Open Access
ARTICLE
Preparation and Sustained Release of 2-Hydroxyethyl Methacrylate -N-Vinyl-2-Pyrrolidone Hydrogel for Ophthalmic Drug
1 State Key Laboratory of Separation Membranes and Membrane Processes, School of Materials Science and Engineering, Tiangong University, Tianjin, 300380, China
2 Department of Ophthalmology, Tianjin Children’s Hospital, Tianjin, 300400, China
3 Department of Materials Engineering, Hebei Construction Material Vocational and Technical College, Qinhuangdao, 066004, China
* Corresponding Authors: Yabin Zhang. Email: ; Lihua Wu. Email:
Journal of Polymer Materials 2024, 41(4), 263-279. https://doi.org/10.32604/jpm.2024.055821
Received 08 July 2024; Accepted 27 September 2024; Issue published 16 December 2024
Abstract
Eye drops are the usual method to treat eye diseases, and gel polymer provides the carrier which could be used for ophthalmic drug delivery. However, the stable and sustained release is the main issue for the ophthalmic drug and is concerned by many researchers. In this study, composite hydrogels were prepared using 2-hydroxyethyl methacrylate (HEMA), and N-vinyl-2-pyrrolidone (NVP) used for ophthalmic drug loading and release. According to the results of Fourier transform infrared spectroscopy (FTIR), the disappearance of the C=C bond in hydrogel indicated that the copolymerization reaction between HEMA and NVP was successful. The hydrogel was opaque and uniform, showing that the cross-linking process was random and even. With the addition of NVP, the water contact angle decreased, and the equilibrium water content increased. Due to the high porosity, the tensile strength of the hydrogel was reduced. The results of X-ray photoelectron spectroscopy showed that Naphazoline eye drops (NEDs) were adsorbed by the obtained hydrogel. The transmittance was above 92% when NEDs were loaded in the hydrogel. The addition of NVP improved the drug loading capacity with a maximum of 80.7 μg/g. When the 10-h release was performed, the cumulative release amount reached about 70% of the total adsorption amount. Composite hydrogel with 10% NVP showed lower cumulative release compared to poly(2-hydroxyethyl methacrylate) (pHEMA) when release operation was performed below 6 h. The linear release of hydrogel with NEDs showed the possibility of clinical application.Graphic Abstract
