Open Access
ARTICLE
Use of Additive Based on Non-Timber Forest Products for the Ecological Stabilization of Raw Earth: Case of the Parkia Biglobosa Nut and Vitellaria Paradoxa
1
L2EGC-Laboratory of Studies and Tests in Civil Engineering, University of Sciences, Technology, Engineering, and Mathematics,
Abomey, Benin
2
IJL-Jean Lamour Institute, University of Lorraine, Nancy, France
3
LEMA-Laboratory of Energetics and Applied Mechanics, University of Abomey-Calavi, Abomey-Calavi, Benin
4
LERMAB-Study and Research Laboratory on Wood Material, University of Lorraine, Nancy, France
* Corresponding Author: Crespin Prudence Yabi. Email:
(This article belongs to the Special Issue: Environmentally Friendly and Renewable Civil Engineer Materials)
Journal of Renewable Materials 2023, 11(12), 4143-4160. https://doi.org/10.32604/jrm.2023.030509
Received 10 April 2023; Accepted 29 June 2023; Issue published 10 November 2023
Abstract
The housing sector today uses elaborate materials such as cement, iron, sand, often prohibitively expensive and whose production generates a strong environmental impact (scarcity of resources, transport, greenhouse gas greenhouse, etc.). In order to meet the challenges of sustainable development, earth construction is experiencing a resurgence of interest these days. Despite its many advantages, raw earth material has drawbacks, in particular its low mechanical resistance and its loss of geometric characteristics in the face of water, which slow down its development. As part of this study, the mechanical characteristics and durability of raw earth were improved by using residual water from the processing of Parkia Biglobosa (nere) and Vitellaria Paradoxa (shea) nuts in order to optimize its use for the construction of modern buildings. To this end, the decoctions resulting from the artisanal transformation of the nut of the Parkia Biglobosa into African mustard and of the Vitellaria Paradoxa into shea butter were added to the raw earth according to volume proportions of 25%, 50%, 75% and 100% of the aqueous solution to obtain the projected composites. Thus, mechanical characterization and durability tests were carried out on the composites obtained. The results revealed that the decoctions of Vitellaria Paradoxa and Parkia Biglobosa improve the compressive strength of the material by up to 90% and 260%, respectively. Furthermore, these decoctions improved the resistance to water penetration of the 100% additive composite by 1.5 times for Vitellaria Paradoxa and 5 times for Parkia Biglobosa. This study shows that it is possible to use decoctions as raw earth stabilizers to build modern, ecological buildings at lower energy costs. However, more in-depth studies on surface wettability and long-term durability are planned to better characterize the geomaterial.Graphic Abstract
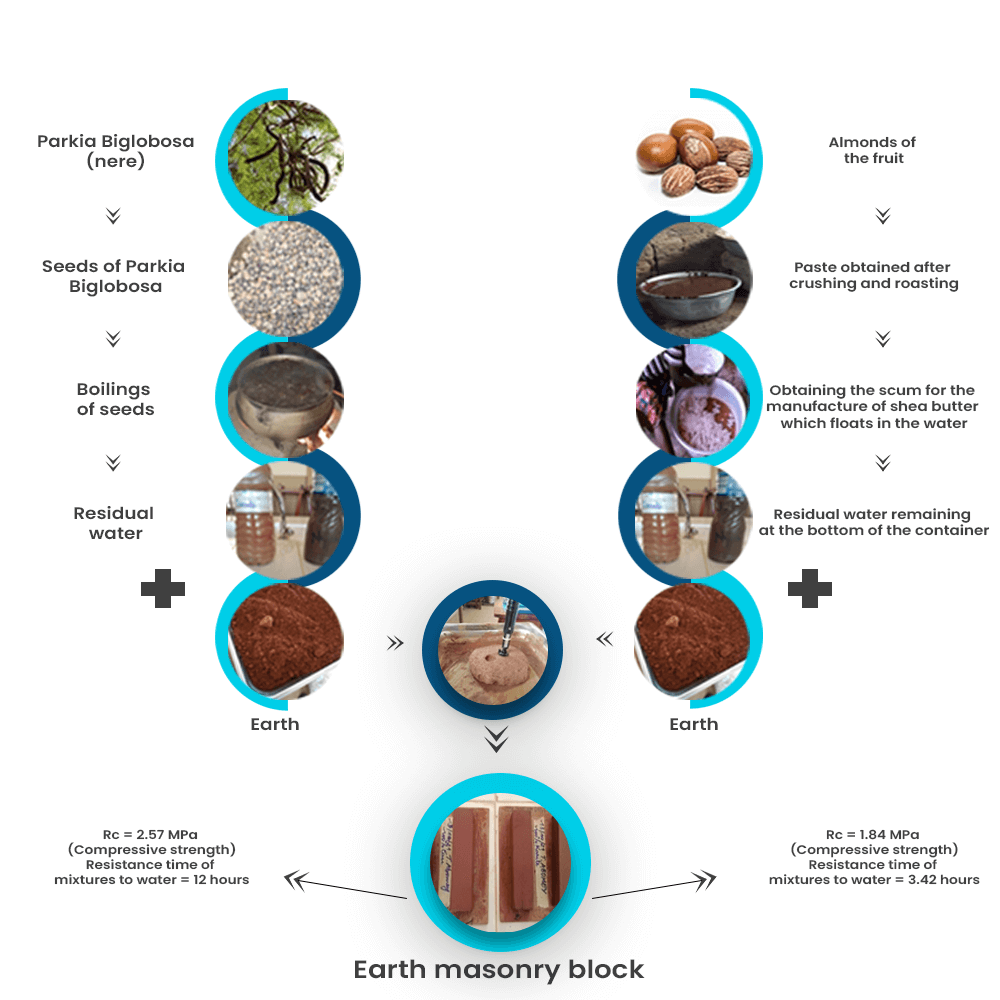
Keywords
Raw earth is one of the oldest materials used for building housing [1,2]. Even today, one-third of the world’s population lives in mud houses [3–5], especially in developing countries where more than half of the population, mostly rural, uses this type of building [6]. The United Kingdom has around half a million functional earthen dwellings, most of which were built before the 20th century [7]. In India, the census of housing stock carried out in 1971 established that 72.2% of dwellings were built of raw earth [6].
