Open Access
ARTICLE
Silica Gel from Chemical Glass Bottle Waste as Adsorbent for Methylene Blue: Optimization Using BBD
Chemistry Department, Faculty of Science and Analytical Data, Institut Teknologi Sepuluh Nopember, Surabaya, Indonesia
* Corresponding Author: Yatim Lailun Ni’mah. Email:
(This article belongs to the Special Issue: Special Issue in Celebration of JRM 10 Years)
Journal of Renewable Materials 2023, 11(12), 4007-4023. https://doi.org/10.32604/jrm.2023.031210
Received 22 May 2023; Accepted 22 August 2023; Issue published 10 November 2023
Abstract
This research focuses on the effective removal of methylene blue dye using silica gel synthesized from chemical glass bottle waste as an environmentally friendly and cost-effective adsorbent. The adsorption process was optimized using Box-Behnken Design (BBD) and Response Surface Methodology (RSM) to investigate the influence of pH (6; 8 and 10), contact time (15; 30 and 45 min), adsorbent mass (30; 50 and 70 mg), and initial concentration (20; 50 and 80 mg/L) of the adsorbate on the adsorption efficiency. The BBD was conducted using Google Colaboratory software, which encompassed 27 experiments with randomly assigned combinations. The silica gel synthesized from chemical glass bottle was characterized by XRD, FTIR, SEM-EDX and TEM. The adsorption result was measured by spectrophotometer UV-Vis. The optimized conditions resulted in a remarkable methylene blue removal efficiency of 99.41%. Characterization of the silica gel demonstrated amorphous morphology and prominent absorption bands characteristic of silica. The Langmuir isotherm model best described the adsorption behavior, revealing chemisorption with a monolayer coverage of methylene blue on the adsorbent surface, and a maximum adsorption capacity of 82.02 mg/g. Additionally, the pseudo-second-order kinetics model indicated a chemisorption mechanism during the adsorption process. The findings highlight the potential of silica gel from chemical glass bottle waste as a promising adsorbent for wastewater treatment, offering economic and environmental benefits. Further investigations can explore its scalability, regenerability, and reusability for industrial-scale applications.Graphic Abstract
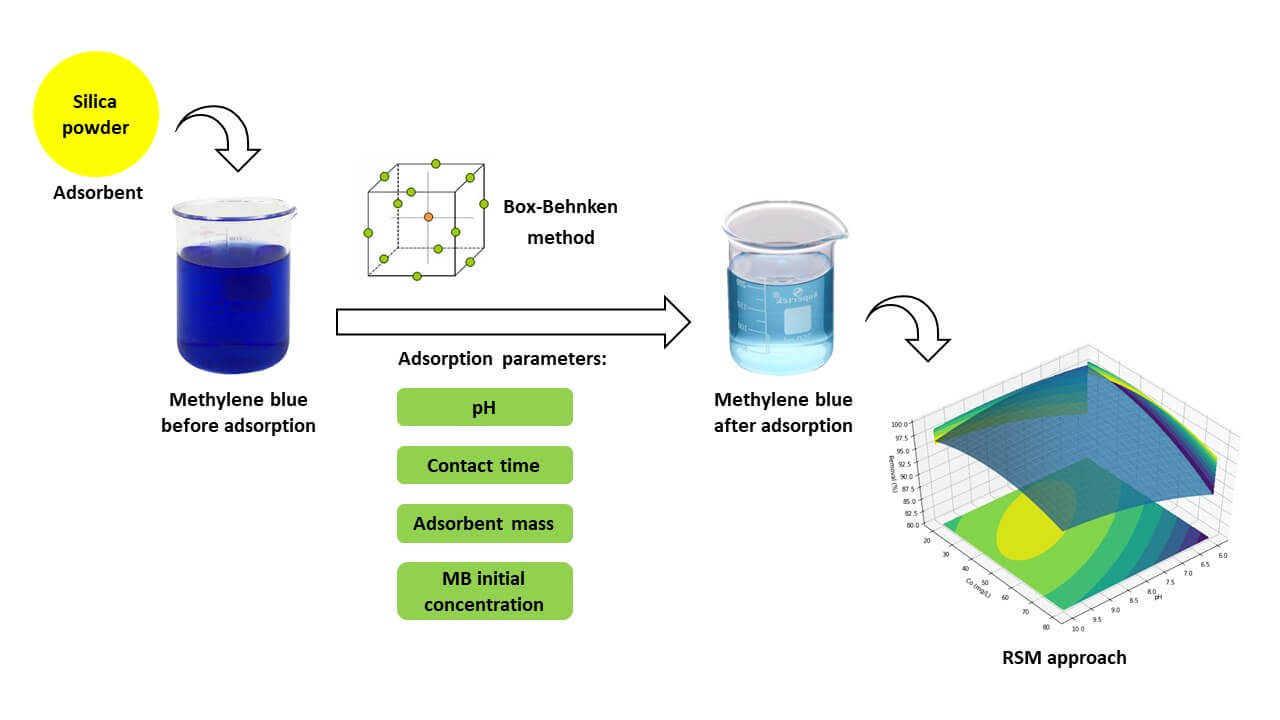
Keywords
The volume of liquid waste containing dyes is escalating rapidly due to its widespread use in various industries [1]. Methylene blue, extensively employed in sectors such as textiles, pharmaceuticals, paper, plastics, rubber, leather, and cosmetics [2], poses considerable health risks, including central nervous system disorders, high blood pressure, skin and throat irritation, nausea, vomiting, and headaches [3,4]. Additionally, its presence in aquatic ecosystems is undesirable due to its inhibitory impact on aquatic flora and fauna activities [5]. The acceptable maximum concentration of methylene blue is 5 mg/kg [3]. Several techniques have been applied to reduce methylene blue concentrations in water, including electrocoagulation [6], ozonation [7], photodegradation [8], membrane processes [9], and bio-removal [10]. Among these, the adsorption technique stands out as the most advantageous due to its simplicity, cost-effectiveness, regenerability, and minimal chemical residue production [11]. Numerous studies have explored the adsorption of methylene blue [12–15].
