Open Access
ARTICLE
Nail Holding Performance of Self-Tapping Screws on Masson Pine and Chinese Fir Dimension Lumbers
1
College of Forestry, Guizhou University, Guiyang, 550025, China
2
Forestry Science and Technology Promotion Station, Xifeng County Bureau of Natural Resources, Guiyang, 551100, China
3
Forest Park Management Section, Zhazuo State-Owned Forest Farm, Guiyang, 550299, China
4
College of Civil Engineering, Kaili University, Qiandongnan, 556011, China
* Corresponding Authors: Zhigang Wu. Email: ; Jiankun Liang. Email:
# De Li and Bengang Zhang have contributed equally to this work
Journal of Renewable Materials 2023, 11(10), 3725-3738. https://doi.org/10.32604/jrm.2023.027895
Received 20 November 2022; Accepted 04 January 2023; Issue published 10 August 2023
Abstract
Screw connection is a type most commonly applied to timber structures. As important commercial tree species in China, Masson pine and Chinese fir have the potential to prepare wood structures. In this study, the effects of the diameter of the self-tapping screw and the guiding bores on the nail holding performance on different sections of Masson pine and Chinese fir dimension lumbers were mainly explored. The results showed that: (1) The nail holding strength of the tangential section was the maximum, followed by that of the radial section, and that of the cross section was the minimum. (2) The nail holding strength of Masson pine was higher than that of Chinese fir. (3) The nail holding strength grew with the increase in the diameter of self-tapping screws, but a large diameter would lead to plastic cracking of the timber, thus further affecting the nail holding strength. Masson pine and Chinese fir reached the maximum nail holding strength when the diameter of self-tapping screws was 3.5 mm. (4) Under a large diameter of screws, prefabricated guiding bores could mitigate timber cracking and improve its nail holding strength. (5) Prefabricated guiding bores were more necessary for the screw connection of Masson pine. The results obtained could provide a scientific basis for the screw connection design of Masson pine and Chinese fir timber structures.Keywords
Timber architecture is environment-friendly, with strong seismic performance and high comfortability [1–5]. Screw connection, an important connection type most commonly applied to timber structures, is characterized by sufficient tightness and toughness and convenient, fast, safe, and reliable construction compared with mortise-tenon connection [4–9]. As important commercial tree species in Southern China, Masson pine and Chinese fir have been widely planted by virtue of their high growth rate, strong updating capability, and high economic benefits [10–12]. At present, the nail holding performance parameters of Masson pine and Chinese fir have been scarcely investigated. Hence, it is urgently necessary to explore the nail holding performance of Masson pine and Chinese fir to ensure reasonable and safe application screws in buildings and furniture with Masson pine and Chinese fir timber structures.
The nail holding strength of timbers, which is the resistance borne by screws when being pulled out of timbers, is an important performance index of timbers and artificial boards. Timber nail holding performance—a kind of heterogenous and anisotropic natural polymer materials—varies from section to section [13–17]. Self-tapping screws and round nails have been commonly used for the connection to timber structures, where round nails resist the pullout force through the frictional force between wood fibers and the nail bar, while self-tapping screws resist the pullout force through the joint action of the friction and shear against wood fibers, so self-tapping screws have better nail holding performance than round nails [18–20] and been more widely applied. Self-tapping screw connection plays a vital role in lightweight wood structure buildings, so the requirements for the nail holding performance of such structures and concrete performance indexes should be met to ensure the stable overall bearing capacity of timber structures. In Code for Design of Timber Structures (GB50005-2003), only the spacing between connection screws and the minimum thickness of timber structures are stipulated. In Method of Testing Nail Holding Power of Wood (GB/T14018-2009), only the nail holding power of ordinary round nails 2.5 mm in diameter under static loading conditions is stipulated as an index measuring wood performance, which is far from enough for the stability and reliability of timber structures. The nail holding performance of timbers is associated with the screw type [18,21], screw diameter [22–24], wood density [19,25] and moisture content [26,27], nailing angle [19], and guiding bores [25,28]. In this study, the influences of the diameter of self-tapping screws and the guiding bores on the nail holding performance at different sections of Masson pine and Chinese fir dimension lumbers were mainly explored, expecting to provide a scientific basis for designing the screw connection of Masson pine and Chinese fir.
