Y., Zhang, J. et al. (2017). Impact of alkali pretreatment on yield, physico-chemical and gelling properties of high quality agar from gracilaria tenuistipitata. Food Hydrocoll, 70, 356–362. DOI 10.1016/j.foodhyd.2016.11.042. [Google Scholar] [CrossRef]
23. Rodriguez, M. C., Matulewicz, M. C., Noseda, M. D., Ducatti, D. R. B., Leonardi, P. I. (2009). Agar from Gracilaria gracilis (Gracilariales, rhodophyta) of the patagonic coast of Argentina-content, structure and physical properties. Bioresource Technology, 100, 1435–1441. DOI 10.1016/j.biortech.2008.08.025. [Google Scholar] [CrossRef]
24. Arvizu-Higuera, D. L., Rodríguez-Montesinos, Y. E., Murillo-Álvarez, J. I., Muñoz-Ochoa, M., Hernández-Carmona, G. (2008). Effect of alkali treatment time and extraction time on agar from Gracilaria vermiculophylla. Journal of Applied Phycology, 20, 515–519. DOI 10.1007/s10811-007-9258-4. [Google Scholar] [CrossRef]
25. Chen, H., Xiao, Q., Weng, H., Zhang, Y., Yang, Q. et al. (2020). Extraction of sulfated agar from Gracilaria lemaneiformis using hydrogen peroxide-assisted enzymatic method. Carbohydrate Polymers, 232, 115790. DOI 10.1016/j.carbpol.2019.115790. [Google Scholar] [CrossRef]
26. Wang, L., Zhang, X., Xia, Y., Zhao, X., Xue, Z. et al. (2019). Cooking-inspired versatile design of an ultrastrong and tough polysaccharide hydrogel through programmed supramolecular interactions. Advanced Materials, 41(31), 1902381. DOI 10.1002/adma.201902381. [Google Scholar] [CrossRef]
27. Guerrero, P., Etxabide, A., Leceta, I., Peñalba, M., de la Caba, K. (2014). Extraction of agar from Gelidium sesquipedale (Rodhopyta) and surface characterization of agar based films. Carbohydrate Polymers, 99, 491–498. DOI 10.1016/j.carbpol.2013.08.049. [Google Scholar] [CrossRef]
28. Yarnpakdee, S., Benjakul, S., Kingwascharapong, P. (2015). Physico-chemical and gel properties of agar from Gracilaria tenuistipitata from the lake of Songkhla, Thailand. Food Hydrocolloids, 51, 217–226. DOI 10.1016/j.foodhyd.2015.05.004. [Google Scholar] [CrossRef]
29. Sekkal, M., Legrand, P., Huvenne, J. P., Verdus, M. C. (1993). The use of FTIR microspectrometry as a new tool for the identification in situ of polygalactanes in red seaweeds. Journal of Molecular Structure, 294, 227–230. DOI 10.1016/0022-2860(93)80356-z. [Google Scholar] [CrossRef]
30. Vuai, S. A. H., Mpatani, F. (2019). Optimization of agar extraction from local seaweed species, Gracilaria salicornia in Tanzania. Phycological Research, 67(4), 261–266. DOI 10.1111/pre.12380. [Google Scholar] [CrossRef]
31. Martinez-Sanz, M., Gomez-Mascaraque, L. G., Ballester, A. R., Martinez-Abad, A., Brodkorb, A. et al. (2019). Production of unpurified agar-based extracts from red seaweed Gelidium sesquipedale by means of simplified extraction protocols. Algal Research-Biomass Biofuels and Bioproducts, 38, 101420. DOI 10.1016/j.algal.2019.101420. [Google Scholar] [CrossRef]
32. Wang, C., Dong, X., Wang, Q. (2009). Effect of coagulation on the structure and property of PAN nascent fibers during dry jet wet-spinning. Journal of Polymer Research, 16(6), 719. DOI 10.1007/s10965-009-9278-7. [Google Scholar] [CrossRef]
33. Liu, S., Tian, X., Zhang, X., Xu, C., Wang, L. et al. (2022). A green MXene-based organohydrogel with tunable mechanics and freezing tolerance for wearable strain sensors. Chinese Chemical Letters, 33, 2205–2211. DOI 10.1016/j.cclet.2021.09.063. [Google Scholar] [CrossRef]
34. Zhao, L., Wang, Y., Li, Z., Deng, Y., Zhao, X. et al. (2019). Facile synthesis of chitosan-gold nanocomposite and its application for exclusively sensitive detection of Ag+ ions. Carbohydrate Polymers, 226, 115290. DOI 10.1016/j.carbpol.2019.115290. [Google Scholar] [CrossRef]
35. Shukla, M. K., Singh, R. P., Reddy, C. R., Jha, B. (2012). Synthesis and characterization of agar-based silver nanoparticles and nanocomposite film with antibacterial applications. Bioresource Technology, 107, 295–300. DOI 10.1016/j.biortech.2011.11.092. [Google Scholar] [CrossRef]
36. Arfat, Y. A., Ahmed, J., Jacob, H. (2017). Preparation and characterization of agar-based nanocomposite films reinforced with bimetallic (Ag-Cu) alloy nanoparticles. Carbohydrate Polymers, 155, 382–390. DOI 10.1016/j.carbpol.2016.08.097. [Google Scholar] [CrossRef]
Cite This Article
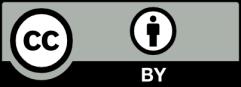