Silica gel is a widely used adsorbent in wastewater treatment, owing to its intriguing properties such as pore size and large surface area [16]. Traditionally, silica gel is synthesized from commercial alkoxides using the sol-gel method [17]. While this approach offers advantages like high purity and precise control over properties through composition adjustments [18,19], the use of commercial alkoxides can be expensive and potentially hazardous. In this context, inorganic waste, such as glass waste, presents a promising alternative. Glass bottle waste contains a high amount of silica (60%–80%) [20], making it an attractive source for synthesizing silica gel at a low cost and with minimal environmental impact. Prior studies have successfully employed glass bottle waste for synthesizing silica gel using the sol-gel method [21,22], offering economic and eco-friendly benefits. Additionally, Table 1 showcases various materials employed for methylene blue adsorption. Table 1 presents a comprehensive overview of several materials employed for the adsorption of methylene blue, a commonly used dye in various industrial applications. The adsorption capacity, expressed in milligrams per gram (mg/g), is used as a measure of the efficiency of each adsorbent in removing methylene blue from aqueous solutions.
Table 1 demonstrates the proficient capacity of peanut husk to capture and eliminate methylene blue molecules from solutions. The magnetic graphene-carbon nanotube showcases its efficacy in methylene blue removal. Utilizing untreated Metroxylon spp. waste as adsorbent shows promise as a potent contender for effective dye removal. Conversely, hydrothermal carbonization of coffee husk displays a relatively diminished adsorption capacity, indicative of its moderate efficiency in methylene blue adsorption. Furthermore, kaolin, a natural clay material, presents the potential to serve as an adsorbent for methylene blue.
The adsorption process is influenced by various factors, which can be optimized using Box-Behnken Design (BBD) and analyzed through Response Surface Methodology (RSM). BBD, a nearly rotatable and non-factorial design [29], requires 3 levels for each factor, represented as −1, 0, and +1. By utilizing BBD, the interactions between factors can be studied, and the number of experiments can be reduced [30]. For instance, Çetintaş [3] used BBD with 3 factors to study methylene blue adsorption, achieving a removal efficiency of 98% and an adsorption capacity of 3.07% (using an adsorbent mass of 0.8 g, pH 5.2, and initial adsorbate concentration of 50 mg/L).
In this study, we optimized the adsorption of methylene blue using Box-Behnken Design (BBD) with four factors: pH, contact time, adsorbent mass, and initial concentration of the adsorbate. Silica gel derived from chemical glass bottle waste served as the adsorbent. To characterize the silica gel, various techniques such as X-Ray Diffraction (XRD), Fourier Transform-Infrared Spectroscopy (FTIR), N2-physisorption (BET), Scanning Electron Microscope-Energy Dispersive X-Ray (SEM-EDX), and Transmission Electron Microscopy (TEM) were employed. Our research aimed to evaluate the efficacy of silica gel in adsorbing methylene blue using BBD. Both kinetic and isotherm models were examined to understand the adsorption behavior of methylene blue on silica gel. Through this study, we strive to contribute to the advancement of effective and sustainable methods for methylene blue removal from wastewater.
2.1 Chemical Glass Bottles Preparation
Chemical glass bottle waste was collected from the waste disposal site in the Chemistry Department, ITS, Surabaya, Indonesia. To prepare the waste for further use, the glass bottles underwent a thorough cleaning process with water until they were completely free of impurities. Subsequently, the cleaned glass bottles were dried and then crushed using a jaw crusher. The crushed glass material was further milled for 2 min to achieve a fine powder consistency. To ensure uniformity, the resulting powder was sieved using 200 and 230 mesh screens [22].