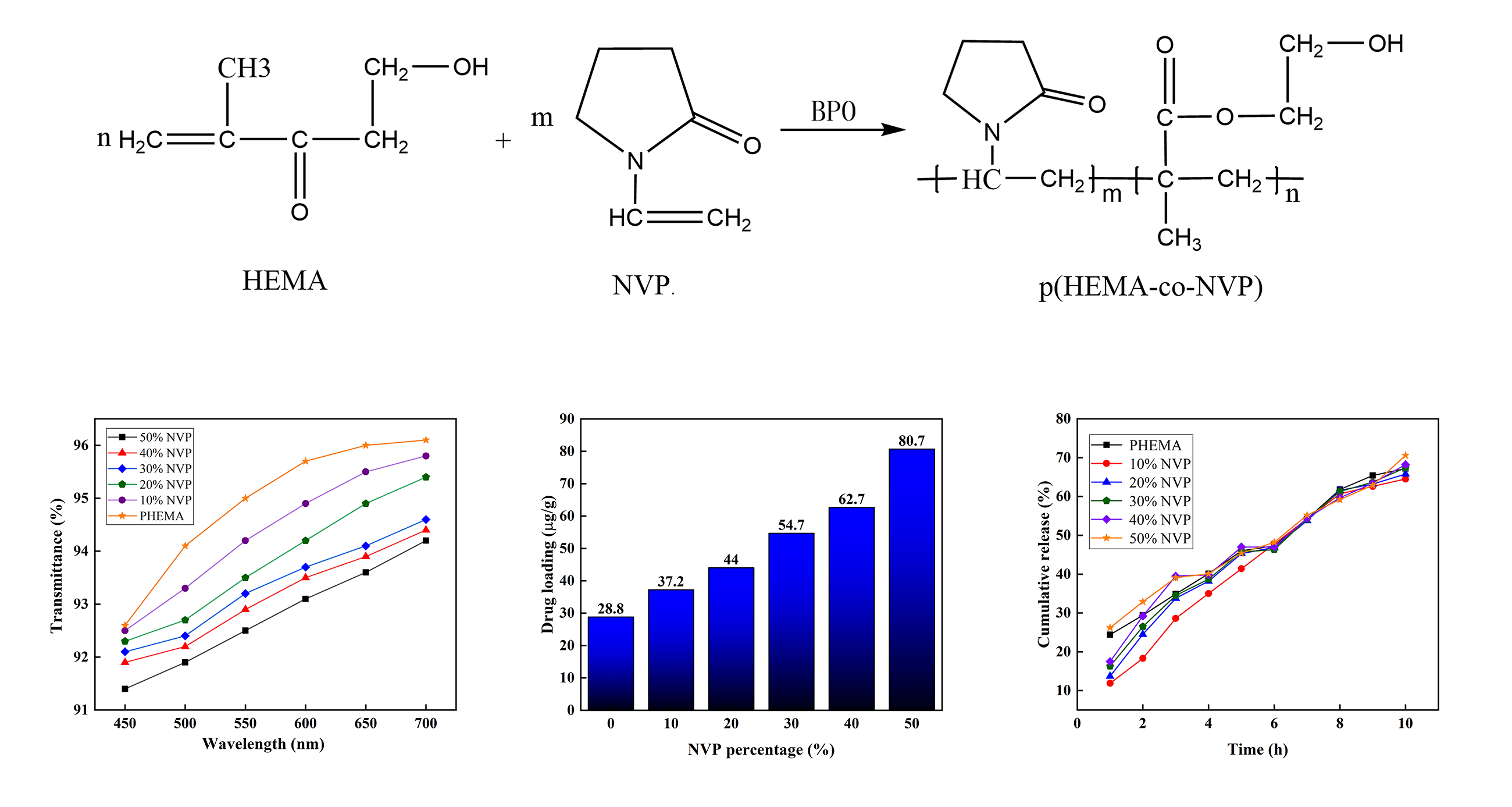
Keywords
As an uncovered organ, the eye could easily be injured by various ocular diseases, including glaucoma, diabetic retinopathy, cataract, dry eye syndrome and so on [1]. About 3.45% of the total 7.33 billion people in the world, 252.6 million, suffered from vision impairment and blindness in 2015 according to Nguyen’s description and the number was increased at an accelerating rate [2]. Multiple ocular drug delivery and treatment strategies have been developed, including eye drops [3,4] injections [5–7], and contact lenses [8–10]. Traditional ocular drug delivery is generally achieved through injection and eye drops. Drugs could enter eyes via intraocular injection. However, the invasiveness of injection is a key issue that seriously affects patient compliance [11,12]. Eye drops are considered the most important initial medical treatment and the most convenient method for treating eye diseases [13]. However, eye drops are easily washed away by blinking and tear reflux, so drug with high concentrations is required usually and used frequently. It leads to the inconvenience and discomfort for patients [14,15].
Over the past few decades, ophthalmic drug delivery methods have been developed to overcome the limitations of eye drops and injected drugs [16,17]. The popular process is to extend drug release or increase the holding time on the eye surface. New ophthalmic formulations [18], such as mucosal adhesive polymers, gel polymers, nanoparticles, implants, or microneedles have been developed. Mun et al. investigated the formulations of clonidine hydrochloride prepared using Poloxamer 188, poloxamer 407, and HPMC K4M. A drug release study showed that in situ gels with 16% poloxamer 407 remained releasing the drug for 10 h. However, too viscous formulations would cause eye irritation and blurred vision. The pleasant, safe, and effective formulations were claimed without in vivo tests [19]. Lewis et al. developed a sustained-release implant delivery system for Bimatoprost SR and compared the intraocular pressure of patients with open angle glaucoma. After 12 weeks, the mean change of intraocular pressure in the implant group seemed to be better than that in the eye drop group. Overall, 52% of patients using implants reported adverse application of hydrogel implant [20].