Masson pine dimension lumbers are produced in Guiyang, aged about 25 years, with an average air-dry density of 0.49 g/cm3, and an average moisture content of 11.7%. Chinese fir dimension lumbers are produced in Guiyang, aged about 25 years, with an average air-dry density of 0.42 g/cm3, and an average moisture content of 11.5%. By reference to Method of Testing Nail Holding Power of Wood (GB/T 14018-2009), Masson pine and Chinese fir were processed into specifications of 150 mm (radial section) × 50 mm (tangential section) × 50 mm (cross section). Self-tapping screws were purchased in the market with a perfectly straight roof bar and free from defects like corrosion, deletion, and hanger-iron at the tip. The specifications of screws are exhibited in Table 1 and Fig. 1.
Figure 1: Different self-tapping screws used for nail holding strength test
According to GB/T 14018-2009, timbers were placed in an environment with constant temperature and humidity and good ventilation conditions for 6 months until their moisture content was stabilized at about 12%. Then, their density was determined, and specimens without visible defects like knots, cracking, decaying, and color change was picked out for the nail holding strength test. A total of 6 screws were screwed in the adjacent cross, radial, and tangential sections of each specimen, as shown in Fig. 2.
Figure 2: Sample diagrams for nail holding strength test
Self-tapping screws were screwed in with a screwdriver, which must keep perpendicular to the surface with a screw-in depth of 20 mm (in case of cracking, the specimen was considered to be disqualified). Within 10–60 min after screws were screwed in, the nail holding strength test was completed on a WDS-50KN universal mechanical testing machine under uniform-velocity (2.5 mm/min) loading conditions (Fig. 3). The nail holding strength was calculated through formula (1), and the final strength was the mean value of 12 specimens. The reported strength was the mean value of 24 data. The maximum and minimum values were deleted, and then the rest of the data were averaged. The standard deviation was less than 10%.
where: P denotes the nail holding strength, N/mm; Pmax is the maximum load, N; L represents the screw-in depth, mm.
Figure 3: The test of nail holding strength
3.1 Effects of the Diameter of Self-Tapping Screws on the Nail Holding Performance of Masson Pine and Chinese Fir Dimension Lumbers
Effects of the diameter of self-tapping screws on the nail holding performance of Chinese fir and Masson pine are displayed in Figs. 4 and 5. It could be observed from Fig. 4 that when the diameter of self-tapping screws grew within 2.0–3.5 mm, the nail holding strength of Masson pine gradually increased from 63.7 to 89.6 N/mm with an increase of 40.7%. As self-tapping screws were screwed into the timber, wood fibers would be subjected to shear and extrusion of self-tapping screws, and the screw pitch and threaded teeth were enlarged with the increase in the screw diameter, thus posing more sufficient embedding and extruding actions on the timber, which, to a certain extent, improved the density of wood around self-tapping screws and enlarged the contact surface between the screw thread and wood. As a result, the shear and extrusion resistance of the wood against screws was enhanced, so the nail holding strength was also greater. When the diameter of the self-tapping screws was 4.0 mm, the nail holding strength started declining, but it was still higher than that under the diameter of 2.0–3.5 mm. A possible reason was that when the diameter of self-tapping screws grew to 4.0 mm, they formed strong extrusion to the timber, which was subjected to internal local cracks due to plastic cracking, along with the decline of nail holding strength.
