Open Access
ARTICLE
Experimental Investigation on Compressive Properties of Fiber Recycled Aggregate Concrete
College of Civil Engineering and Architecture, Guangxi University of Science and Technology, Liuzhou, 545006, China
* Corresponding Author: Yuliang Chen. Email:
(This article belongs to the Special Issue: Environmentally Friendly and Renewable Civil Engineer Materials)
Journal of Renewable Materials 2023, 11(11), 3957-3975. https://doi.org/10.32604/jrm.2023.028290
Received 10 December 2022; Accepted 20 February 2023; Issue published 31 October 2023
Abstract
This paper presents an experimental study to explore the compressive properties of fiber recycled aggregate concrete. A total of 75 specimens with the replacement rate of recycled coarse aggregate and fiber type were conducted under a uniaxial compressive test. The failure modes, stress-strain whole curves, peak stress, peak strain, and energy dissipation capacity were systematically observed and revealed. Test results indicate that steel fiber has the best modification effect on energy dissipation capacity and the toughness index of recycled concrete, corresponding to the enhancement of 81.75% and 22.90% on average. The addition of polyvinyl alcohol fiber can effectively improve the compressive strength and energy dissipation capacity of recycled aggregate concrete by 28.49% and 29.43% on average, respectively. The compressive strength and energy dissipation capacity of recycled aggregate concrete is increased by an average of 16.5% and 24.4% by incorporating carbon fiber. The energy dissipation capacity of recycled aggregate concrete is increased by an average of 13.5% with the incorporation of polypropylene fiber. However, the addition of carbon fiber results in a slight reduction of toughness by 16.97%, and the effect of polyvinyl alcohol fiber on the energy dissipation capacity is limited. Besides, with the increase in replacement rate, the compressive strength and the energy dissipation capacity of recycled coarse aggregate concrete with fiber decreased, and toughness first decreased and then increased. Finally, based on the analysis of test data, a segment-based stress-strain model of fiber recycled aggregate concrete was proposed, which shows good agreement with the test results.Graphic Abstract
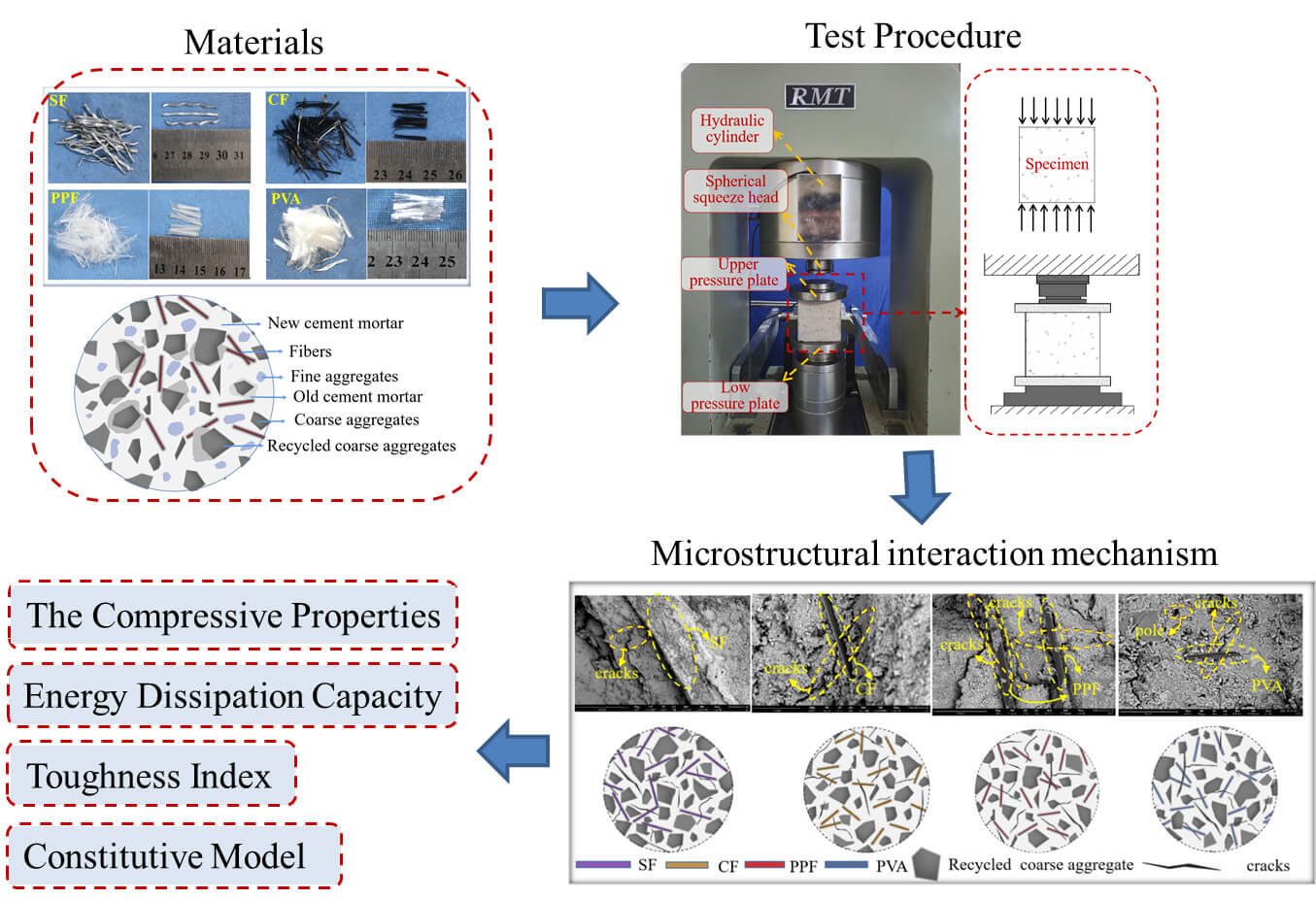
Keywords
Nomenclature
| Stress of fiber recycled aggregate concrete (MPa) |
| Strain of fiber recycled aggregate concrete |
| Peak stress of fiber recycled aggregate concrete (MPa) |
| Peak strain of fiber recycled aggregate concrete |
| The compressive strength of fiber recycled aggregate concrete (MPa) |
| The compressive strength of the ordinary recycled concrete (MPa) |
| Peak stress of the ordinary recycled concrete |
Concrete is one of the most used construction materials, and the growing demand is so pronounced. A large amount of stone, sand, cement and other materials are required to be used annually, which will soon exhaust non-renewable natural resources. With accelerated industrialization and urbanization, huge amounts of construction and demolition waste are generated [1]. The building waste is mainly transported to the landfills and disposed of, while the indecomposable building waste destroys the soil structure and causes serious environmental hazards.