HEMA or pHEMA-based hydrogel was studied by many researchers because it exhibited excellent biocompatibility [21], low toxicity [22], clinical translation [23], and so on. As a result, the soft pHEMA-based hydrogel is considered being as a safe and comfortable carrier during ophthalmic treatments [24,25] and provides the possibility of clinical translatability. Consequently, the contact lens originating from pHEMA-based hydrogel is especially suitable for ocular drug delivery [26,27]. Contact lenses can successfully reduce drug washout resulting from tears. In addition, plenty of pores in hydrogel contact lenses are helpful for the sustained release of drugs. Furqan et al. prepared hydrogels with timolol using commercial HEMA, and the obtained hydrogels showed low drug loading capacity and high release amount in the initial stage [28]. Ankita et al. designed a novel semicircular ring including timolol and hyaluronic acid, and implanted it into a contact lens. Although the contact lens could retain the release rate which could meet the therapeutic requirement, the in vivo pharmacodynamics studies showed that its drug loading capacity was lower than that of single eye drop [29]. Joseph et al. developed a contact lens implanted the latanoprost-loaded film. The delivery time, above 30 days, was prolonged for the contact lens. However, the properties including mechanical strength, water content, ion and oxygen permeability were decreased after long time delivery treatment [30]. Many researchers studied the effect of NVP on the contact lens. Wang et al. coated polymerized NVP and poly (ethylene glycol) methacrylate (PEGMA) on the lens. The water contact angle of the NVP/PEGMA-coated lens was decreased obviously, and excellent hydrophilicity and significantly lower protein adsorption were obtained [31]. Sun et al. explored the impact of silane-modified silica nanoparticles and NVP on the properties of HEMA corneal contact lens, and they found that NVP could help to increase the hydrophilicity of the lens [32]. The above approaches showed that the contact lens could be used to sustain the release of ophthalmic drugs. However, some issues, e.g., high initial burst release, and unstable properties, need to be addressed in drug delivery using HEMA-based hydrogels.
To overcome the issues, a binary copolymer hydrogel was prepared using HEMA as the main matrix. NVP was copolymerized with HEMA to improve the surface hydrophilicity of the copolymer hydrogel. The effects of NVP addition on the porosity, equilibrium water content, tensile strength, and light transmittance were studied. The loading and sustained release of hydrogel were also investigated.
Ophthalmic grade HEMA and NVP were supplied by Tianjin Xiens Reagent Co., Ltd., Tianjin, China. Benzoyl peroxide (BPO) was obtained from Chengdu Kelong Chemical Reagent Factory, Chengdu, China. These reagents were analytic and used without further purification. Naphazoline Eye Drops containing naphazoline hydrochloride, chiorphenamine maleate, and vitamin B12 were purchased from Jiangxi Shanliang Pharmaceutical Co., Ltd., China. Distilled water was provided by Tianjin Lanyang Industrial Water Management Office, Tianjin, China.
The hydrogels were obtained via a polymerization reaction between NVP and HEMA monomers in the presence of BPO. The O-O bond in BPO could provide free radicals which are helpful for the copolymerization reaction between HEMA and NVP [33]. Firstly, NVP and HEMA were mixed at room temperature, and mixtures were obtained. The weight ratios of NVP and HEMA were 0:100, 10:90, 20:80, 30:70, 40:60, and 50:50, respectively. Then, BPO was added into the mixtures as an initiator and cross-linking agent, and the weight ratio was 0.25 wt% of the above mixture. Subsequently, the mixture was sealed and stirred for 40 min until the BPO was dissolved completely. As a result, the precursor solution was prepared. The precursor was kept without air for 30 min. After that, the uniform precursor solutions were poured into a mold and placed in a vacuum oven at 85°C for 2.5 h. Polymerization took place until transformation to hydrogel was completed. After that, the hydrogels were dried at 60°C for 24 h to remove the water in the samples. Table 1 lists the weight ratios of raw materials.