The synthesis method utilized in this study follows the procedure previously reported by Ni’mah et al. [22]. Initially, chemical glass bottle waste powder was combined with NaOH in a 1:3 (w/w) ratio, followed by calcination at 800°C for 4 h. Sodium silicate solutions were obtained by dissolving sodium silicate in boiling water and then filtering. Subsequently, a 3M HCl solution was added dropwise to the stirred solutions until a white gel was formed. The resulting white gel was left to age for 18 h, after which it was filtered and washed with deionized water until a neutral pH was achieved. The obtained silica gel was then dried in an oven at 80°C for 12 h.
2.3 Preparation of Methylene Blue
Methylene blue, obtained from Merck (C.I. 52015; Molar Mass: 319.86 g/mol, anhydrous), was used in this study. To create methylene blue solutions with varying concentrations, a stock solution of 500 mg/L was prepared and then diluted accordingly.
2.4 Adsorption Studies (Box-Behnken Design)
In this study, a 50 mL methylene blue solution was combined with the adsorbent at room temperature and stirred at 450 rpm. The adsorption process was investigated using Box-Behnken Design (BBD) to analyze the impact of various factors, including pH, contact time (t), adsorbent mass (m), and initial concentration of the adsorbate (Co). The BBD was conducted using Google Colaboratory software, which encompassed 27 experiments with randomly assigned combinations, as outlined in Table 2.
Following the adsorption process, the mixture was subjected to centrifugation at 3500 rpm for 10 min. The concentration of methylene blue was then measured using a UV-Vis spectrophotometer at a wavelength of 665 nm. The regression equation for methylene blue (R2 = 0.9964) is shown in Eq. (2.1).
The removal of methylene blue using silica gel synthesized from chemical glass bottles was determined using Eq. (2.2).
where Co (mg/L) and Ce (mg/L) represent the initial and equilibrium concentrations of the methylene blue solution, respectively.
The correlation between independent and dependent variables is represented by the second-order polynomial equation, given as Eq. (2.3).
In this equation, Y denotes the dependent variable (response), β0 stands for the constant coefficient, while βi, βii, and βij represent the linear, quadratic, and interaction coefficients, respectively. Xi and Xj are the independent variables, and ε represents the experimental error.
To explore the adsorption mechanism, this study employed the Langmuir, Freundlich, Temkin, and Dubinin-Radushkevich isotherm models. The concentration of the methylene blue solution varied within the range of 20 to 80 mg/L. Furthermore, the adsorption kinetics model was examined using the pseudo-first-order and pseudo-second-order models. The contact time was varied at 15, 20, 25, 30, 35, 40, and 45 min.
Fig. 1 displays the XRD diffractogram of the silica gel. A broad peak appears at 2θ = 22.8°, suggesting that the silica gel phase is amorphous. This observation aligns closely with previous research, where silica gel commonly exhibits broad peaks within the range of 2θ = 20°–30° [12,22].
Figure 1: X-ray diffractogram of silica gel
Fig. 2 presents the FTIR spectra of the chemical glass bottle powder and silica gel. In both materials, specific absorption bands are observed: at 400–450, 700–780, and 1000–1050 cm−1, which correspond to the O-Si-O bending vibration, Si-O-Si symmetric stretching vibration, and Si-O-Si asymmetric stretching vibration, respectively. These absorption bands are characteristic of silica and are commonly observed [31]. Additionally, both spectra display absorption bands at 1600–1700 cm−1, indicating the presence of H-O-H bending vibration and suggesting the existence of H2O molecules in both materials. The broad absorption band observed at 3400–3600 cm−1 corresponds to the stretching vibrations of O-H, signifying a substantial number of hydroxyl groups on the pore surfaces of both materials.
Figure 2: FTIR spectra of bottle glass and silica gel
The chemical glass bottle powder exhibits a distinct absorption band at 1400–1500 cm−1, which indicates the presence of B-O stretching vibrations in the boron oxide structure. This observation is consistent with the fact that chemical glass bottles belong to the category of borosilicate glass, which contains boron oxide. However, the B-O absorption band is not present in the FTIR spectra of silica gel due to the sodium silicate filtration process. The FTIR spectra in this research show similarities with previous studies conducted by Ni’mah et al. [22].
The TEM analysis results presented in Figs. 3a and 3b indicate that the silica gel particles exhibit a spherical shape with an average size below 50 nm, and they tend to agglomerate, forming clusters of particles. These observations align with previous studies conducted by Hoang et al. [32], who also reported similar characteristics of silica gel particles with a spherical shape and agglomeration tendency. Moreover, the TEM analysis revealed no significant difference between the morphology of silica gel before and after the adsorption process. This suggests that the adsorption of methylene blue did not cause substantial changes in the overall structure and shape of the silica gel particles.