Figure 4: Diameter of self-tapping screws on the nail holding strength of Masson pine and Chinese fir
Figure 5: Diameters of self-tapping screws on CVs of nail holding strength of Masson pine and Chinese fir
When the diameter of self-tapping screws was 2.0–4.0 mm, the nail holding strength of Chinese fir presented a similar variation trend to Masson pine, increasing from 50.7 to 85.6 N/mm with an increase of 68.8%. At 2.0–3.0 mm, the extruding action formed by screwing into the timber showed an approximately uniform-velocity growth trend, and this process belonged to an elastic stage of the stress-strain curve chart, which could be attributed to the pure elastic deformation. When the diameter of self-tapping screws was 3.0–3.5 mm, the wood density was nearby the ultimate strain point leading to fracture because of extrusion, and this process belonged to a highly elastic deformation stage, in which the nail holding strength grew rapidly. As the diameter of self-tapping screws reached 4.0 mm, the nail holding strength of both Chinese fir and Masson pine declined since the extruding action of self-tapping screws on the timber exceeded the ultimate fracture strength of the timber in this case, resulting in plastic cracking of wood fibers.
By comparing the nail holding strength of Masson pine and Chinese fir, as shown in Fig. 4, it could be known that the nail holding strength of Masson pine was always higher than that of Chinese fir. Because the gripping strength was positively correlated with the wood density, Masson pine enjoyed higher nail holding strength due to its greater density.
The coefficient of variation (CV) of nail gripping strength reflects the stability of screw connections to some extent [25,29]. It could be seen from Fig. 5 that when the diameter of self-tapping screws was 2.0–4.0 mm, the CVs of nail holding strength of both Masson pine and Chinese fir showed a declining trend at first and then tended to be stable. Considering the nail holding strength and stability, the diameter of self-tapping screws should be over 3.5 mm for the screw connection of Masson pine and Chinese fir.
3.2 Nail Holding Performance of Three Sections of Masson Pine and Chinese Fir
The nail holding performance test results of different sections of Chinese fir and Masson pine are displayed in Figs. 6 and 7. The nail holding strength of Masson pine on the cross, radial, and tangential sections was 39.9, 60.3, and 71.4 N/mm and sorted as tangential section > radial section > cross section, and their CVs were sorted as cross section > radial section > tangential section. Wood fibers presented a parallel arrangement in the longitudinal direction, fibers on the cross section bonding with hydrogen bond, and the self-tapping screws screwed from the cross section were parallel to wood fibers. In this case, the nail holding strength mainly depended on the extrusion force between wood fibers, so it was low. The self-tapping screws screwed in from radial, and tangential sections would generate a shear action on wood fibers, and the nail holding strength on radial and tangential sections mainly depended on the shear resistance of wood fibers, so the nail holding strength on radial and tangential sections was significantly higher than that on the cross section. The nail holding strength on the radial section was smaller than that on the tangential section for the following reasons. (1) Earlywood and latewood differences between radial and tangential sections: Latewood density and shear strength were greater than earlywood density and shear strength. On the radial section, earlywood and latewood were connected in series, but on the tangential section, they were parallelly connected (Fig. 8). On the radial section, therefore, screws directly penetrated earlywood or latewood. On the tangential section, screws penetrated into earlywood and latewood in a continuous and successive way. Hence, the nail holding strength on the tangential section was higher than that on the radial section. (2) Lignin content differences between radial and tangential sections: Lignin, an amorphous substance, endows wood with deformation resistance. In general, the lignin content of the radial cell wall of wood fibers is higher than that in the radial cell wall [14,30–32]. Therefore, the nail holding strength on the radial section was lower than that on the tangential section.
Figure 6: Nail holding strength on cross-, radial-and tangential section of Masson pine and Chinese fir
Figure 7: CVs of nail holding strength on cross, radial and tangential section of Masson pine and Chinese fir
Figure 8: Section views of nail holding tests on the three sections of Masson pine and Chinese fir
The nail holding strength of Chinese fir on cross, radial, and tangential sections was 37.9, 56.1, and 63.4 N/mm, respectively, and the strong trend on the three sections was identical with that of Masson pine, namely the nail holding strength was sorted as tangential section > radial section > cross section, and the CVs were sorted as cross section > radial section > tangential section. On the same section, however, the nail holding strength of Masson pine was always higher than that of Chinese fir, being 8.0, 4.2, and 2.0 N/mm higher than that of Chinese fir on tangential, radial, and cross sections, respectively, which was related to the large density and high latewood rate of Masson pine (Fig. 8).