Since the appearance of cement, traditional raw earth construction techniques have been gradually abandoned to the detriment of “hard” systems considered more modern, but which are also much more expensive, polluting, energy-consuming [8], etc. Finally, these techniques are poorly adapted to the rural context, particularly in Africa. With the climate crisis, the need to reduce CO2 emissions (greenhouse gas) as much as possible is now imposed on all sectors, which is reviving the interest in this ancestral way of construction, typically ecological [9,10]. Generally, the problems associated with earthen constructions are the loss of geometric characteristics due to erosion of the surface under the effect of bad weather and low mechanical resistance [5,11–13]. However, care must be taken to improve the durability of raw earth as much as possible, and even its mechanical behavior, given its durability concerning water (humidity, erosion, mold, etc.).
For this purpose, artificial additives like cement, lime, bitumen, metakaolin, rice husk ash [14–18], etc., have been added to the raw earth, but the use of these products has no impact on the carbon balance, which puts the environmental interest of their use into perspective. The use of additives and other renewable materials of plant and/or animal origin, which can improve the properties (strength, durability, plasticity, surface appearance [19], then constitutes an interesting alternative that is important to optimize sustainable and ecological construction techniques using stabilized raw earth [10].
Remember that the compressive strength of unsterilized mud bricks is between 0.6 and 2.25 MPa [20,21]. Pressed and/or treated with a small amount of cement [22], it can reach 1.5 to 3 MPa [23].
Several studies have already focused on the interest in incorporating plant additives into raw earth. For example, some authors [11,24–27] have shown that by substituting, in a sand–clay mixture, the mixing water with a decoction of Parkia Biglobosa pods, a geomaterial was obtained with a compressive strength greater than that of the mixture without addition. They also found that this “additive” allowed the small grains to fit better between the large ones, and therefore the decoction had a plasticizing effect on the clay. Other authors [28,29] investigated a process for reusing Vitellaria Paradoxa waste as an economical material to improve the manufacture of fired clay bricks. The addition of these wastes practically did not affect the chemical and mineralogical transformations that occur during firing, but made it possible to increase the mechanical resistance and reduce the water absorption of the bricks obtained [29]. Alam et al. in 2015 [22] used alginate (polysaccharides obtained from brown algae) as a stabilizer and Galán-Marin et al. in 2010 [30] used sheep’s wool fibers as reinforcement. The results show that the addition of alginate alone improves the compressive strength of raw earth by 69% (it goes from 2.23 to 3.77) and that the addition of wool alone allows an increase in compressive strength by 37% (it goes from 2.23 to 3.05). When alginate and wool fibers are combined, the compressive strength doubles.
It is in this context that the present work takes place. Its main objective is to experimentally test the use of the decoction products of Parkia Biglobosa and Vitellaria Paradoxa as a natural additive for the production of building materials in stabilized raw earth.