The application and development of recycled aggregate concrete can solve the problem of disposal of construction solid waste and the depletion of natural mineral resources [2,3]. Compared to ordinary concrete, the mechanical performance of recycled aggregate concrete is slightly lower, and its usage is often limited to concrete pavement projects and non-structural concrete products [4–6]. This is due to some defects, such as old cement mortar and micro-cracks on the surface of recycled coarse aggregates, which caused a high number of weak interfaces between cement mortar and coarse aggregates inside the recycled aggregates concrete [7–9]. To improve the performance of recycled aggregate concrete, some methods have been proposed and studied [10–14], but the reuse of method in engineering applications is still limited as a result of the demerits of these techniques is time-consuming, cost-intensive, and inconvenient in practice. Therefore, the most practical approach is to use the crack-blocking and toughening effects of fibers to improve the utilization rate of recycled aggregate concrete and its development and application in practical engineering promotion [15–18].
In recent years, in order to further enhance the actual utilization value of recycled coarse aggregates, several researchers explored the properties of recycled aggregate concrete with incorporating different types of fibers [19–22]. In a study of the mechanical properties of steel fiber recycled aggregate concrete by Kaplan et al. [23], it was found that at recycled coarse aggregate content of 50%, the incorporated 1% steel fiber makes the improvement effect on the deformation performance of the concrete improved to the best effect. Shi et al. [24] investigated the effect of two types of steel fiber on the steel fiber reinforced concrete’s uniaxial compression and tension behavior. The results showed that adding fibers did not significantly change concrete compressive strength. Gao et al. [25] considered that a 1% volume replacement of steel fiber could improve the ductility and energy dissipation of the steel fiber reinforced aggregate concrete columns. An experimental investigation was conducted by Han et al. [26] to study the effect of adding carbon fibers to concrete. The author concluded that an obvious enhancement in the compressive strength. Chen et al. [27] indicated that the dynamic tensile strength and dynamic increase factor of concrete adding carbon fiber were lower than those of the control group without the carbon fiber. Guo et al. [28] assessed that carbon fibers increased the flexural and splitting strength of carbon fiber reinforced concrete, and the effect of carbon fiber length on the strength was quite limited for the concrete. Altalabani et al. [29] demonstrated that polypropylene fiber additive had the smallest effect on the compressive strength of self-compacting lightweight concrete. Qin et al. [30] revealed that due to the bridging effects of polypropylene fiber and the unit volume of polypropylene-fiber-fabric being less than that of polypropylene-fiber, the polypropylene fiber reinforced concrete under maximum load had lower breaking velocity than the concrete mixed with polypropylene fiber fabrics. Test results demonstrated that the effectiveness of polypropylene fiber concrete against freeze-thaw was discovered to increase slightly when compared to control concrete. Hesami et al. [31] proved that the addition of polypropylene fibers to Self-compacting concrete containing tire rubber crumb significantly increased the compressive, tensile, flexural, and abrasion strength. Zhang et al. [32] experimentally investigated the mechanical properties and the durability performances of normal and Polyvinyl Alcohol fiber (PVA) reinforced rubber concrete and found that ordinary concrete with the addition of rubber aggregate and PVA fiber reduced the compressive strength.
In summary, although existing studies have obtained much research production in improving the compressive mechanical behavior of recycled aggregate concrete by incorporating the single fiber and hybrid fiber since different fibers have their own characteristics, the mechanism of action varies greatly when fibers are stressed together with the cement matrix. Given the foundation of the existing study, this paper focuses on the investigation of four different fibers recycled aggregate concrete (FRAC) under compressive experiments to further explore the modification performance of fiber types on recycled concrete. The research revealed the modification performance and damage mechanism of different fiber types on compressive resistance of recycled aggregates concrete by scanning electron microscopy (SEM) and the influence of the above parameters on the mechanical properties of the compressive strength. And energy-consuming capacity of the recycled aggregates concrete was deeply analyzed. Besides, the piecewise constitutive model of the stress-strain relationship is proposed on different fiber recycled aggregate concrete to provide the basis and reference for the engineering application of fiber recycled concrete.
The recycled coarse aggregates (RCA) were made by crushing the abandoned concrete beams and columns of building demolition with a jaw crusher, and then recycled coarse aggregate used was 5–20 mm continuous grading, as shown in Fig. 1. Natural coarse aggregate (NCA) also used continuous grading macadam materials with 5–20 mm. The two types of coarse aggregates presented same particle size distributions, which were both within the bound limit of ASTMC33/C33M–13 [33], the physical properties of coarse aggregate are shown in Table 1. Furthermore, the adding a single fiber in the study, including corrugated steel fiber (SF), carbon fiber (CF), polypropylene fiber (PPF), and polyvinyl alcohol fiber (PVA) was selected and the physical properties were shown in Table 2, and the appearance was shown in Fig. 2. Normal river sands with fineness modulus of 2.38 were used as the fine aggregates, and an apparent density was 2640 kg/m3. The ordinary Portland cement (P.O.42.5) was used in the concrete mixtures. The mixing water was tap water in the city.
Figure 1: Particle size distribution of recycle coarse aggregate
Figure 2: Appearance of fiber
The mix proportion design was conducted according to the specification for mix proportion design of ordinary concrete, According to the concrete ratio design standard JGJ55-2011 [34]. The designed strength of recycled aggregate concrete used in trials was C35. Based on the replacement ratio of RCA with 0% (normal concrete), recycled aggregate concrete was prepared with the replacement ratio changed. That is, the NCA was replaced with the RCA in all coarse aggregates according to the equivalent replacement ratio, and the water-binder ratio, cement, fine aggregates, and coarse aggregates keep the total amount unchanged. Due to the water absorption rate of RCA being higher than that of NCA, and in order to ensure the workability of the obtained concrete, the additional water was added with the additional water by considering the amount of RCA. The proportions of five concrete mixtures were fabricated, as shown in Table 3.