Figure 3: TEM of silica gel (a) before adsorption and (b) after adsorption
The SEM-EDX analysis, as shown in Figs. 4a and 4b, demonstrated slight differences in the morphology of the silica gel before and after MB adsorption. The EDX results revealed that the major elements in the material before adsorption were Si and O. However, after adsorption, the intensity of the carbon element surpassed that of Si and O. These findings strongly suggested that MB was successfully adsorbed and occupied the surface of the silica gel. This observation aligns with the previous study conducted by Ni’mah et al. [22].
Figure 4: SEM-EDX of silica gel (a) before adsorption and (b) after adsorption
3.2 Optimization of Methylene Blue Adsorption
In this study, the optimal conditions for methylene blue adsorption were determined by investigating multiple independent factors, namely pH, contact time, adsorbent mass, and initial adsorbate concentration, using the Box-Behnken Design (BBD) and analyzed through Response Surface Methodology (RSM). The experimental and predicted values of methylene blue removal are presented in Table 3. The highest observed methylene blue removal in the experiments reached 99.06%, while the maximum predicted removal from the BBD model was 100.71%.
The regression equation for methylene blue removal in this research was expressed in Eq. (3.1).
where R is the removal of methylene blue (%), X0 is pH, X1 is contact time, X2 is adsorbent mass, and X3 is the initial concentration of adsorbate.
Fig. 5a presents the correlation between the predicted and experimental values of methylene blue removal. The residuals in Fig. 5b appear as random errors, indicating a good fit between the predicted and observed data. The density plot in Fig. 5c shows a bell-shaped curve with the peak centered around 0, suggesting that the residuals follow a normal distribution. These findings collectively indicate the suitability of the regression model for optimizing methylene blue adsorption.
Figure 5: (a) The regression curve of predicted and experiment removal of methylene blue, (b) residuals values, and (c) density plot
The statistical validation of methylene blue adsorption optimization was performed using Ordinary Least Square (OLS) regression analysis, and the results are presented in Table 4. The obtained p-value for the model was 0.000933, indicating that the input variables significantly influenced the output variable for methylene blue adsorption (p-value < 0.05). Furthermore, the coefficient of determination (R2) between the model and experimental data was 0.889, demonstrating a strong correlation between the experimental results and the predictions of the model.
The RSM plots (Figs. 6a–6f) revealed pH as a significant factor influencing the surface charge distribution of the adsorbent [33]. The optimal pH for methylene blue removal using silica gel from chemical glass bottle waste was found to be 8. Silica gel possesses a pH value of 2.8–3, and at pH values above 3, the silica surface becomes negatively charged, favoring the attraction of cationic species like methylene blue [34]. In alkaline conditions, Si-O- becomes the dominant functional group on the adsorbent surface, resulting from the deprotonation of Si-OH, leading to enhanced electrostatic interaction between methylene blue (a cationic dye) and the adsorbent (anionic charged surface) [3].
Figure 6: The RSM plots of methylene blue adsorption as a function of (a) MB initial concentration vs. pH, (b) adsorbent mass vs. pH, (c) contact time vs. pH, (d) MB initial concentration vs. contact time, (e) adsorbent mass vs. contact time, and (f) MB initial concentration vs. adsorbent mass
The contact time during the adsorption process significantly influences the removal efficiency, as longer contact times lead to increased adsorption of the adsorbate. The RSM plot considering the interaction between contact time and adsorbent mass revealed two optimal regions. However, taking into account the interaction between contact time, pH, and the initial concentration of the adsorbate, the optimal contact time was determined to be 45 min. The mass of the adsorbent used in the adsorption process affects the availability of active surface sites for adsorbate molecules. The optimization results for adsorbent mass and initial concentration of the adsorbate in this research were 60 mg and 40 mg/L, respectively.
Based on the obtained adsorption optimization results, the methylene blue removal reached 99.41%. This removal efficiency surpassed that reported by Çatlıoğlu et al. [35], where they achieved 91.89% methylene blue removal using banana peel as the adsorbent. Moreover, the methylene blue removal in this research exceeded the findings of Çetintaş [3], who employed leaching residues as the adsorbent.
The adsorption isotherm models are valuable tools for elucidating the adsorption mechanism, assessing the maximum adsorption capacity, and understanding the characteristics of the adsorbent [36]. In this study, four adsorption isotherm models, namely Langmuir, Freundlich, Temkin, and Dubinin-Radushkevich, were employed to investigate the adsorption mechanism of methylene blue. The Langmuir isotherm equation is represented by Eq. (3.2).
where qe is the amount of the methylene blue adsorbed at equilibrium (mg/g), qm is the maximum adsorption capacity (mg/g), and KL is the Langmuir constant (L/mg).
The Freundlich isotherm equation is expressed as Eq. (3.3).
where KF is Freundlich’s constant (L/mg) and 1/n is a parameter showing the surface heterogeneity.