The earth used comes from a lateritic clay formation, commonly called “Terre de Barre” or “Bar Earth”, taken from the Republic of Benin (West Africa), in the Atlantic department, municipality of Abomey-Calavi (6°28′36.52″N 2°19′34.92″E). Previous studies [31] revealed good homogeneity of this material on the Abomey-Calavi plateau. Sampling was carried out in three equidistant wells of 20 m at respective depths of 0.5, 1, and 1.5 m (Fig. 1). The materials were mixed and quartered by standard NF X 31-100 [32]. The soil samples are first dried in an oven to constant weight at 50°C and sieved through a 2 mm sieve before being mixed with water and/or with additives. The Abomey-Calavi Bar Earth was characterized by geotechnical tests (particle size analysis, Atterberg limit, grain density), chemical (X-ray fluorescence), and mineralogical (DRX) tests.
Figure 1: Sampling of bar earth
The decoction waters associated with processing the Vitellaria Paradoxa (shea) seed (Fig. 2) into butter and the Parkia Biglobosa (nere) nut (Fig. 3) into mustard or cheese were used. The choice of these products is justified by:
• the very wide availability of these varieties in sub-Saharan Africa;
• very low CO2 emissions associated with their production and processing;
• the traditional use of decoction water by rural populations in raw clay soil with or without plant fibers for the construction of granaries, the manufacture of bricks, the rendering of huts, and the manufacture of pottery;
• the development in Benin of the Parkia Biglobosa and Vitellaria Paradoxa processing industry generates large quantities of these by-products.
Figure 2: Vitellaria Paradoxa (shea tree)
Figure 3: Parkia Biglobosa (nere)
For Parkia Biglobosa, the cooking water is recovered by boiling 250 grams of raw seeds in 0.50 liters of drinking water. During the cooking time which lasts an average of 6 h, an addition of 250 ml of water is made to constantly maintain the water on the seeds. The residual water of 250 ml is recovered after skimming and recovery of recoverable material.
For Vitellaria Paradoxa, it is the residual water from the manufacture of butter that is recovered. The steps consist of peeling the fruit to recover the almonds, which are crushed, roasted, and crushed into a paste. The paste is churned into a container where lukewarm water is added to bring up the scum and leave waste at the bottom of the container. After skimming the material, the residual water is recovered.
These different waters have been kept in hermetically sealed jars to prevent their degradation over time (generally used within a week).
Five mixtures of raw earth or geomaterials were made. They were obtained by manually mixing the dry earth by the endogenous practice of earthen builders, either with pure water (control mixture T), or with one or other of the pure additives (mixture M4, Parkia Biglobosa P or Vitellaria Paradoxa V), or with a mixture of water and additive in relative proportions of 25%, 50% and 75%, respectively (mixture M1, M2 and M3). Table 1 illustrates the composition of the liquid solution used to shape the mixtures. The quantity of liquid solution used was adjusted to obtain pastes of a consistency comparable to that used in the practice of traditional “banco” constructions in Benin and whose contents are around 30%, well above the optimum Proctor which is around 18%. This consistency was characterized using a pocket penetrometer (Fig. 4), on preparations made by masons on real sites. The average stress measured is around 200 KPa (2 bars).
Figure 4: Pocket penetrometer
The 10 cm × 10 cm × 2 cm and 4 cm × 4 cm × 16 cm type specimens were manufactured from the mixtures thus produced. The mixtures obtained are filled into the molds without compacting other than manually using a spatula to obtain regular faces. Before filling, the interior of the molds was coated with a thin plastic film (Fig. 5) to facilitate immediate de-molding instead of releasing oil which could influence the results of the durability tests. The mold is turned over on support then the various walls of the mold are gently dismantled without damaging the specimen. After removal from the mold, the specimens placed on support are dried in the open air in an enclosure at 20°C. until constant mass.
Figure 5: Mold covered with plastic film
The actual density of the soil sample is determined according to the standard NFEN1097-6, 2001 [33]. It is calculated from the mass/volume ratio. The mass is determined by weighing the saturated test sample, surface dry, and again after drying in an oven. The volume is calculated from the mass of the volume of water displaced, determined by weighing in the case of the pycnometer method.
Organic matter undergoes considerable volume changes under the effect of loadings and variations in moisture content. Some soils, from geologically recent deposits, may contain organic matter. In the laboratory, the overall organic matter content was measured on the residue passing 2 mm, previously dried at 50° to constant mass, which is reacted with hydrogen peroxide. A second steaming allows by difference to know the weight and therefore the organic matter content [34].