2.3 Design and Production Specimen
To investigate the failure mechanism of uniaxial compression on recycled aggregate concrete with different fiber types, the replacement ratio of the RCA and the fiber type were used as the experimental variables in the study. The recycled coarse aggregate replacement rate was considered with 0%, 30%, 50%, 70%, and 100%, and the fiber type was considered four typical fibers, including steel fiber, carbon fiber, polypropylene fiber, polyvinyl alcohol fiber. The four fiber volume contents (Vf) had 1.0%, 0.3%, 0.1%, and 0.1%, respectively, according to the Chinese Code for Technical Specification for Application of Fiber Reinforced Concrete JGJ/T 221-2010 [35]. The different design parameters for each group specimen are shown in Table 4. For concrete mixtures with fiber, a total of 75 standard cube specimens with a dimension of 150 mm × 150 mm × 150 mm were designed and cast. The preparation and mixtures of test specimens for this research to obtain steel fiber recycled aggregate concrete, carbon fiber recycled aggregate concrete, polypropylene fiber recycled aggregate concrete, polyvinyl alcohol fiber recycled aggregate concrete was performed based on Standard test methods for fiber reinforced concrete. Firstly, the cement and aggregate were mixed in the forced mixer for two minutes, and then the single fiber was added by hand successively and mixed for two minutes. Finally, the water and the additional water were added to the concrete mixer and mixed for two minutes. After mixing completely, the concrete mixture was poured into the plastic molds and compacted on a vibration table. Finally, the specimens were demoulded after approximately 24 h and were placed in a curing room with a temperature of 20°C ± 2°C for 28 days.
The specimens were loading on the “Cube Compressive Strength Test” method specified in Chinese Code for Test Method of Mechanical Properties on Ordinary Concrete GB/T 50081-2019 [36]. The compressive strength test of the specimens were conducted by using the RMT-301 testing machine, as demonstrated in Fig. 3, and the test loading procedure adopted the displacement control method and the loading rate was 0.02 mm/s. The test stopped when the diagonal crack runs through specimens.
Figure 3: Compression test setup and detail drawing
The typical failure modes of different fiber recycled aggregate concrete samples under compressive loading are shown in Fig. 4. It can be observed that the fiber recycled aggregate concrete presented a quadrangular cone failure, which was characterized by positive and reverse quadrangular cone shape. This result was consistent with the previous investigation [37,38]. At the initial loading stage, the no-cracks were observed on the test blocks’ surface. This is because the concrete undertook minor loading, and the deformation of the specimen is mainly produced by aggregate and cement crystals. As the load increased, the micro-cracks began to appear at the end of the specimen surface, and the cracks developed in the direction parallel to the axial loading. When the load increased to the peak load, the lateral deformation of fiber recycled aggregate concrete increased significantly, and all of the volumes of the specimen expanded. Moreover, the diagonal cracks on the specimens’ surface also increased, widening and gradually running through.
Figure 4: Failure modes of different FRAC at the final damaged load
The micrographs interaction mechanism of the broken pieces of different fiber recycled aggregate concrete shown in Fig. 5 was obtained with a scanning electron microscope (SEM).
Figure 5: Microstructural interaction mechanism of FRAC
In the SEM test, first of all, the crushed specimens should be selected as specimens with regular shape, flat surface, thickness not more than 1 cm, and width of about 4–5 mm in small fragments, and the specimen fragment needed to contain the interface between aggregate and cement matrix, fiber and cement matrix connection. Then, the specimen will be placed in the drying oven to dry and take out the specimen. The conductive film will be attached to the flatter part of the fragment, and the specimen will be sprayed with gold. Finally, the gold-sprayed specimen is placed in the sample chamber, vacuumed, focussed, and imaged by high vacuum mode at 5 kV accelerating voltage, and captured [39].
As shown in Fig. 5a, although SF played a bridging role in the development of macroscopic cracks, there were obvious cracks between steel fiber and cement matrix, creating weak stress areas between the cement matrix. When SF was mixed in the specimen, the enhanced modes of steel fiber were not only reflected in the reduction of the expansion of primary cracks within the matrix at the early stage of crack development but also in improving the strength of the matrix around SF and enhancing the crack arresting effect of SF. Observed from Figs. 5b–5d, the incorporation of CF, PVA, and PPF fibers not only bore the traction force between the cracks but also gave full play to crack-arresting effectiveness. The surface hydration of PVA was better than that of CF and PPF, and the hydration product of the reaction between its surface and cement mortar increases the adhesion between cement mortar and PVA. However, due to a large number of harmful crystals adhering to the surface of polypropylene fiber and the existence of micro-cracks on the surface of some polypropylene fibers and cement matrix, which made the fibers easy to slip out of the cement matrix, and indicating that the adhesion between polypropylene fibers and cement matrix was small, as found in similar research by Qin et al. [30] and He et al. [40]. After peak load, the inclined cracks on the surface of fiber regenerated concrete rapidly increased and continued extending to the middle of the test specimens, and the inclined through cracks were gradually formed. Meanwhile, some concrete peeled off from the test specimen surface. The intersection angle between the failure section of specimens mixed with SF or CF fibers and the load vertical line was small, while the intersection angle between the failure section of specimens mixed with PVA, and PPF fibers and the load vertical line was larger. The reason was that polyvinyl alcohol fiber and polypropylene fiber have a small modulus of elasticity, which can inhibit early microscopic crack development, but the effect of fiber on macroscopic crack arrest was not obvious. After the peak load, the macroscopic cracks of the specimen expanded and linked up rapidly, forming the main crack, and the fibers were pulled off.
The impact of the fiber type is shown in Fig. 6, the recycled aggregate replacement rate on the stress (σ)-strain (ε) curves of the fiber recycled aggregate concrete. It can be seen that the stress-strain curves could be divided into four stages [41], that is, an elastic stage, the plastic deformation stage, the descending section, and a residual descending section. When the load increased from starting load to 60% of peak stress, the stress-strain curve of fiber recycled aggregate concrete increased linearly. In this stage, as shown in Figs. 6a–6c, When the replacement rate of recycled coarse aggregate was 0%, 30%, and 50%, the curve slope of specimens containing the SF, CF, and PPF fiber was slightly smaller than that of recycled aggregate concrete, and their initial stiffness was reduced. However, adding the PVA fiber into concrete increased on initial stiffness of specimens compared to recycled aggregate concrete. With the increased load, the specimens entered the plastic deformation stage, and the stress-strain curves gradually deviated from the linearity and showed a slightly convex trend. This was mainly because the micro-cracks in the fiber recycled aggregate concrete began to extend, and new micro-cracks were produced in weak areas such as pores. As demonstrated in Fig. 6c, the strain increased obviously when the replacement rate of recycled coarse aggregate is 50%. This was mainly because steel fibers are incorporated. The crack arresting effect of steel fibers will further increase the occlusion force between the recycled aggregate and the cement matrix, resulting in the increased deformation capacity of the specimens. As demonstrated in Fig. 6d, when the replacement rate of recycled coarse aggregate is 70%, the curve slope and their initial stiffness of specimens containing the SF, CF, and PVA fiber was slightly higher than that of recycled aggregate concrete. While adding the PPF fiber into concrete decreased on initial stiffness of specimens compared to recycled aggregate concrete. After the peak stress, the descending stage was entered. It can be seen from Fig. 6 that the slope of the stress-strain curves for specimen with steel fibers was gradually decreased, while the slope of the stress-strain curve for the specimens added the single CF, PPF, and PVA fibers rapidly declined. This may imply that the steel fibers played a role in the crack resistance fully, which limited the cracks’ development and the specimens’ lateral deformation. In the residual descending section, the dropping rate of the stress slowed down, and the residual stress tended to steady with increasing strain.