The Temkin isotherm equation is expressed as Eq. (3.4).
where R is the gas constant (J/molK), T is the temperature (K), b is the adsorption heat constant (J/mol), and KT is the Temkin constant (L/mg).
The Dubinin-Radushkevich isotherm equation is expressed as Eqs. (3.5) and (3.6).
where ε is the adsorption potential based on Polanyi theory (kJ/mol) and KDR is the Dubinin-Radushkevich constant (mol2kJ−1).
The parameters obtained from fitting the adsorption isotherms (Figs. 7a–7d) are presented in Table 5. The results indicate that the R2 value for the Langmuir isotherm model (0.98) was higher compared to the Freundlich (0.63), Temkin (0.79), and Dubinin-Radushkevich (0.52) models. Therefore, the adsorption data in this study followed the Langmuir isotherm model. The Langmuir isotherm model suggests that the adsorption mechanism involves chemisorption or chemical interactions between the adsorbent and the adsorbate during the adsorption process, and it also indicates that adsorption occurs in a monolayer [37]. The maximum adsorption capacity of silica from chemical glass bottle waste, based on the Langmuir isotherm model, was determined to be 82.02 mg/g.
Figure 7: (a) Langmuir, (b) Freundlich, (c) Temkin, and (d) Dubinin-Radushkevich
Adsorption kinetics is a crucial factor in understanding the rate and mechanism of adsorption, directly impacting the efficiency and application of an adsorbent [38]. In this study, the adsorption kinetics were investigated using the pseudo-first-order and pseudo-second-order models. The pseudo-first-order equation is represented by Eq. (3.7).
where qe is the amount of the methylene blue adsorbed at equilibrium (mg/g), qt is the amount of methylene blue adsorbed at t time (mg/g), k1 is the pseudo-first-order rate constant (minute−1), and t is time (minutes).
The pseudo-second-order equation is expressed as Eq. (3.8).
where k2 is the pseudo-second-order rate constant (minute−1).
The parameters obtained from fitting the adsorption kinetics data (Figs. 8a and 8b) are summarized in Table 6. The results reveal that the pseudo-second-order kinetics model exhibits a higher R2 value (0.99) compared to the pseudo-first-order kinetics (0.04). This finding leads to the conclusion that the adsorption of methylene blue using silica gel from chemical glass bottle waste follows the pseudo-second-order kinetics. The pseudo-second-order kinetics model indicates that the adsorption process involves chemisorption [37].
Figure 8: (a) Pseudo-first-order and (b) Pseudo-second-order
The uptake of methylene blue (MB) involves adsorption mechanisms that apply to both non-porous and porous solids. One hypothesis suggests that the adsorption of MB molecules might take place in a solitary phase, marked by the rapid attainment of equilibrium on the adsorbent’s surface, as shown in Fig. 9. Moreover, a more gradual phase could involve the infiltration of MB into pores and its interaction with binding sites characterized by higher energy levels [39].
Figure 9: Adsorption mechanisms for methylene blue uptake in non-porous and porous solids [39]
In conclusion, this research demonstrated the successful application of silica gel synthesized from chemical glass bottle waste as an effective adsorbent for the removal of methylene blue dye. The adsorption process was optimized using the Box-Behnken Design (BBD) and Response Surface Methodology (RSM) to determine the key factors influencing the adsorption efficiency. The optimized conditions for methylene blue adsorption were found to be at pH 8, with a contact time of 45 min, an adsorbent mass of 60 mg, and an initial concentration of adsorbate at 40 mg/L, resulting in a remarkable removal efficiency of 99.41%.
Characterization of the synthesized silica gel revealed amorphous morphology with prominent absorption bands characteristic of silica. The Langmuir isotherm model was found to best describe the adsorption behavior, indicating a chemisorption process and a monolayer coverage of methylene blue on the adsorbent surface. The maximum adsorption capacity obtained from the Langmuir isotherm model was determined to be 82.02 mg/g, further confirming the high adsorption potential of the silica gel. The kinetics study showed that the adsorption of methylene blue followed the pseudo-second-order kinetics model, suggesting a chemisorption mechanism during the adsorption process.
Overall, the utilization of silica gel derived from chemical glass bottle waste as an adsorbent offers economic and environmental advantages. The results of this study contribute valuable insights into the potential application of silica gel as an efficient and sustainable adsorbent for the removal of methylene blue dye from wastewater. Future studies can further explore the scalability of this adsorbent on a larger industrial scale and investigate its performance under diverse wastewater conditions. Additionally, exploring its renewability and reusability for multiple adsorption cycles can provide essential information for practical applications in wastewater treatment.
Acknowledgement: The authors thank Directorate of Research and Community Service (DRPM, Direktorat Riset dan Pengabdian Kepada Masyarakat) ITS for their support.
Funding Statement: This research was funded by Directorate of Research and Community Service (DRPM, Direktorat Riset dan Pengabdian Kepada Masyarakat) ITS through the ITS Research Local Grant (No. 1727/PKS/ITS/2023).