The Bar Earth of Abomey Calavi was identified by geotechnical tests, namely: particle size analysis and Atterberg limit. The particle size analysis test consisted in separating the agglomerated grains from a known mass of material by stirring underwater, splitting this soil, once dry, using a series of sieves, and in successfully weighing the cumulative refusal on each sieve [35]. The Atterberg limits (liquidity and plasticity limits) are the weight of water contents corresponding to particular states of soil. They were determined by standard NFP94-051, 1993 [36].
These listed tests make it possible to determine the main characteristics of the soil. Based on these tests, a classification has been proposed according to ASSHO to allow the prediction of the main geotechnical properties of the earth under study.
2.2.3 Chemical and Mineralogical Analysis of Materials
Chemical analysis by X-ray fluorescence spectrometry was used for all raw materials (soil and stabilizers) and mixtures (soil + solution) were performed. This analysis lets us know the nature of the chemical elements present in a sample and their mass concentration. The principle consists in directing a beam of X-rays on the sample to be analyzed. Under the effect of these X-rays, the atoms constituting the sample pass from their ground state to an excited state causing the emission of photons with wavelengths dependent on the chemical element to be analyzed. It is the phenomenon of X-ray fluorescence which is a secondary emission of X-rays, characteristic of the atoms that make up the sample.
The mineralogical analysis was carried out by X-ray diffraction. This technique makes it possible to determine the nature of each crystalline phase within a sample. As part of this study, the materials were characterized using the Bruker D8 Advance diffractometer equipped with a Ge(111) monochromator, making it possible to discriminate the Kα1 wavelength of copper (λ = 1.54056 Å) and the pitch is 0.03°, sweep from 6° to 70° in 2 h 15, 40 kV, 40 mA. This analysis was carried out on the earth material previously reduced to powder by a grinder and on the dry residues of the stabilizers obtained by drying in an oven at 50°C. A volume of the stabilizers until constant weight.
2.2.4 Shrinkage Test and Loss of Mass
The dimensional variation of geomaterials is evaluated by measuring variations in the length of a 4 × 4 × 16 type earth specimen (Fig. 6). The test was carried out based on the French standard NFP15-433, 1994 [37]. To facilitate the demolding of the fresh mixtures and to avoid the use of oil for molding which could modify the properties of the test specimens, the molds were previously covered with a plastic film as shown in the Fig. 5. The measurements of the dimensional variations of the specimens were made using studs inserted at the ends of the specimens (Figs. 6 and 7) during manufacture. The variations are measured at regular times of 4 h during the day; from a time To corresponding to the demolding of the specimens to fully appreciate the evolution of the shrinkage.
Figure 6: 4 × 4 × 16 specimens with inserted studs
Figure 7: Measure of the dimensional variation
The longitudinal shrinkage is determined by the formula:
with, R: shrinkage of the material (10−6 m/m);
L0 = 160 mm initial length (To);
L1: length at measurement time T.
The specimens are weighed before each measurement of the dimensional variation to determine the mass loss of the geomaterial. We obtain the loss of mass by the formula:
where:
m1: initial mass of the specimen (T0);
m2: mass of the specimen at measurement time T.
2.2.5 Compressive Strength Tests
The compressive strength of the geomaterials was carried out by standard NFEN196-1, 1995 [38] and using an IGM-type traction/compression machine in a constant speed mode of 2.4 kN/s, with a capacity of 250 kN and 10 N precision. It is controlled by a computer. The specimens are broken into two more or less equal parts by bending using the press (Fig. 8). Then, the compressive strengths are measured on each of the half-test pieces.
Figure 8: 3 points bending tests
2.2.6 Drip Test and Absorption Tests
The specimens with dimensions of 10 cm × 10 cm × 2 cm were subjected to drip tests according to the procedure described by the CRAterre laboratory manual (6). CRAterre is an earth research and application center. It became a research laboratory on raw earth architecture based at the National School of Architecture of Grenoble (ENSAG) in France. This test consists in subjecting the specimens to regular drops of water falling from a height of 2.5 m (Fig. 9) at the rate of one drop per second until the latter is punctured or deteriorates. The water resistance time of the specimen is noted.
Figure 9: Schema showing the drip test device
These same types of test specimens were partially immersed in water to a height of 5 mm for 10 min to determine the partial absorption capacity of the various mixtures. The total absorption capacity was determined by fully immersing the specimens in water for 10 min, the height of the water above the specimen was at least 5 mm. The water absorption coefficient Cb of each specimen is expressed by the formula:
where:
m1: mass of the specimen before the start of the test;
m2: mass of the specimen after immersion in water;
S: the surface of the submerged face, in square centimeters.