Figure 6: Stress-strain curves of fiber recycle aggregate concrete
The slope of the stress-strain curve and peak load of recycled aggregate concrete tended to decrease with the increase of the recycled coarse aggregate replacement ratio, as shown in Fig. 6. This is mainly due to the defective effect the recycled aggregate coarse was obvious such as old cement mortar adhering to the surface of recycled coarse aggregate and the existence of micro-cracks, which resulted in more weak interfaces between cement mortar and coarse aggregate. According to Adessina et al. [42] reported the attached old cement mortar determines the strength of concrete containing RCA. As can be seen from Figs. 6a, 6c, and 6d, the slope of the stress-strain curve and the peak load on the recycled concrete incorporated with CF and SF fibers decreased first and then increased with increasing recycled coarse aggregate replacement rate. The slope of the stress-strain curve and the peak load of the recycled aggregate concrete mixed with PPF fibers decreased first and then increased compared to the ordinary concrete with PPF fibers, as shown in Figs. 6a and 6c. As demonstrated in Fig. 6, the slope of the stress-strain curve for the recycled aggregate concrete with PVA tended to increase with the rise in the replacement rate of recycled coarse aggregate. It is mainly attributed to the fact that the pores inside the recycled coarse aggregate would cause stress concentration phenomenon, which caused premature damage cracks in the test block.
The peak stress σc and peak strain εc were shown in Table 5, and the experimental results were the average values of the test data of the same group for specimens.
3.3.1 Effect of Fiber Type on the Compressive Strength
The various relation between the compressive strength of the fiber recycle aggregate concrete and fiber type is shown in Fig. 7. It can be observed that the incorporation of SF, CF, and PVA fibers result in an increase of compressive strength in comparison with recycled aggregate concrete, while the compressive strength of recycled aggregate concrete was reduced with PPF fiber. Li et al. [39] and Karahan et al. [43] also studied that the addition of polypropylene fibers into concrete could lower its compressive strength. The compressive strength of recycled aggregate concrete with SF, CF, and PVA fibers was increased by 8.86%, 16.49%, and 28.09% on average, respectively, compared to the recycled aggregate concrete, where there exists the lowest increment on the recycled aggregate concrete with SF fiber. This was mainly attributed to the reason that the boundary effect of SF fiber was barely played at an elastic stage and the plastic deformation stage. In addition, the compressive strength of recycled aggregate concrete with CF and PVA was increased obviously. One reason is that CF, and PVA fibers dispersed in recycled aggregate concrete uniformly, which made the internal structure of the specimens compact. Another reason is that CF and PVA fiber could inhibit the generation and development of micro-cracks, which causes the enhanced effect of the fibers more than the negative influence of the recycled coarse aggregate. However, the compressive strength of all specimens containing PPF fiber declined from 25.74% to 4.68% when the recycled coarse aggregate replacement rate was increased from 50% to 70%. The following explanation could be given: (1) there are micro-cracks within the recycled coarse aggregate and defects such as old mortar on the surface [44,45]; (2) the PPF fiber of the specimens can hinder the air bubbles generated during the concrete mixing process [46].
Figure 7: Effect of fiber type on peak stress
3.3.2 Effect of the Replacement Rate on the Compressive Strength
The effect of the compressive strength of fiber recycled aggregate concrete with various the replacement rate of recycled coarse aggregate is shown in Fig. 8. The ratio of compressive strength of recycled concrete with different fiber types to that of ordinary recycled concrete with the same replacement rate was fc/fco. As observed from Fig. 8, compared to the carbon fiber concrete, the peak stress of specimens incorporated with CF increased by 0.79%–21.34% when the recycled coarse aggregate replacement rate increased from 0% to 100%. It was indicated that the enhanced effect of the fibers more than the negative influence of the recycled coarse aggregate, which caused the increase of the compressive strength. However, when the recycled aggregate concrete with the addition of PVA fibers decreased by 4.18%–20.97%, implying that there was an obvious weakening effect of the replacement rate on adding PVA of recycled aggregate concrete. Chen et al. [47] also proved that the shear strength decreases of polyvinyl alcohol fiber with an increasing replacement rate of recycled coarse aggregate. The recycled aggregate concrete with PPF fiber was decreased by 4.68%–25.74% in comparison with the polypropylene fiber concrete. It is implied that the reinforcing effect of polypropylene fibers on recycled concrete gradually decreases as the replacement rate increases. On the one hand, because of the poor hydrophilicity of PPF, the degree of hydration reaction between the fiber surface and the cement matrix is weak, and fewer hydration products are gathered on the fiber surface, making the adhesion between PPF and the cement matrix poor. On the other hand, the reinforcing effect of fiber crack arrest is weaker than the negative effect of recycled coarse aggregate.
Figure 8: Effect of replacement rate on normalized compressive strength
3.3.3 Effect of the Fiber Type on Peak Strain
The comparative relationship between the peak strains of fiber recycled aggregate concrete and different fiber types is shown in Fig. 9. It can be found that the incorporation of SF, CF and PPF fibers can improve the peak strain of recycled aggregate concrete [48,49]. This can be ascribed to the fibers’ bridging effect during the specimen’s compression. While the peak strains of specimens with PVA fiber were reduced by 16.62% compared with that of recycled aggregate concrete, indicating that the incorporation of PVA fiber made recycled aggregate concrete closely, and further, the stiffness and bearing capacity of recycled aggregate concrete was increased. When the replacement rate of recycled coarse aggregate was 50%, the corresponding peak strain of all specimens with SF fiber was 19.52 × 10−3, which can be 50.97% higher than that of the recycled aggregate concrete. And these results were consistent with Afroughsabet et al.’s studies [50]. This is mainly due to the old mortar attached to the surface of recycled coarse aggregate causing the porosity of fiber recycled aggregate concrete, and the gel within specimens was increased after the cement played the hydration reaction, which made the deformation specimens expand.