Author Contributions: The authors confirm contribution to the paper as follows: study conception and design: Yatim Lailun Ni’mah and Suprapto Suprapto; data collection: Putri Augista Nur Azizah; analysis and interpretation of results: Yatim Lailun Ni’mah and Suprapto Suprapto; draft manuscript preparation: Putri Augista Nur Azizah. All authors reviewed the results and approved the final version of the manuscript.
Availability of Data and Materials: The authors confirm that the data supporting the findings of this study are available within the article.
Conflicts of Interest: The authors declare that they have no conflicts of interest to report regarding the present study.
References
1. Crini, G. (2006). Non-conventional low-cost adsorbents for dye removal: A review. Bioresource Technology, 97(9), 1061–1085. https://doi.org/10.1016/j.biortech.2005.05.001 [Google Scholar] [PubMed] [CrossRef]
2. Mohammed, M. A., Shitu, A., Ibrahim, A. (2014). Removal of methylene blue using low cost adsorbent: A review. Research Journal of Chemical Sciences, 4(1), 91–102. [Google Scholar]
3. Çetintaş, S. (2021). An alternative application for reuse of leaching residues: Determination of adsorption behaviour for methylene blue and process optimization. Sustainable Chemistry and Pharmacy, 23(8), 100504. https://doi.org/10.1016/j.scp.2021.100504 [Google Scholar] [CrossRef]
4. Mashkoor, F., Nasar, A. (2020). Magsorbents: Potential candidates in wastewater treatment technology—a review on the removal of methylene blue dye. Journal of Magnetism and Magnetic Materials, 500(5790), 166408. https://doi.org/10.1016/j.jmmm.2020.166408 [Google Scholar] [CrossRef]
5. Begum, R., Najeeb, J., Sattar, A., Naseem, K., Irfan, A. et al. (2019). Chemical reduction of methylene blue in the presence of nanocatalysts: A critical review. Reviews in Chemical Engineering, 36(6), 749–770. https://doi.org/10.1515/revce-2018-0047 [Google Scholar] [CrossRef]
6. Duan, X. (2018). Application of modified electrocoagulation for efficient color removal from synthetic methylene blue wastewater. International Journal of Electrochemical Science, 13(6), 5575–5588. https://doi.org/10.20964/2018.06.15 [Google Scholar] [CrossRef]
7. Zhang, J., Lee, K. H., Cui, L., Jeong, T. (2009). Degradation of methylene blue in aqueous solution by ozone-based processes. Journal of Industrial and Engineering Chemistry, 15(2), 185–189. https://doi.org/10.1016/j.jiec.2008.09.014 [Google Scholar] [CrossRef]
8. Singh, J., Chang, Y. Y., Koduru, J. R., Yang, J. K. (2018). Potential degradation of methylene blue (MB) by nano-metallic particles: A kinetic study and possible mechanism of MB degradation. Environmental Engineering Research, 23(1), 1–9. https://doi.org/10.4491/eer.2016.158 [Google Scholar] [CrossRef]
9. Li, Q., Li, Y., Ma, X., Du, Q., Sui, K. et al. (2017). Filtration and adsorption properties of porous calcium alginate membrane for methylene blue removal from water. Chemical Engineering Journal, 316, 623–630. https://doi.org/10.1016/j.cej.2017.01.098 [Google Scholar] [CrossRef]
10. Cittrarasu, V., Balasubramanian, B., Kaliannan, D., Park, S., Maluventhan, V. et al. (2019). Biological mediated Ag nanoparticles from Barleria longiflora for antimicrobial activity and photocatalytic degradation using methylene blue. Artificial Cells, Nanomedicine, and Biotechnology, 47(1), 2424–2430. https://doi.org/10.1080/21691401.2019.1626407 [Google Scholar] [PubMed] [CrossRef]
11. Al-Sabagh, A. M., Moustafa, Y. M., Hamdy, A., Killa, H. M., Ghanem, R. T. M. et al. (2018). Preparation and characterization of sulfonated polystyrene/magnetite nanocomposites for organic dye adsorption. Egyptian Journal of Petroleum, 27(3), 403–413. https://doi.org/10.1016/j.ejpe.2017.07.004 [Google Scholar] [CrossRef]
12. Dhmees, A. S., Khaleel, N. M., Mahmoud, S. A. (2018). Synthesis of silica nanoparticles from blast furnace slag as cost-effective adsorbent for efficient azo-dye removal. Egyptian Journal of Petroleum, 27(4), 1113–1121. https://doi.org/10.1016/j.ejpe.2018.03.012 [Google Scholar] [CrossRef]