3.1 Characteristics of the Soil Sample from Abomey-Calavi (Southern Benin)
If we designate by TB-Ab-C, the Bar Earth of Abomey-Calavi, the geotechnical characteristics of this earth are given in Table 2. The grain distribution is shown in Fig. 10.
Figure 10: Particle size curve of the earth of Abomey-Calavi
She is composed of particles the grain size of which is less than 2 mm. The percentage of undersized materials obtained by washing with an 80 μm sieve is approximately 18%. The nature of this earth is the moistened sandy clay.
The soil analysis revealed that the organic matter content is 0.9%. It is less than 2% which represents the limit recommended by Taallah [39]. According to the ASSHO classification, which is more suitable for earth construction [6], the earth under study has characteristics that correspond to class A2-7-a.
XRD analysis shows that the Abomey Bar Earth is mainly made up of quartz, kaolinite, hematite, microcline, and albite (Fig. 11).
Figure 11: Abomey-Calavi bar earth diffractogram
However, the analysis of the chemical composition (Table 3) indicates that silica and alumina are the main oxides in the Bar Earth sample from Abomey Calavi. The mass ratio SiO2/Al2O3 is about 4.6. According to Konan, 2006, this value is much higher than that of pure kaolin which is around 1.18 and therefore suggests the presence of free silica and other clay minerals [40]. Observation with an optical microscope (Fig. 12) shows the presence of silica crystals. The iron oxide present in the sample is characteristic of hematite in the soil.
Figure 12: Optical microscope observation of the bar earth of Abomey-Calavi
3.2 Characteristics of Stabilizers Based on Parkia Biglobosa and Vitellaria Paradoxa
The XRD analysis of the dry extract stabilizers based on Parkia Biglobosa and Vitellaria Paradoxa (Fig. 13) revealed that they are composed of organic molecules of the Oxo bis Diphenyl antimony and Vinylheptaphenyltrisiloxane type.
Figure 13: DRX on dry stabilizer residues
The chemical composition of the stabilizers obtained by ion chromatography is shown in Fig. 14. It emerges from this figure that the two types of stabilizers are composed of the same type of sugar, but in different proportions. We note that Ac galactu and Galactose are the dominant sugars of the stabilizer based on Parkia Biglobosa, while the dominant sugars of the stabilizer based on Vitellaria Paradoxa are glucose, Arabinose, and Xylose.
Figure 14: Sugars present in the stabilizers
3.3 Physical and Mechanical Characteristics of Geomaterials
If we designate by T the “adobe” geomaterial control of soil mixture +100% water, Adb10.25 the “adobe” geomaterial of soil mixture +0.25% stabilizer based on Vitellaria Paradoxa and 0.75% water, etc., the physical and mechanical characteristics in particular, final mass loss, final dimensional variation (shrinkage) and compressive strength of the geomaterials developed are presented in Table 4.
To better illustrate the influence of stabilizers on mass loss, shrinkage, and compressive strength, Figs. 15–17 deduced from Table 4, have been drawn.
Figure 15: Mass loss after drying depending on stabilizer dosages
Figure 16: Dimensional variation (shrinkage) after drying depending on stabilizer dosages
Figure 17: Compressive strength of mixtures according to stabilizer dosages
In Fig. 14, we observe that the final loss of mass decreases according to the dosages of Vitellaria Paradoxa and Parkia Biglobosa in the aqueous phase. We note a final mass loss of 18.35% for T and 12.25% for Adb4100 and 11.78% for Adb8100. Compared to T, this represents a reduction of approximately 35% in the loss of mass for a mixture made with 100% stabilizer. It is also noted that the loss of total mass is practically achieved with a dosage of 25% of Parkia Biglobosa in the mixture. For Vitellaria Paradoxa, the loss of mass becomes significant when the aqueous solution contains 75% of this product.
Fig. 15 shows that the shrinkage decreases practically in the same way as a function of the dosages of the two types of stabilizers. A total shrinkage of 4.72% for T, 3.55% for Adb4100, and 3.14% for Adb8100 is observed. Which represents respectively a total decrease of approximately 25% and 33% compared to T.
We note in Fig. 16 an increase in compressive strength with increasing stabilizer dosage. This improvement in resistance is better with the stabilizer of Parkia Biglobosa than with the stabilizer of Vitellaria Paradoxa. The compressive strength goes from 0.97 MPa for T to 1.84 MPa for Adb4100 and 2.56 MPa for Adb8100. This represents a variation of 90% for the Adb4100 sample and 260% for Adb8100.
From the Figs. 15–17, we deduce that the use of stabilizers based on Parkia Biglobosa and Vitellaria Paradoxa instead of water in the mixing of kneaded earth effectively improves the physical properties and mechanical adobes.