Figure 9: Effect of the fiber type on peak strain
3.3.4 Effect of the Replacement Rate on Peak Strain
The effect of different replacement rates on peak strain for fiber recycled aggregate concrete is shown in Fig. 10. The ratio of peak strain for fiber recycled aggregate concrete to that of recycled aggregate concrete at the same replacement rate was ε/εco. With the increase of the replacement rate, the peak strain of the adding SF recycled aggregate concrete showed a declining trend compared to the steel fiber concrete. This finding conformed with the previous study [25]. While there was no obvious effect on the peak strain of recycled aggregate concrete with CF, implying that carbon fiber could enhance the bridge effect and effectively make up for the deficiency of recycled coarse aggregate. The peak strain of all specimens with PVA fiber declined by 7.66%–28.68% when the replacement rate of recycled coarse aggregate was increased from 0% to 100%.
Figure 10: Effect of fiber type on normalized peak strain
According to the stress-strain curves of the fiber recycled aggregate concrete, the area beneath the stress-strain curves from the beginning of loading to the peak load is considered as damaged energy absorption (CBE). The area beneath the stress-strain curves from the beginning of loading to 85% of the falling section’s peak load is considered total energy absorption (CTE) [51,52]. The toughness index (TR) is used to evaluate the deformability of the columns, which is defined as the ratio between CTE and CBE. The division of energy consumption area is shown in Fig. 11.
Figure 11: Division of energy consumption area
Based on the defined parameters, the damaged energy absorption, the total energy absorption, and the toughness index of each group of specimens were obtained by calculation, as shown in Table 6.
4.1 Effect of Fiber Type on Energy Dissipation Capacity
The relation of the energy dissipation at different fiber types for the fiber recycled aggregate concrete is shown in Fig. 12, where the damaged energy absorption and the total energy absorption of the specimens with SF, CF, PPF, and PVA fibers were obtained by calculating the average value with the replacement rate of recycled coarse aggregate. As observed in Fig. 12, the energy dissipation and the toughness index of recycled aggregate concrete with fibers show a similar increasing trend. This was also previously observed by other researchers [53,54]. The average damaged energy absorption with SF, CF, PPF, and PVA was increased by 57.57%, 42.23%, 10.42%, and 13.76%, higher than the recycled aggregate concrete. The average total energy absorption with SF, CF, PPF, and PVA was raised by 81.75%, 24.41%, 13.51%, and 29.44%, higher than the recycled aggregate concrete. It was demonstrated that adding the fibers to concrete mixture could improve energy dissipation when compared with recycled aggregate concrete, where the incorporation SF was obvious in enhancing the energy consumption of the specimens. This is because the water-cement ratio of recycled concrete was reduced after the water of the cement mixture was absorbed by the recycled coarse aggregate so that the adhesion between the steel fibers and the matrix was closer, and the energy consumption of the specimen was better.
Figure 12: Relationship between energy consumption and fiber type of FRAC
As can be seen from Fig. 12, the toughness index of the specimen with SF, PPF, and PVA fiber was 2.56, 2.32, and 2.22, respectively, corresponding to enhanced by 22.90%, 11.33%, 6.18% compared to the recycled aggregate concrete. In the previous studies by Wang et al. [55] and Song et al. [56], the incorporation of fiber positively affected the compression toughness of recycled concrete. This indicates that the SF and PPF fiber had a stronger crack-arresting and toughening effect on the specimens.
4.2 Effect of the Replacement Rate on Energy Dissipation Capacity
The relationship between the energy dissipation and the replacement rate of recycled aggregate concrete is shown in Fig. 13, where the damaged energy absorption and the total energy absorption of the specimens with 0%, 30%, 50%, 70% and 100% were obtained by calculating the average value with the SF, CF, PPF, and PVA fibers at each the replacement rate.
Figure 13: Relationship between energy consumption and replacement rate of FRAC
Overall, the energy dissipation capacity of the recycle aggregate concrete showed a decreasing tendency, and the toughness index first increased and then decreased. Compared with the ordinary concrete, the damaged energy absorption and the total energy absorption were 330.14 and 534.12 J, corresponding to a reduction of 1.39%, and 7.94% when the recycled aggregate concrete replacement rate was 30%. This was attributed to the concrete matrix bearing the load mainly in the elastic stage, while the inner recycled coarse aggregate had a micro-crack, and the number of cracks increased with the rise of the replacement rate, which caused the energy dissipation capacity of the recycled aggregate concrete to be reduced. When the replacement rate of recycled aggregate concrete was 50%, 70%, and 100%, the energy dissipation capacity showed a slightly decreasing tendency, and the toughness index of recycled aggregate concrete declined by 1.71%, 6.65%, and 0.74% compared with the ordinary concrete. It is shown that the increase of pores and micro-cracks in the recycled coarse aggregate made the specimens decline.
5 The Stress-Strain Curve Model for Fiber Recycled Concrete
According to the characteristics of fiber recycled aggregate concrete, the relevant parameters of the existing model suggested by Guo [57] were modified, which established the stress-strain curve model of fiber recycled concrete. The model expressions suggested by Guo [57] were followed by Eq. (1).
where x = ε/εc, y = δ/δc. ε and δ are every stress and strain, respectively, corresponding to the stress-strain curves of the fiber recycled aggregate concrete. εc is the peak strain, and δc is the peak stress. The initial slope of the stress-strain curve is indicated by the a. b denotes the region that the descending section surrounds the stress-strain curve. The equation parameters a and b values of the fiber recycled aggregate concrete under different types of fiber and the replacement ratio of RCA could be obtained by fitting the test data. To accurately calculate the range of adaptation of the formula, the average values of a and b fitted to each group of different fiber recycled concrete were selected as the suggested values.
Based on the analysis of the experimental results, the calculated value a and b was on average values under the different replacement ratio of FRAC. a and b were 0.97 and 2.18 for recycled aggregates concrete. a and b were 1.25 and 1.03 respectively for steel fiber recycled aggregates concrete. a and b were 0.73 and 3.17 respectively for carbon fiber recycled aggregates concrete. a and b were 0.88 and 3.29 respectively for polypropylene fiber recycled aggregates concrete. a and b were 0.49 and 1.76 respectively for polyvinyl alcohol fiber recycled aggregates concrete.
As presented in Fig. 14, it can be found that there is a certain amount of increase in values and a decrease in b values after adding the SF fiber. The decrease of values and the increase of b values were shown for the recycled aggregate concrete with CF and PPF fibers compared with the recycled aggregate concrete. The effect of PVA fibers on decreasing a and b values was pronounced compared with the recycled aggregate concrete. Besides, a and b values of the fiber recycled aggregate concrete decreased as the replacement ratio of recycled aggregate concrete increased from 0% to 100%. The calculated average values a and b were added to (1), which obtained the stress-strain curve model of fiber recycled concrete. It can be found from the fitting curve that the test result fitted well with expression result at the ascending section, while there were error curves at descending section.