3.3 Effects of Guiding Bores on the Nail Holding Performance of Masson Pine and Chinese Fir
At the screw connection position on the radial section of Chinese fir and Masson pine, guiding bores 1.8 mm in diameter and 20 mm in depth were preprocessed, followed by the nail holding strength test, with results displayed in Figs. 9 and 10.
Figure 9: Effects of guiding bores on the nail holding strength of Masson pine and Chinese fir
Figure 10: Effects of guiding bores on the CVs of nail holding strength of Masson pine and Chinese fir
As the diameter of self-tapping screws gradually grew within 2.0–4.0 mm, the nail holding strength of Masson pine with guiding bores gradually increased, while that of Masson pine without guiding bores increased initially and then decreased (the maximum value appeared under the diameter of 3.5 mm). As the above analysis, the decline in nail holding strength was attributed to plastic cracking. Hence, guiding bores could mitigate the influence of plastic cracking on nail holding strength.
When the diameter of self-tapping screws was 2.0 and 2.5 mm, the nail holding strength of Masson pine with guiding bores was smaller than that without guiding bores. At 3.0, 3.5, and 4.0 mm, the nail holding strength of Masson pine with guiding bores was 71.9, 85.5, and 87.8 N/mm, respectively, while that without guiding bores was 67.5, 79.8, and 74.3 N/mm, respectively, so the nail holding strength of Masson pine with guiding bores was always higher than that without guiding bores. As the diameter increased, the amplitude was elevated with an increased rate of 6.5%, 7.1%, and 18.2%, respectively. The reason was that at a small diameter of self-tapping screws, it was easy to screw in wood, the specimens with guiding bores presented insufficient shear and extrusion with wood fibers around screws, and the nail pullout of self-tapping screws generated relatively minor shear failure to surrounding wood fibers. With the increase in the diameter of self-tapping screws, the screw pitch and threaded teeth were enlarged and showed sufficient shear and extrusion with surrounding wood fibers so as to cause the rapid growth of frictional force induced by shear force and extrusion. The specimens without guiding bores cracked, to different extents, at 3.0, 3.5, and 4.0 mm, while those with guiding bores did not experience obvious plastic cracking, so the nail holding strength was significantly improved.
Guiding bores generated an identical influence on Chinese fir’s nail holding strength with that of Masson pine’s nail holding strength. It was not until the diameter of self-tapping screws was 3.5 and 4.0 mm that the nail holding strength of Chinese fir with guiding bores started exceeding that without guiding bores, which was related to the high elastic modulus of Chinese fir. As shown in Fig. 10, when the diameter of self-tapping screws was 4.0 mm, the CVs of nail holding strength of both Masson pine and Chinese fir with guiding bores were smaller than that without guiding bores, indicating that at a large diameter of self-tapping screws, guiding bores could, to some extent, enhance the nail holding strength and its stability. The influences of the depth and diameter of guiding bores on the nail holding strength of Chinese fir and Masson pine will be further investigated.
3.4 Load-Displacement Curves of Nail Holding Test
Fig. 11 displays the load-displacement curves obtained through the nail holding strength test on the cross, radial, and tangential sections of Chinese fir and Masson pine at the diameter of 2.0–4.0 mm of self-tapping screws.