By comparing the measured compressive strength values to those of geomaterials studied by Heathcote in 1991 [41] and the normative requirements of the Australian, Mexican, and UNESCO codes [42–44] regarding the performance of stabilized blocks (Table 5), it is obvious that the optimal sample is the adobe “Adb8100”.
3.4 Durability of Geomaterials
To measure that the durability of the formulated adobes is guaranteed, an erosion test was performed (Fig. 9). From the observation of the histogram of Fig. 18, we note that the breaking time of the adobes increases with the increase in the dosage of stabilizer. The addition of admixtures improves the water resistance of adobes.
Figure 18: Resistance time of mixtures to water depending on stabilizer dosages
Indeed, the control adobes perforate after approximately 2.5 h while the Adb8100 adobes have a resistance of up to 12 h, which represents an improvement rate of about 500%.
On the other hand, this improvement is about 46% for the adobe Adb4100 compared to the mixture T. This clearly shows the influence that stabilizers have on the water resistance of formulated geomaterials and more particularly the stabilizer of Vitellaria Paradoxa. These stabilizers play a role in waterproofing because they contribute to the resistance to perforation of water drops.
The performance of adobes against water could be explained by the fact that the stabilizers participate in the good consolidation of the clay plates.
The behavior of adobes in water is also studied by absorption tests. The results of these tests are shown in Figs. 19 and 20.
Figure 19: Absorption coefficient according to stabilizer contents
Figure 20: Total absorption coefficient depending on stabilizer contents
Fig. 19 shows us the shape of the absorption coefficient as a function of the dosage of the stabilizer. We find that there is not a large variation in the absorption coefficient by capillarity for the control samples. However, for the samples with 25% stabilizer, we note a significant absorption rate for all the samples having undergone stabilization.
By observing the curves in Fig. 20, we notice that the “Adb8100” geomaterial behaves better in water compared to the others.
By comparing these different results, it can be seen that the geo-material prepared has better performance for mixtures of 100% stabilizer and better with the stabilizer based on Parkia Biglobosa. But we can also see that the performances begin to be remarkable from mixtures of 50% stabilizer. And as the availability of 100% stabilizer for earthen constructions will be difficult, we recommend the realization of geomaterials a mixture with 50% stabilizer based on Parkia Biglobosa.
The mixtures of Abomey Calavi Bar Earth with the Vitellaria Paradoxa and Parkia Biglobosa stabilizers have proven to be effective because the geomaterials produced have values of mechanical resistance in compression higher than those obtained without the addition of stabilizers. These results are better with Parkia Biglobosa than with Vitellaria Paradoxa. The study of the durability of geomaterials facing water also proved to be more effective with Parkia Biglobosa than with Vitellaria Paradoxa. These results demonstrate the possibility of stabilizing the land with non-timber forest products. In short, the decoctions studied are promising additives for stabilizing the earth in order to be able to use it as a structural material. Thus through this study, we recommend a mixture of 50% stabilizer based on Parkia Biglobosa for the manufacture of geomaterials. Further studies on surface wettability and long-term durability are planned to better characterize the geomaterial. Also, in perspective, it is planned to study the influence of the organic adjuvants studied on the compressed earth bricks for better mechanical resistance.
Acknowledgement: We would like to thank the Benin state for the scholarship granted to an author within the framework of this study.
Funding Statement: This work was supported by the Beninese state. An author received a trainer training grant from the Benin state for stays in the Jean Lamour Institute Laboratory of the University of Lorraine in France to carry out tests.
Author Contributions: The authors confirm contribution to the paper as follows: study conception and design: Cheissou Bio Koto Tamou, Edmond Codjo Adjovi, Mohamed Gibigaye; data collection: Jean-Michel Mechling, Cheissou Bio Koto Tamou, Nicolas Brosse, Gildas Edjrossè Fructueux Godonou; analysis and interpretation of results: Crespin Prudence Yabi, Cheissou Bio Koto Tamou, Gildas Edjrossè Fructueux Godonou; draft manuscript preparation: Crespin Prudence Yabi, Cheissou Bio Koto Tamou, Gildas Edjrossè Fructueux Godonou. All authors reviewed the results and approved the final version of the manuscript.
Availability of Data and Materials: The data that support the findings of this study are available from the corresponding author, upon reasonable request.
Conflicts of Interest: The authors declare that they have no conflicts of interest to report regarding the present study.