Fourier transform infrared spectrometer (FTIR, Nicolet iS50, Thermo Fisher Scientific, Waltham, MA, USA) was used to analyze the chemical reaction in the hydrogels in the wavenumber range of 400–4000 cm−1. The surface microstructure of pHEMA and p(HEMA-co-NVP) copolymer was observed by scanning electron microscope (SEM, phenom XL, Phenom-World, Eindhoven, Netherlands). The accelerating voltage of 2 kV was selected. The element composition of hydrogels was determined using X-ray photoelectron spectroscopy (XPS, NEXSA, Thermo Fisher, Waltham, MA, USA). The water contact angles of the pHEMA hydrogel and p(HEMA-co-NVP) composite hydrogel in the air were measured using a dynamic contact angle measurement instrument (DSA-100, KRUSS, Hamburg, Germany). The swollen hydrogel samples were cut into squares with the size of 10 mm × 10 mm. In order to keep the pore structure, the freeze-drying process was performed in advance using liquid nitrogen. A 2 μL water droplet was dropped onto the sample, three different positions were selected on each hydrogel sample, and the average value was calculated to obtain the water contact angle. The porosity of the hydrogel was determined based on the Archimedes principle. The measurement process has been described elsewhere [34,35]. Three samples with the same NVP addition were used to detect the porosity and the average value was calculated.
The tensile strength, elongation at break, and optical transparency were also measured for saturated hydrogel. The samples were immersed in physiological saline solution for 6 h until the weight was kept stable, and the saturated hydrogel samples were obtained. The tensile strength and elongation at break were measured using an electronic universal testing machine (SES-1000, Shimadzu, Nanjing, China) at room temperature. The tensile speed was 10 mm/min. Before the mechanical measurement, the saturated hydrogel samples were sheared into dumbbell shapes. The effect of NVP content on optical transparency was also studied. The absorbance of the hydrogel was measured using a UV-visible spectrophotometer (UV-2600i, Shimadzu, Nanjing, China) at wavelengths of 450, 500, 550, 600, 650, and 700 nm. The physiological saline solution was selected as the reference solution during the optical transparency measurement.
The equilibrium water content of hydrogel was also detected. The dry hydrogel sample was weighed and then soaked in deionized water for 24 h until its weight did not change. The weights before and after swelling were named Md and Ms, respectively. Each sample was tested three times to obtain the average value. The equilibrium water content of the hydrogel was calculated using Eq. (1):
where SR represents the equilibrium water content, Md and Ms are the weights of the hydrogel before and after swelling.
The Naphazoline Eye Drops (NEDs) were diluted using deionized water, and the weight ratio of NEDs and deionized water was 1:1. Dry hydrogel was immersed in diluted NEDs solution, and kept for 24 h at room temperature. Drug loading measurement was performed at a dark place to avoid NEDs oxidation. The UV absorbance measurement was performed using a UV-visible spectrophotometer before and after the absorption experiment, respectively. The strongest adsorption peak at a specific wavelength was detected for the NEDs solution. The obtained adsorption curve was shown in Fig. 1, and the wavelength, 361 nm, was used to measure the transmittance of NEDs solution with a series of concentrations. After that, the transmittance value was fitted with the content of NEDs, and a straight line was obtained. As a result, the concentration of NEDs solution in our experiment was deduced according to the plotted line and was used to calculate the drug loading capacity and cumulative release percentage of the hydrogel.
Figure 1: Adsorption curve of NEDs solution
The drug loading of hydrogel was calculated using the Eq. (2):
where Q represents the loading capacity, W0 is the dry weight of the hydrogel, C1 and C2 refer to the concentration of NEDs solution before and after the absorption test, V1 and V2 are the volume of NEDs solution before and after the absorption test, respectively.