Figure 11: Load-displacement curves obtained through nail holding tests of Chinese fir and Masson pine
Cross section: The load-displacement curve rose at first and then declined. After it declined to a certain value, continuous round blunt short peaks were generated as the displacement continued to grow, and the greater the diameter of self-tapping screws, the longer the short peak period. This was because the nail holding strength of the cross section mainly derived from the extrusion between wood fibers, namely, the static frictional force between self-tapping screws and fibers. When screws were pulled out and after the nail holding strength reached the limit value, the wood fibers embedded into the threaded portion and adjacent fibers gradually fractured and sideslipped, and the static frictional force was turned into the dynamic frictional force, which gradually declined to a certain extent and was turned into the static frictional force again. During the process, the static and dynamic frictional force were continuously mutually transformed, the load-displacement curve fluctuated up and down, and more short peaks were formed. As self-tapping screws were gradually pulled out, the contact area between nail bars and wood gradually diminished, and short peaks gradually declined.
Radial section and tangential section: When the diameter of self-tapping screws was 2.0 and 2.5 mm, the load-displacement curve increased initially and then declined slowly. This was because when the diameter was small, the screw pitch was small, generating a minor shear force to the wood, and the shear force was equivalent to the extrusion force [28,33]. When the nail holding strength reached the maximum value, wood fibers were sheared, slidded, and peeled off, and in this case, a frictional force equivalent to the shear force still existed, so the load-displacement curve slowly declined. When the diameter of self-tapping screws was 3.0, 3.5, and 4.0 mm, the load-displacement curve grew at first and then declined rapidly. The reason was that at a large diameter of self-tapping screws, the screw pitch increased and generated a large shear force towards the wood, and the shear resistance of wood fibers dominated the nail holding strength. When screws were pulled out and after the nail holding strength reached its limit value, wood fibers were sheared, and the nail holding strength was rapidly weakened.
3.5 The Phenomena during Nail Holding Test
It was found in the test process that as the diameter of self-tapping screws increased, wood cracking tended to occur on the radial and tangential sections, and as self-tapping screws were pulled out, the peeling degree on the wood surface was gradually aggravated (Figs. 12 and 13). The cracking phenomenon of Masson pine was more obvious than that of Chinse fir, especially when the diameter of self-tapping screws was 4.0 mm. The qualified specimens dropped remarkably. At a large diameter, the cracking and fiber peeling phenomena of Masson pine and Chinese fir with prefabricated guiding bores were obviously improved during the test, and the nail holding strength was further enhanced. Without guiding bores, the nail holding strength on the tangential section of Masson pine was 51.2 N/mm at a diameter of 2.0 mm, and it reached the maximum value of 79.8 N/mm at 3.5 mm; for Chinese fir, the nail holding strength on the tangential section was 45.0 N/mm at a diameter of 2.0 mm, and reached the maximum value of 80.5 N/mm at the diameter of 3.5 mm. With guiding bores, the nail holding strength of Masson pine was 85.5 N/mm at the diameter of 3.5 mm, and reached the maximum value of 87.8 N/mm at the diameter of 4.0 mm; for Chinese fir, its nail holding strength reached the peak value of 81.6 N/mm at 3.5 mm, and reached the maximum value of 85.3 N/mm at the diameter of 4.0 mm.
Figure 12: Failure appearance on Masson pine under different screw diameters and sections
Figure 13: Failure appearance on Chinese fir under different screw diameters and sections
Despite the partially higher physical properties like the density of Masson pine than those of Chinese fir, Masson pine dimension lumbers, which were relatively hard, were more prone to cracking when large-diameter self-tapping screws were screwed in their radial and tangential sections. Hence, prefabricated guiding holes were more essential for the screw connection of Masson pine.
The influences of the diameter of self-tapping screw and the guiding bores on the nail holding performance on different sections of Masson pine and Chinese fir were mainly explored, aiming to provide a scientific basis for the screw connection design of Masson pine and Chinese fir timber structures in this study. The results showed that:
(1) The nail holding strength of radial and tangential sections mainly depended on the shear resistance of wood fibers, but due to the earlywood and latewood differences and lignin content differences, the nail holding strength of the tangential section was the maximum, followed by that of the radial section. The extrusion force mainly decided the nail holding strength of the cross section between wood fibers, which was the minimum.