References
1. van Damme, H., Houben, H. (2018). Earth concrete. Stabilization revisited. Cement and Concrete Research, 114, 90–102. [Google Scholar]
2. Teixeira, E. R., Machado, G., Junior, A. D. P., Guarnier, C., Fernandes, J. et al. (2020). Mechanical and thermal performance characterisation of compressed earth blocks. Energies, 13(11), 1–22. [Google Scholar]
3. Jannat, N., Hussien, A., Abdullah, B., Cotgrave, A. (2020). Application of agro and non-agro waste materials for unfired earth blocks construction: A review. Construction and Building Materials, 254, 1–29. [Google Scholar]
4. Meimaroglou, N., Mouzakis, C. (2019). Cation exchange capacity (CECtexture, consistency and organic matter in soil assessment for earth construction: The case of earth mortars. Construction and Building Materials, 221, 27–39. [Google Scholar]
5. Ouedraogo, K. A. J. (2019). Stabilization of sustainable and ecological building materials based on raw earth using organic and/or mineral binders with low environmental impacts (Ph.D. Thesis). Toulouse Midi-Pyrénées Federal University, France. [Google Scholar]
6. Houben, H., Guillaud, H. (2006). Traité de construction en terre. Marseille: Parenthèse. CRATerre. [Google Scholar]
7. Little, B., Morton, T. (2007). Building with earth in Scotland. In: Innovative design and sustainability. Edinburgh: Scottish Executive Central Research Unit. [Google Scholar]
8. Bide, T., Novellino, A., Petavratzi, E., Watson, C. S. (2023). A bottom-up building stock quantification methodology for construction minerals using earth observation. The case of Hanoi. Cleaner Environmental Systems, 8, 1–15. [Google Scholar]
9. Paula Junior, A. C., Jacinto, C., Turco, C., Fernandes, J., Teixeira, E. et al. (2022). Analysis of the effect of incorporating construction and demolition waste on the environmental and mechanical performance of earth-based mixtures. Construction and Building Materials, 330, 1–21. [Google Scholar]
10. Ouedraogo, K. A. J., Aubert, J. E., Tribout, C., Millogo, Y., Escadeillas, G. (2021). Ovalbumin as a natural organic binder for stabilizing unfired earth bricks: Understanding vernacular techniques to inspire modern constructions. Journal of Cultural Heritage, 50, 139–149. [Google Scholar]
11. Banakinao, S., Tiem, S., Attipou, K., Novinyo, K., Lolo, K. et al. (2017). Use of the nere pod (Parkia Biglobosa) for the improvement of mechanical properties of soils. American Journal of Applied Sciences, 14, 302–308. [Google Scholar]
12. Milohin, G. (2019). Valorization of glass bottle waste and wood ash in the manufacture of fired clay bricks (Ph.D. Thesis). University of Lorraine (FranceUniversity of Abomey Calavi (Benin). [Google Scholar]
13. Islam, M. S., Elahi, T. E., Shahriar, A. R., Mumtaz, N. (2020). Effectiveness of fly ash and cement for compressed stabilized earth block construction. Construction and Building Materials, 259, 1–26. [Google Scholar]
14. El Bourki, A., Koutous, A., Hilali, E. (2023). A review on the use of date palm fibers to reinforce earth-based construction materials. Materials Today: Proceedings, pp. 1–10. https://doi.org/10.1016/j.matpr.2023.05.613 [Google Scholar] [CrossRef]
15. Ma, C., Xie, Y., Long, G., Chen, B., Chen, L. (2017). Effects of fly ash on mechanical and physical properties of earth-based construction. Construction and Building Materials, 157(1), 1074–1083. [Google Scholar]
16. Nadeem, M., Gul, A., Bahrami, A., Azab, M., Wali, S. (2023). Results in engineering evaluation of mechanical properties of cored interlocking blocks–A step toward affordable masonry material. Results in Engineering Journal, 18, 1–11. [Google Scholar]
17. Dao, K., Ouedraogo, M., Millogo, Y., Aubert, J. E., Gomina, M. (2018). Thermal, hydric and mechanical behaviors of adobes stabilized with cement. Construction and Building Materials, 158, 84–96. [Google Scholar]
18. Seco, A., Urmeneta, P., Prieto, E., Marcelino, S., García, B. et al. (2017). Estimated and real durability of unfired clay bricks: Determining factors and representativeness of the laboratory tests. Construction and Building Materials, 131(133–134), 600–605. [Google Scholar]
19. Zerbo, L., Sorgho, B., Bressollier, P., Ouedraogo, R., Gomina, M. et al. (2016). Study of the mechanical properties of clay geomaterials containing the decoction of Parkia Biglobosa (néré). Chemistry Reports, 19, 895–901. [Google Scholar]