13. Peres, E. C., Slaviero, J. C., Cunha, A. M., Hosseini-Bandegharaei, A., Dotto, G. L. (2018). Microwave synthesis of silica nanoparticles and its application for methylene blue adsorption. Journal of Environmental Chemical Engineering, 6(1), 649–659. https://doi.org/10.1016/j.jece.2017.12.062 [Google Scholar] [CrossRef]
14. Han, X., Wang, Y., Zhang, N., Meng, J., Li, Y. et al. (2021). Facile synthesis of mesoporous silica derived from iron ore tailings for efficient adsorption of methylene blue. Colloids and Surfaces A: Physicochemical and Engineering Aspects, 617(5), 126391. https://doi.org/10.1016/j.colsurfa.2021.126391 [Google Scholar] [CrossRef]
15. Meechai, T., Poonsawat, T., Limchoowong, N., Laksee, S., Chumkaeo, P. et al. (2023). One-pot synthesis of iron oxide—gamma irradiated chitosan modified SBA-15 mesoporous silica for effective methylene blue dye removal. Heliyon, 9(5), e16178. https://doi.org/10.1016/j.heliyon.2023.e16178 [Google Scholar] [PubMed] [CrossRef]
16. Czarnobaj, K. (2008). Preparation and characterization of silica xerogels as carriers for drugs. Drug Delivery, 15(8), 485–492. https://doi.org/10.1080/10717540802321495 [Google Scholar] [PubMed] [CrossRef]
17. Prasad, T., Halder, S., Dhar, S. S. (2020). Process parameter effects on particle size reduction of sol-gel synthesized silica nanoparticles. Materials Today: Proceedings, 22, 1669–1675. https://doi.org/10.1016/j.matpr.2020.02.184 [Google Scholar] [CrossRef]
18. Pierre, A. C. (2020). Introduction to sol-gel processing. Switzerland AG: Springer Nature. https://doi.org/10.1007/978-3-030-38144-8 [Google Scholar] [CrossRef]
19. Baskaran, K., Ali, M., Gingrich, K., Porter, D. L., Chong, S. et al. (2022). Sol-gel derived silica: A review of polymer-tailored properties for energy and environmental applications. Microporous and Mesoporous Materials, 336, 111874. https://doi.org/10.1016/j.micromeso.2022.111874 [Google Scholar] [CrossRef]
20. Hasanuzzaman, M., Rafferty, A., Sajjia, M., Olabi, A. G. (2016). Properties of glass materials. Encyclopedia of Materials: Technical Ceramics and Glasses, 647–657. https://doi.org/10.1016/B978-0-12-818542-1.03998-9 [Google Scholar] [CrossRef]
21. Owoeye, S. S., Jegede, F. I., Borisade, S. G. (2020). Preparation and characterization of nano-sized silica xerogel particles using sodium silicate solution extracted from waste container glasses. Materials Chemistry and Physics, 248, 122915. https://doi.org/10.1016/j.matchemphys.2020.122915 [Google Scholar] [CrossRef]
22. Ni’mah, Y. L., Suprapto, S., Subandi, A. P. K., Yuningsih, N. E., Pertiwi, A. C. (2022). The optimization of silica gel synthesis from chemical bottle waste using response surface methodology. Arabian Journal of Chemistry, 15(12), 104329. https://doi.org/10.1016/j.arabjc.2022.104329 [Google Scholar] [CrossRef]
23. Han, X., Wang, W., Ma, X. (2011). Adsorption characteristics of methylene blue onto low cost biomass material lotus leaf. Chemical Engineering Journal, 171(1), 1–8. https://doi.org/10.1016/j.cej.2011.02.067 [Google Scholar] [CrossRef]
24. Wang, P., Cao, M., Wang, C., Ao, Y., Hou, J. et al. (2014). Kinetics and thermodynamics of adsorption of methylene blue by a magnetic graphene-carbon nanotube composite. Applied Surface Science, 290, 116–124. https://doi.org/10.1016/j.apsusc.2013.11.010 [Google Scholar] [CrossRef]
25. Fu, J., Chen, Z., Wang, M., Liu, S., Zhang, J. et al. (2015). Adsorption of methylene blue by a high-efficiency adsorbent (polydopamine microspheresKinetics, isotherm, thermodynamics and mechanism analysis. Chemical Engineering Journal, 259, 53–61. https://doi.org/10.1016/j.cej.2014.07.101 [Google Scholar] [CrossRef]
26. Amode, J. O., Santos, J. H., Alam, Z. M., Mirza, A. H., Mei, C. C. (2016). Adsorption of methylene blue from aqueous solution using untreated and treated (Metroxylon spp.) waste adsorbent: Equilibrium and kinetics studies. International Journal of Industrial Chemistry, 7(3), 333–345. https://doi.org/10.1007/s40090-016-0085-9 [Google Scholar] [CrossRef]