(2) Due to the higher density, the nail holding strength of Masson pine was higher than that of Chinese fir.
(3) The nail holding strength grew with the increase in the diameter of self-tapping screws, but a large diameter would lead to plastic cracking of the timber, thus further affecting the nail holding strength. Masson pine and Chinese fir reached the maximum nail holding strength when the diameter of self-tapping screws was 3.5 mm.
(4) Under a large diameter of screws, prefabricated guiding bores could mitigate timber cracking and improve its nail holding strength.
(5) Masson pine was very prone to cracking if self-tapping screws were screwed in, so prefabricated guiding bores were more necessary for the screw connection of Masson pine.
Funding Statement: This work was funded by the National Natural Science Foundation of China (32160348), Forestry Science and Technology Research Project of Guizhou Forestry Bureau (J[2022]21 and [2020]C14), Department Program of Guizhou Province ([2020]1Y128), the Cultivation Project of Guizhou University of China ([2019]37).
Conflicts of Interest: The authors declare that they have no conflicts of interest to report regarding the present study.
References
1. Berwart, S., Estrella, X., Montaño, J., Santa-María, H., Almazán, J. L. et al. (2022). A simplified approach to assess the technical prefeasibility of multistory wood-frame buildings in high seismic zones. Engineering Structures, 257, 114035. https://doi.org/10.1016/j.engstruct.2022.114035 [Google Scholar] [CrossRef]
2. Li, H., Wang, B., Wei, P., Wang, L. (2019). Cross-laminated timber (CLT) in China: A state-of-the-art. Journal of Bioresources and Bioproducts, 4(1), 22–31. https://doi.org/10.21967/jbb.v4i1.190 [Google Scholar] [CrossRef]
3. Wu, G., Zhong, Y., Gong, Y., Ren, H. (2022). The calculation method of stability coefficient of CLT members in axial compression based on the effective slenderness ratio. Journal of Forestry Engineering, 7(1), 59–65. https://doi.org/10.13360/j.issn.2096-1359.202105008 [Google Scholar] [CrossRef]
4. Wei, P., Wang, B. J., Li, Z., Ju, R. (2020). Development of cross-laminated timber (CLT) products from stress graded Canadian hem-fir. Wood Research, 65 (2), 335–346. https://doi.org/10.37763/wr.1336-4561/65.2.335346 [Google Scholar] [CrossRef]
5. Dúbravská, K., Tereňová, L., Štefková, J. (2020). CLT construction performance under thermal loading. Wood Research, 65(4), 605–614. https://doi.org/10.37763/wr.1336-4561/65.4.605614 [Google Scholar] [CrossRef]
6. Yang, Z., Xia, H., Tan, J., Feng, Y., Huang, Y. (2020). Selection of superior families of Pinus massoniana in southern China for large-diameter construction timber. Journal of Forestry Research, 31(2), 475–484. https://doi.org/10.1007/s11676-018-0815-2 [Google Scholar] [CrossRef]
7. Wang, Y., Lee, S. H. (2018). A theoretical model developed for predicting nail withdrawal load from wood by mechanics. European Journal of Wood and Wood Products, 76(3), 973–978. https://doi.org/10.1007/s00107-017-1227-2 [Google Scholar] [CrossRef]
8. Zhang, B., Wu, Z., Liang, J., Yu, L., Xi, X. et al. (2022). Effects of polyethylene glycol on the flexibility of cold-setting melamine-urea-formaldehyde resin. European Journal of Wood and Wood Products, 80, 975–984. https://doi.org/10.1007/s00107-022-01801-x [Google Scholar] [CrossRef]