20. Losini, A. E., Grillet, A. C., Bellotto, M., Woloszyn, M., Dotelli, G. (2020). Natural additives and biopolymers for raw earth construction stabilization–A review. Construction and Building Materials, 304, 1–11. [Google Scholar]
21. Koutous, A., Hilali, E. (2019). Grain shape effects on the mechanical behavior of compacted earth. Case Studies in Construction Materials, 11, e00303. [Google Scholar]
22. Alam, I., Naseer, A., Shah, A. A. (2015). Economical stabilization of clay for earth buildings construction in rainy and flood-prone areas. Construction and Building Materials, 77(1), 154–159. [Google Scholar]
23. Anglade, E., Aubert, J. E., Sellier, A., Papon, A. (2022). Physical and mechanical properties of clay-sand mixes to assess the performance of earth construction materials. Journal of Building Engineering, 51(4), 1–15. [Google Scholar]
24. Medvey, B., Dobszay, G. (2020). Durability of stabilized earthen constructions: A review. Geotechnical and Geological Engineering, 38(3), 2403–2425. [Google Scholar]
25. Sorgho, B., Zerbo, L., Keita, I., Dembele, C., Plea, M. et al. (2013). Strength and creep behavior of geomaterials for building with tannin addition. Materials and Structures, 47(6), 937–946. [Google Scholar]
26. Banakinao, S., Tiem, S., Lolo, K. (2016). Dataset of the use of tannin of néré (Parkia-Biglobosa) as a solution for the sustainability of soil constructions in West Africa. Data in Brief, 8(2), 474–483. [Google Scholar] [PubMed]
27. Koutous, A., Hilali, E. (2021). Reinforcing rammed earth with plant fibers: A case study. Science Direct, 14(1), 1–19. [Google Scholar]
28. Adazabra, A. N., Viruthagiri, G., Shanmugam, N. (2017). Management of spent shea waste: An instrumental characterization and valorization in clay bricks construction. Waste Management, 64, 286–304. [Google Scholar] [PubMed]
29. Adazabra, A. N., Viruthagiri, G., Kannan, P. (2017). Influence of spent shea waste addition on the technological properties of fired clay bricks. Journal of Building Engineering, 11(9), 166–177. [Google Scholar]
30. Galán-marín, C., Rivera-gómez, C., Petric, J. (2010). Clay-based composite stabilized with natural polymer and fiber. Construction and Building Materials, 24(8), 1462–1468. [Google Scholar]
31. Gbaguidi, V., Zevounou, C., Houeto, A., Houessou, A., Vedogbeton, N. et al. (2015). Research and characterization project on bar soil and alterites and their self-locking agglomerates in stabilized soil. OBRGM; LERGC; ECCO-GC. [Google Scholar]
32. NF X 31-100 (2020). Sampling-method for taking soil samples for physico-chemical analysis. AFNOR. [Google Scholar]
33. NFEN1097-6 (2001). Real density and water absorption. Museum, 4, 155–217. [Google Scholar]
34. NFP94-055 (1993). Organic matter content of a soil. [Google Scholar]
35. NFP94-056 (1996). Particle size analysis. AFNOR. [Google Scholar]
36. NFP94-051 (1993). Determination of Atterberg limits. AFNOR. [Google Scholar]
37. NFP15-433 (1994). Test methods for cements-Determination of shrinkage and swelling. AFNOR. [Google Scholar]
38. NFEN196-1 (1995). Cement testing methods determination of mechanical strength. AFNOR. [Google Scholar]
39. Taallah, B. (2014). Study of the physico-mechanical behavior of compressed earth blocks with fibres (Ph.D. Thesis). Mohamed Khider University-Biskra. [Google Scholar]
40. Konan, K. L. (2006). Interction between clay materials and a basic medium rich in calcium (Ph.D. Thesis). University of Limoge. [Google Scholar]
41. Heathcote, K. (1991). Compressive strength of cement stabilized pressed earth blocks. Building Research & Information, 19, 101–105. [Google Scholar]
42. Australian Standard 3700 (1998). Masonry in buildings (known as the SAA Masonry Code). [Google Scholar]
43. Issuing Agency (2015). Housing and construction: 2015 New Mexico commercial building code, pp. 1–15. Construction Industries Division (CIDState Records Center and Archives (SRCA). [Google Scholar]
44. Unido International Labour Office (1987). Small-scale manufacture of stabilized soil blocks, pp. 1–186. Vienna, United Nation. [Google Scholar]
Cite This Article
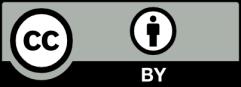
This work is licensed under a Creative Commons Attribution 4.0 International License , which permits unrestricted use, distribution, and reproduction in any medium, provided the original work is properly cited.