Figure 14: Comparison of test and calculated stress-strain curves of the FRAC
This paper studied the mechanical behavior of fiber recycled aggregate concrete under unaxial compression. The experimental behaviors were the influence of recycled coarse aggregate replacement rate (0%, 30%, 50%, 70% and 100%) and fiber type (corrugated steel fiber (SF), carbon fiber (CF), polypropylene fiber (PPF), and polyvinyl alcohol fiber (PVA) ) on the compressive performance of recycled aggregate concrete. From the experimental investigation, the main conclusions drawn from this paper are as follows:
(1) The failure modes of fiber recycled aggregate concrete are similar to ordinary concrete under uniaxial compression, which is positive and reverse quadrangular cone shape. Among them, during destruction, there is a larger transverse expansion on recycled aggregate concrete with steel fibers.
(2) The addition of polyvinyl alcohol fiber can effectively enhance the compressive strength of recycled aggregate concrete by 28.49% on average. Besides, steel fiber has the best energy-absorption capability and toughness index, corresponding to the ameliorative effect of 81.75% and 22.90%, while the increase in compressive strength has no obvious effect.
(3) The compressive strength of fiber recycled aggregate concrete decreases with an increasing replacement ratio. Among these, a maximum strength loss of the fiber recycled aggregate concrete occurs when the replacement ratio is increased from 50% to 70%. With the increase of the replacement ratio, the energy dissipation capacity and toughness of the fiber recycle aggregate concrete declined by 12.4% and 4.18% on average.
(4) Based on the test result under uniaxial compression, the piecewise constitutive model of the stress-strain relationship is proposed, which considers the effect of fiber type and recycled aggregate replacement rate. The curve obtained by the equation agrees well with the experimental curve.
Funding Statement: This work is supported by the Postdoctoral Science Foundation of China (2021M693854), the Doctoral Foundation of Guangxi University of Science and Technology (No. 18Z09), and Bagui Scholar Program sponsored from the People’s Government of Guangxi Zhuang Autonomous Region (No. 2019(79)). The financial support is gratefully acknowledged.
Conflicts of Interest: The authors declare that they have no conflicts of interest to report regarding the present study.
References
1. Park, W. J., Kim, R., Roh, S., Ban, H. (2020). Identifying the major construction wastes in the building construction phase based on life cycle assessments. Sustainability, 12(19), 8096. https://doi.org/10.3390/su12198096 [Google Scholar] [CrossRef]
2. Wijayasundara, M., Mendis, P., Crawford, R. H. (2018). Integrated assessment of the use of recycled concrete aggregate replacing natural aggregate in structural concrete. Journal of Cleaner Production, 174, 591–604. https://doi.org/10.1016/j.jclepro.2017.10.301. [Google Scholar] [CrossRef]
3. Kurda, R., de Brito, J., Silvestre, J. D. (2017). Combined influence of recycled concrete aggregates and high contents of fly ash on concrete properties. Construction and Building Materials, 157, 554–572. https://doi.org/10.1016/j.conbuildmat.2017.09.128 [Google Scholar] [CrossRef]
4. Xiao, J., Tang, Y., Chen, H., Zhang, H., Xia, B. (2022). Effects of recycled aggregate combinations and recycled powder contents on fracture behavior of fully recycled aggregate concrete. Journal of Cleaner Production, 366(9), 132895. https://doi.org/10.1016/j.jclepro.2022.132895 [Google Scholar] [CrossRef]
5. Thomas, J., Thaickavil, N. N., Wilson, P. M. (2018). Strength and durability of concrete containing recycled concrete aggregates. Journal of Building Engineering, 19, 349–365. https://doi.org/10.1016/j.jobe.2018.05.007 [Google Scholar] [CrossRef]
6. Pacheco, J., de Brito, J., Chastre, C., Evangelista, L. (2019). Experimental investigation on the variability of the main mechanical properties of concrete produced with coarse recycled concrete aggregates. Construction and Building Materials, 201(2), 110–120. https://doi.org/10.1016/j.conbuildmat.2018.12.200 [Google Scholar] [CrossRef]
7. Kim, Y., Hanif, A., Kazmi, S. M., Munir, M. J., Park, C. (2018). Properties enhancement of recycled aggregate concrete through pretreatment of coarse aggregates–comparative assessment of assorted techniques. Journal of Cleaner Production, 191, 339–349. https://doi.org/10.1016/j.jclepro.2018.04.192 [Google Scholar] [CrossRef]
8. Geng, Y., Wang, Q., Wang, Y., Zhang, H. (2019). Influence of service time of recycled coarse aggregate on the mechanical properties of recycled aggregate concrete. Materials and Structures, 52(5), 1–16. https://doi.org/10.1617/s11527-019-1395-0 [Google Scholar] [CrossRef]
9. McGinnis, M. J., Davis, M., de la Rosa, A., Weldon, B. D., Kurama, Y. C. (2017). Strength and stiffness of concrete with recycled concrete aggregates. Construction and Building Materials, 154, 258–269. https://doi.org/10.1016/j.conbuildmat.2017.07.015 [Google Scholar] [CrossRef]
10. Wu, K., Luo, S., Zheng, J., Yan, J., Xiao, J. (2022). Influence of carbonation treatment on the properties of multiple interface transition zones and recycled aggregate concrete. Cement and Concrete Composites, 127, 104402. https://doi.org/10.1016/j.cemconcomp.2021.104402 [Google Scholar] [CrossRef]
11. Truong, G. T., Son, M. K., Choi, K. K. (2019). Mechanical performance and durability of latex-modified fiber-reinforced concrete. Journal of Advanced Concrete Technology, 17(2), 79–92. https://doi.org/10.3151/jact.17.79 [Google Scholar] [CrossRef]
12. Alqarni, A. S., Abbas, H., Al-Shwikh, K. M., Al-Salloum, Y. A. (2021). Treatment of recycled concrete aggregate to enhance concrete performance. Construction and Building Materials, 307, 124960. https://doi.org/10.1016/j.conbuildmat.2021.124960 [Google Scholar] [CrossRef]