9. Zhang, L., Chen, Z., Dong, H., Fu, S., Ma, L. et al. (2021). Wood plastic composites based wood wall’s structure and thermal insulation performance. Journal of Bioresources and Bioproducts, 6(1), 65–74. https://doi.org/10.1016/j.jobab.2021.01.005 [Google Scholar] [CrossRef]
10. Wang, S., Hou, J., Jiang, Z., Yu, Y. (2022). Study on periodic hot-press drying and surface densification of Chinese fir lumber. Journal of Forestry Engineering, 7(3), 40–45. https://doi.org/10.13360/j.issn.2096-1359.202108012 [Google Scholar] [CrossRef]
11. Wang, F., Liu, J., Lyu, W. (2019). Effect of boron compounds on properties of Chinese fir wood treated with PMUF resin. Journal of Bioresources and Bioproducts, 4(1), 60–66. https://doi.org/10.21967/jbb.v4i1.182 [Google Scholar] [CrossRef]
12. Wu, H., Lei, J., Li, X., Wang, H., Duan, A. et al. (2021). Aggregation distributions across stand age in provenances of Cunninghamia lanceolata (Lamb.) hook. Forest Ecology and Management, 494, 119317. https://doi.org/10.1016/j.foreco.2021.119317 [Google Scholar] [CrossRef]
13. Song, J., Chen, C., Zhu, S., Zhu, M., Dai, J. et al. (2018). Processing bulk natural wood into a high-performance structural material. Nature, 554(7691), 224–228. https://doi.org/10.1038/nature25476 [Google Scholar] [PubMed] [CrossRef]
14. Zhao, R. J., Fei, B. H., Guo, W., Zhou, H. B. (2010). Nail withdrawal strength of Chinese fir dimension lumbers. Journal of Building Materials, 13(4), 463–467. https://doi.org/10.3969/j.issn.1007-9629.2010.04.009 [Google Scholar] [CrossRef]
15. Xia, T., Zhou, Y., Zheng, W., Dong, L., Wang, Z. et al. (2022). Failure mode and damage assessment of the midply-bamboo shear walls. Journal of Forestry Engineering, 7(3), 53–59. https://doi.org/10.13360/j.issn.2096-1359.202109018 [Google Scholar] [CrossRef]
16. Liu, J., Yu, P., Wang, D., Chen, Z., Cui, Q. et al. (2020). Wood-derived hybrid scaffold with highly anisotropic features on mechanics and liquid transport toward cell migration and alignment. ACS Applied Materials & Interfaces, 12(15), 17957–17966. https://doi.org/10.1021/acsami.0c00646 [Google Scholar] [PubMed] [CrossRef]
17. Barcík, Š., Gašparík, M., Horejš, P. (2014). Influence of thermal modification on nail withdrawal strength of spruce wood. BioResources, 9(4), 5963–5975. https://doi.org/10.15376/biores.9.4.5963-5975 [Google Scholar] [CrossRef]
18. Rammer, D. R., Winistorfer, S. G., Bender, D. A. (2001). Withdrawal strength of threaded nails. Journal of Structural Engineering, 127(4), 442–449. https://doi.org/10.1061/(asce)0733-9445(2001)127:4(442) [Google Scholar] [CrossRef]
19. Teng, Q. C., Wang, F. B., Que, Z. L., Zeng, N. (2020). Effects of angles on the screw and nail withdrawal strength in dimension lumber. Scientia Silvae Sinicae, 56(1), 154–161. https://doi.org/10.11707/j.1001-7488.20200115 [Google Scholar] [CrossRef]
20. Celebi, G., Kilic, M. (2007). Nail and screw withdrawal strength of laminated veneer lumber made up hardwood and softwood layers. Construction and Building Materials, 21(4), 894–900. https://doi.org/10.1016/j.conbuildmat.2005.12.015 [Google Scholar] [CrossRef]
21. Aytekin, A. (2008). Determination of screw and nail withdrawal resistance of some important wood species. International Journal of Molecular Sciences, 9(4), 626–637. https://doi.org/10.3390/ijms9040626 [Google Scholar] [PubMed] [CrossRef]