27. Ronix, A., Pezoti, O., Souza, L. S., Souza, I. P. A. F., Bedin, K. C. et al. (2017). Hydrothermal carbonization of coffee husk: Optimization of experimental parameters and adsorption of methylene blue dye. Journal of Environmental Chemical Engineering, 5(5), 4841–4849. https://doi.org/10.1016/j.jece.2017.08.035 [Google Scholar] [CrossRef]
28. Mouni, L., Belkhiri, L., Bollinger, J. -C., Bouzaza, A., Assadi, A. et al. (2018). Removal of methylene blue from aqueous solutions by adsorption on Kaolin: Kinetic and equilibrium studies, Algeria. Applied Clay Science, 153, 38–45. https://doi.org/10.1016/j.clay.2017.11.034 [Google Scholar] [CrossRef]
29. Das, A. K., Dewanjee, S. (2018). Chapter 3-Optimization of extraction using mathematical models and computation. Computational Phytochemistry, 75–106. https://doi.org/10.1016/B978-0-12-812364-5.00003-1 [Google Scholar] [CrossRef]
30. Madondo, N. I., Chetty, M. (2022). Anaerobic co-digestion of sewage sludge and bio-based glycerol: Optimisation of process variables using one-factor-at-a-time (OFAT) and Box-Behnken design (BBD) techniques. South African Journal of Chemical Engineering, 40, 87–99. https://doi.org/10.1016/j.sajce.2022.02.003 [Google Scholar] [CrossRef]
31. Ke, G. J., Liu, T. S., Yang, P. F., Tang, X. L. (2019). Synthesis of mesoporous silica and its adsorption properties for chloride ions. Materials Science Forum, 956, 282–293. https://doi.org/10.4028/www.scientific.net/MSF.956.282 [Google Scholar] [CrossRef]
32. Hoang, C. V., Thoai, D. N., Cam, N. T. D., Phuong, T. T. T., Lieu, N. T. et al. (2022). Large-scale synthesis of nanosilica from silica sand for plant stimulant applications. ACS Omega, 7(45), 41687–41695. https://doi.org/10.1021/acsomega.2c05760 [Google Scholar] [PubMed] [CrossRef]
33. Guo, W., Wang, S., Wang, Y., Lu, S., Gao, Y. (2018). Sorptive removal of phenanthrene from aqueous solutions using magnetic and non-magnetic rice husk-derived biochars. Royal Society Open Science, 5(5), 172382. https://doi.org/10.1098/rsos.172382 [Google Scholar] [PubMed] [CrossRef]
34. Cloarec, J. P., Chevalier, C., Chevolot, Y., Baron, T., Souifi, A. (2016). pH driven addressing of silicon nanowires onto Si3N4/SiO2 micro-patterned surfaces. Nanotechnology, 27(29), 295602. https://doi.org/10.1088/0957-4484/27/29/295602 [Google Scholar] [PubMed] [CrossRef]
35. Çatlıoğlu, F. (2021). Preparation and application of Fe-modified banana peel in the adsorption of methylene blue: Process optimization using response surface methodology. Environmental Nanotechnology, Monitoring & Management, 16, 100517. https://doi.org/10.1016/j.enmm.2021.100517 [Google Scholar] [CrossRef]
36. Wang, J., Guo, X. (2020). Adsorption isotherm models: Classification, physical meaning, application and solving method. Chemosphere, 258, 127279. https://doi.org/10.1016/j.chemosphere.2020.127279 [Google Scholar] [PubMed] [CrossRef]
37. Abdulhameed, A. S., Hum, N. N. M. F., Rangabhashiyam, S., Jawad, A. H., Wilson, L. D. et al. (2021). Statistical modeling and mechanistic pathway for methylene blue dye removal by high surface area and mesoporous grass-based activated carbon using K2CO3 activator. Journal of Environmental Chemical Engineering, 9(4), 105530. https://doi.org/10.1016/j.jece.2021.105530 [Google Scholar] [CrossRef]
38. Bensalah, H., Younssi, S. A., Ouammou, M., Gurlo, A., Bekheet, M. F. (2020). Azo dye adsorption on an industrial waste-transformed hydroxyapatite adsorbent: Kinetics, isotherms, mechanism and regeneration studies. Journal of Environmental Chemical Engineering, 8(3), 103807. https://doi.org/10.1016/j.jece.2020.103807 [Google Scholar] [CrossRef]
39. Al Ghouti, M., Al Absi, R. S. (2020). Mechanistic understanding of the adsorption and thermodynamic aspects of cationic methylene blue dye onto cellulosic olive stones biomass from wastewater. Scientific Reports, 10(1), 15928. https://doi.org/10.1038/s41598-020-72996-3 [Google Scholar] [PubMed] [CrossRef]
Cite This Article
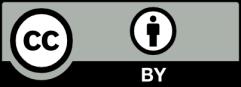
This work is licensed under a Creative Commons Attribution 4.0 International License , which permits unrestricted use, distribution, and reproduction in any medium, provided the original work is properly cited.