13. Lu, B., Shi, C., Cao, Z., Guo, M., Zheng, J. (2019). Effect of carbonated coarse recycled concrete aggregate on the properties and microstructure of recycled concrete. Journal of Cleaner Production, 233, 421–428. https://doi.org/10.1016/j.jclepro.2019.05.350 [Google Scholar] [CrossRef]
14. El-Hawary, M., Al-Sulily, A. (2020). Internal curing of recycled aggregates concrete. Journal of Cleaner Production, 275, 122911. https://doi.org/10.1016/j.jclepro.2020.122911 [Google Scholar] [CrossRef]
15. Dadmand, B., Pourbaba, M., Sadaghian, H. (2020). Effectiveness of steel fibers in ultra-high-performance fiber-reinforced concrete construction. Advances in Concrete Construction, 10(3), 195–209. [Google Scholar]
16. Fan, Z., Liu, H., Liu, G., Wang, X., Cui, W. (2022). Compressive performance of fiber reinforced recycled aggregate concrete by basalt fiber reinforced polymer-polyvinyl chloride composite Jackets. Journal of Renewable Materials, 4(11), 1763–1791. [Google Scholar]
17. Zhang, X., Gao, X., Wang, X., Meng, E., Wang, F. (2020). Axial compression performance of basalt-fiber-reinforced recycled-concrete-filled square steel tubular stub column. Advances in Concrete Construction, 10(6), 559–571. [Google Scholar]
18. Chen, Y., Li, P., Ye, P., Li, H., Liang, X. (2022). Experimental investigation on the mechanical behavior of polyvinyl alcohol fiber recycled aggregate concrete under triaxial compression. Construction and Building Materials, 350, 128825. https://doi.org/10.1016/j.conbuildmat.2022.128825 [Google Scholar] [CrossRef]
19. Hogancamp, J., Grasley, Z. (2017). The use of microfine cement to enhance the efficacy of carbon nanofibers with respect to drying shrinkage crack resistance of portland cement mortars. Cement and Concrete Composites, 83, 405–414. https://doi.org/10.1016/j.cemconcomp.2017.08.006 [Google Scholar] [CrossRef]
20. Zong, S., Liu, Z., Li, S., Lu, Y., Zheng, A. (2021). Stress-strain behaviour of steel-fibre-reinforced recycled aggregate concrete under axial tension. Journal of Cleaner Production, 278, 123248. https://doi.org/10.1016/j.jclepro.2020.123248 [Google Scholar] [CrossRef]
21. Mudadu, A., Tiberti, G., Plizzari, G. A., Morbi, A. (2019). Post-cracking behavior of polypropylene fiber reinforced concrete under bending and uniaxial tensile tests. Structural Concrete, 20(4), 1411–1424. https://doi.org/10.1002/suco.201800224 [Google Scholar] [CrossRef]
22. Du, X., Li, Y., Si, Z., Huang, L., Chen, X. (2022). Effects of basalt fiber and polyvinyl alcohol fiber on the properties of recycled aggregate concrete and optimization of fiber contents. Construction and Building Materials, 340(1), 127646. https://doi.org/10.1016/j.conbuildmat.2022.127646 [Google Scholar] [CrossRef]
23. Kaplan, G., Bayraktar, O. Y., Gholampour, A., Gencel, O., Koksal, F. et al. (2021). Mechanical and durability properties of steel fiber-reinforced concrete containing coarse recycled concrete aggregate. Structural Concrete, 22(5), 2791–2812. https://doi.org/10.1002/suco.202100028 [Google Scholar] [CrossRef]
24. Shi, X. J., Park, P., Rew, Y., Huang, K. J., Sim, C. (2020). Constitutive behaviors of steel fiber reinforced concrete under uniaxial compression and tension. Construction and Building Materials, 233, 117316. https://doi.org/10.1016/j.conbuildmat.2019.117316 [Google Scholar] [CrossRef]
25. Gao, D., Li, W., Pang, Y., Huang, Y. (2021). Behavior analysis and strength prediction of steel fiber reinforced recycled aggregate concrete column under axial compression. Construction and Building Materials, 290, 123278. https://doi.org/10.1016/j.conbuildmat.2021.123278 [Google Scholar] [CrossRef]
26. Han, B., Zhang, L., Zhang, C., Wang, Y., Yu, X. et al. (2016). Reinforcement effect and mechanism of carbon fibers to mechanical and electrically conductive properties of cement-based materials. Construction and Building Materials, 125, 479–489. https://doi.org/10.1016/j.conbuildmat.2016.08.063 [Google Scholar] [CrossRef]
27. Chen, Z. Y., Yang, J. (2020). Experimental study on dynamic splitting characteristics of carbon fiber reinforced concrete. Materials, 14(1), 94. https://doi.org/10.3390/ma14010094 [Google Scholar] [PubMed] [CrossRef]
28. Guo, Z., Zhuang, C. L., Li, Z. H., Chen, Y. (2021). Mechanical properties of carbon fiber reinforced concrete (CFRC) after exposure to high temperatures. Composite Structures, 256, 113072. https://doi.org/10.1016/j.compstruct.2020.113072 [Google Scholar] [CrossRef]
29. Altalabani, D., Bzeni, D. K., Linsel, S. (2020). Mechanical properties and load deflection relationship of polypropylene fiber reinforced self-compacting lightweight concrete. Construction and Building Materials, 252, 119084. https://doi.org/10.1016/j.conbuildmat.2020.119084 [Google Scholar] [CrossRef]
30. Qin, Y., Zhang, X., Chai, J., Xu, Z., Li, S. (2019). Experimental study of compressive behavior of polypropylene-fiber-reinforced and polypropylene-fiber-fabric-reinforced concrete. Construction and Building Materials, 194, 216–225. https://doi.org/10.1016/j.conbuildmat.2018.11.042 [Google Scholar] [CrossRef]
31. Hesami, S., Hikouei, I. S., Emadi, S. A. A. (2016). Mechanical behavior of self-compacting concrete pavements incorporating recycled tire rubber crumb and reinforced with polypropylene fiber. Journal of Cleaner Production, 133, 228–234. https://doi.org/10.1016/j.jclepro.2016.04.079 [Google Scholar] [CrossRef]
32. Zhang, H., Wang, L., Zheng, K., Bakura, T. J., Totakhil, P. G. (2018). Research on compressive impact dynamic behavior and constitutive model of polypropylene fiber reinforced concrete. Construction and Building Materials, 187, 584–595. https://doi.org/10.1016/j.conbuildmat.2018.07.164 [Google Scholar] [CrossRef]
33. ASTM C33/C33M-13 (2013). Standard specification for concrete aggregates. West Conshohocken: PA ASTM International. [Google Scholar]
34. JGJ 55-2011 (2011). Specification for mix proportion design of ordinary concrete. Beijing, China: China Architecture & Building Press. [Google Scholar]
35. JGJ/T 221-2010 (2010). Technical specification for application of fiber reinforced concrete. Beijing, China: China Architecture & Building Press. [Google Scholar]