22. Khai, T. D., Young, J. G. (2022). Withdrawal capacity and strength of self-tapping screws on cross-laminated timber. Structures, 37, 772–786. https://doi.org/10.1016/j.istruc.2022.01.033 [Google Scholar] [CrossRef]
23. Dong, C., Zhang, S., Wang, J., Chui, Y. (2021). Static bending creep properties of furfurylated poplar wood. Construction and Building Materials, 269, 121308. https://doi.org/10.1016/j.conbuildmat.2020.121308 [Google Scholar] [CrossRef]
24. Gehloff, M. (2011). Pull-out resistance of self-tapping wood screws with continuous thread (Master Thesis). The University of British Columbia, Canada. [Google Scholar]
25. Brandner, R. (2019). Properties of axially loaded self-tapping screws with focus on application in hardwood. Wood Material Science & Engineering, 14(5), 254–268. https://doi.org/10.1080/17480272.2019.1635204 [Google Scholar] [CrossRef]
26. Gutknecht, M. P., MacDougall, C. (2019). Withdrawal resistance of structural self-tapping screws parallel-to-grain in common Canadian timber species. Canadian Journal of Civil Engineering, 46(10), 952–962. https://doi.org/10.1139/cjce-2018-0374 [Google Scholar] [CrossRef]
27. Akyildiz, M. H. (2014). Screw-nail withdrawal and bonding strength of paulownia (Paulownia tomentosaSteud.) wood. Journal of Wood Science, 60(3), 201–206. https://doi.org/10.1007/s10086-014-1391-5 [Google Scholar] [CrossRef]
28. Que, Z., Yang, L., Wang, F., Zhu, X., Pan, B. (2014). Effects of vessel diameter on screw withdrawal strength in dimension lumber of wood structure. Journal of Northwest Forestry University, 30(5), 195–198. https://doi.org/10.3969/j.issn.1001-7461.2014.05.37 [Google Scholar] [CrossRef]
29. Ceylan, A., Girgin, Z. C. (2020). Comparisons on withdrawal resistance of resin and phosphate coated annular ring nails in CLT specimens. Construction and Building Materials, 23(30), 117742. https://doi.org/10.1016/j.conbuildmat.2019.117742 [Google Scholar] [CrossRef]
30. Zhang, J. T., Wang, Z. Q., Zhao, T., Liu, Y. X., Dong, W. Q. (2020). Study on shear performance of 3-layer CLT T&G joints under monotonic loading. Journal of Forestry Engineering, 5(4), 67–72. https://doi.org/10.13360/j.issn.2096-1359.201910014 [Google Scholar] [CrossRef]
31. Wu, G. F., Zhu, E. C., Zong, Y., Gong, Y. C., Ren, H. Q. (2018). Development and investigation of a hybrid built-up column made of small diameter logs originating from juvenile trees. Journal of Wood Science, 64(4), 356–363. https://doi.org/10.1007/s10086-017-1689-1 [Google Scholar] [CrossRef]
32. Abdoli, F., Rashidi, M., Rostampour-Haftkhani, A., Layeghi, M., Ebrahimi, G. (2022). Withdrawal performance of nails and screws in cross-laminated timber (CLT) made of poplar (Populus alba) and fir (Abies alba). Polymers, 14(15), 3129. https://doi.org/10.3390/polym14153129 [Google Scholar] [PubMed] [CrossRef]
33. Deng, L., Wang, Z., Zhao, R., Zhou, X., Wu, G. (2017). Research on nail withdrawal strength of three species of dimension lumbers. China Forest Products Industry, 44(12), 7–10+27. https://doi.org/10.19531/j.issn1001-5299.201712002 [Google Scholar] [CrossRef]
Cite This Article
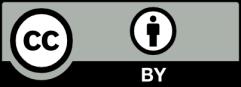
This work is licensed under a Creative Commons Attribution 4.0 International License , which permits unrestricted use, distribution, and reproduction in any medium, provided the original work is properly cited.