36. GB/T 50081-2011 (2019). Standard for test method of mechanical properties on ordinary concrete. Beijing, China: China Standard Press. [Google Scholar]
37. Zhang, X., Kuang, X., Wang, F., Wang, S. (2019). Strength indices and conversion relations for basalt fiber-reinforced recycled aggregate concrete. Revista DYNA, 94(1), 82–87. https://doi.org/10.6036/DYNAII [Google Scholar] [CrossRef]
38. Zhang, C., Wang, Y., Zhang, X., Ding, Y., Xu, P. (2021). Mechanical properties and microstructure of basalt fiber-reinforced recycled concrete. Journal of Cleaner Production, 278, 123252. https://doi.org/10.1016/j.jclepro.2020.123252 [Google Scholar] [CrossRef]
39. Li, Y., Zhou, Y., Wang, R., Li, Y., Wu, X. et al. (2022). Experimental investigation on the properties of the interface between RCC layers subjected to early-age frost damage. Cement and Concrete Composites, 134, 104745. https://doi.org/10.1016/j.cemconcomp.2022.104745 [Google Scholar] [CrossRef]
40. He, W., Kong, X., Fu, Y., Zhou, C., Zheng, Z. (2020). Experimental investigation on the mechanical properties and microstructure of hybrid fiber reinforced recycled aggregate concrete. Construction and Building Materials, 261, 120488. https://doi.org/10.1016/j.conbuildmat.2020.120488 [Google Scholar] [CrossRef]
41. Wu, Z., Zhang, J., Yu, H., Ma, H., Wang, H. et al. (2022). Experiment and mesoscopic modelling on the dynamic compressive behaviors of a new carbon fiber-reinforced cement-based composite. Cement and Concrete Composites, 130, 104519. https://doi.org/10.1016/j.cemconcomp.2022.104519 [Google Scholar] [CrossRef]
42. Adessina, A., Fraj, A. B., Barthélémy, J. F., Chateau, C., Garnier, D. (2019). Experimental and micromechanical investigation on the mechanical and durability properties of recycled aggregates concrete. Cement and Concrete Research, 126, 105900. https://doi.org/10.1016/j.cemconres.2019.105900. [Google Scholar] [CrossRef]
43. Karahan, O., Atiş, C. D. (2011). The durability properties of polypropylene fiber reinforced fly ash concrete. Materials & Design, 32(2), 1044–1049. https://doi.org/10.1016/j.matdes.2010.07.011 [Google Scholar] [CrossRef]
44. Verian, K. P., Ashraf, W., Cao, Y. (2018). Properties of recycled concrete aggregate and their influence in new concrete production. Resources, Conservation and Recycling, 133, 30–49. https://doi.org/10.1016/j.resconrec.2018.02.005 [Google Scholar] [CrossRef]
45. Wang, R., Yu, N., Li, Y. (2020). Methods for improving the microstructure of recycled concrete aggregate: A review. Construction and Building Materials, 242, 118164. https://doi.org/10.1016/j.conbuildmat.2020.118164 [Google Scholar] [CrossRef]
46. Matar, P., Zéhil, G. P. (2019). Effects of polypropylene fibers on the physical and mechanical properties of recycled aggregate concrete. Journal of Wuhan University of Technology-Mater. Sci. Ed., 34(6), 1327–1344. https://doi.org/10.1007/s11595-019-2196-6 [Google Scholar] [CrossRef]
47. Chen, Y., Zhang, S., Ye, P., Liang, X. (2022). Mechanical properties and damage constitutive of recycled aggregate concrete with polyvinyl alcohol fiber under compression and shear. Case Studies in Construction Materials, 17, e01466. [Google Scholar]
48. Niu, H., Wang, L., Li, J., Ji, J. (2021). Experimental study on mechanical properties of steel-polyvinyl Alcohol fibre-reinforced recycled concrete. Applied Sciences, 11(22), 10550. https://doi.org/10.3390/app112210550 [Google Scholar] [CrossRef]
49. Aflaki Samani, M., Jabbari Lak, S. (2019). Experimental investigation on the mechanical properties of recycled aggregate concrete reinforced by waste carbon fibers. International Journal of Environmental Science and Technology, 16(8), 4519–4530. https://doi.org/10.1007/s13762-018-1855-z [Google Scholar] [CrossRef]
50. Afroughsabet, V., Biolzi, L., Ozbakkaloglu, T. (2017). Influence of double hooked-end steel fibers and slag on mechanical and durability properties of high performance recycled aggregate concrete. Composite Structures, 181, 273–284. https://doi.org/10.1016/j.compstruct.2017.08.086 [Google Scholar] [CrossRef]
51. Khan, M., Ali, M. (2018). Effectiveness of hair and wave polypropylene fibers for concrete roads. Construction and Building Materials, 166, 581–591. https://doi.org/10.1016/j.conbuildmat.2018.01.167 [Google Scholar] [CrossRef]
52. Zia, A., Ali, M. (2017). Behavior of fiber reinforced concrete for controlling the rate of cracking in canal-lining. Construction and Building Materials, 155(3), 726–739. https://doi.org/10.1016/j.conbuildmat.2017.08.078 [Google Scholar] [CrossRef]
53. Ye, P., Chen, Z., Su, W. (2022). Mechanical properties of fully recycled coarse aggregate concrete with polypropylene fiber. Case Studies in Construction Materials, 17, e01352. https://doi.org/10.1016/j.cscm.2022.e01352 [Google Scholar] [CrossRef]
54. Noushini, A., Samali, B., Vessalas, K. (2013). Effect of polyvinyl alcohol (PVA) fibre on dynamic and material properties of fibre reinforced concrete. Construction and Building Materials, 49, 374–383. https://doi.org/10.1016/j.conbuildmat.2013.08.035 [Google Scholar] [CrossRef]
55. Wang, J., Dai, Q., Si, R., Guo, S. (2018). Investigation of properties and performances of Polyvinyl Alcohol (PVA) fiber-reinforced rubber concrete. Construction and Building Materials, 193, 631–642. https://doi.org/10.1016/j.conbuildmat.2018.11.002 [Google Scholar] [CrossRef]
56. Song, W., Yin, J. (2016). Hybrid effect evaluation of steel fiber and carbon fiber on the performance of the fiber reinforced concrete. Materials, 9(8), 704. https://doi.org/10.3390/ma9080704 [Google Scholar] [PubMed] [CrossRef]
57. Guo, Z. H. (2013). Principles of reinforced concrete. Beijing, China: Tsinghua University Press. [Google Scholar]
Cite This Article
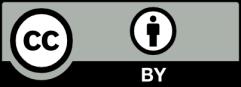
This work is licensed under a Creative Commons Attribution 4.0 International License , which permits unrestricted use, distribution, and reproduction in any medium, provided the original work is properly cited.