PBS buffer solution with a pH of 7.4 was prepared beforehand. 0.2 g KCl, 0.2 g KH2PO4, 8.0 g NaCl, and 2.9 g NaHPO4·12H2O were added into 1000 ml deionized water and the PBS buffer solution was obtained. The hydrogel sample with NEDs solution was immersed into the above buffer solution and subsequently kept at 37°C. Then, 3 ml solution was taken out every hour and used to detect the absorbance. Another 3 ml green PBS buffer solution was added to keep the solution at the same weight. The UV-visible spectrophotometer was used to measure the absorbance using the wavelength of 361 nm before and after drug releasing process. The cumulative release percentage was calculated according to Eq. (3):
where y represents the cumulative release percentage, V1 is the total volume of the PBS buffer solution, C is the drug concentration of PBS buffer solution at every hour, Ci−1 means the drug content in PBS buffer solution at the last hour, V2 is the volume of solution which is taken out from the PBS buffer solution, and m is the total mass of drug in the hydrogel.
The change of functional groups could be used to induce the polymerization reaction. Fig. 2 shows the FTIR spectra of HEMA, NVP, pHEMA, and p(HEMA-co-NVP). The related frequencies are listed in Table 2. For the NVP spectrum, the peaks at 1270 and 1030 cm−1 were attributed to the C-N bond in the phthalimide group. The absorption peaks of the C=C bond were at 1660 and 751 cm−1. There was an absorption peak at 1330 cm−1 corresponding to the C=O bond. For the HEMA spectrum, the peak at 3423 cm−1 was assigned to OH stretching vibration due to the intramolecular and intermolecular hydrogen bonding. The weak peak at 2945 cm-1 corresponded to the C-H and CH3 stretching vibrations. The peak at 1708 cm−1 was attributed to the C=O stretching vibration. The absorption peak at 1650 cm−1 was due to C=C stretching vibration. The absorption peaks at low wavenumbers could be owed to C-O or C-H bonds. Compared with HEMA spectrum, the pHEMA showed similar absorption peaks. However, the peaks at 3423 and 2945 cm−1 were disappeared. What must be mentioned was that the peak at 1650 cm−1 also vanished, indicating that the C=C bond was absent in pHEMA. Therefore, it could be concluded that the self-polymerization of HEMA took place and pHEMA had been successfully synthesized [36–38]. The spectrum of p(HEMA-co-NVP) showed different adsorption peaks. The peaks at 1702 cm−1 in the NVP spectrum and 1708 cm−1 in the HEMA spectrum became broader. The intensity of peak at 1660 cm−1 was lower obviously, implying that the amount of C=C bond was decreased. Other characterization peaks at 1150 cm−1 in HEMA and 1270 cm−1 in NVP, also existed, but they became weak obviously. The weak bands at 850 to 950 cm−1 corresponded to the out-of-plane deformation vibration of C-H in the [CH2-CH2]n group, confirming that cross-linked network structure formed in the hydrogel sample. So, it could be concluded that partial copolymerization took place and residual monomer may be present in composite hydrogel. However, self-polymerization of HEMA took place easily according to the above study. Fig. 3 exhibited the formulas of the polymerization reaction. As a result, the effect of residual monomer NVP on the hydrogel was researched in our study.
Figure 2: FTIR spectra of NVP, HEMA, pHEMA, and p(HEMA-co-NVP) hydrogel
Figure 3: (a) Reaction between HEMA and NVP, and (b) self-polymerization reaction of HEMA
Fig. 4 shows the photograph and electron micrograph of pHEMA without NVP and p(HEMA-co-NVP) composite hydrogel with 20 wt% NVP. As shown in Fig. 4a,b, the hydrogel was opaque and uniform. The pHEMA hydrogel without NVP also showed the same appearance, indicating that the self-polymerization of HEMA was successful. Fig. 4c,d exhibits the surface micrograph of the above samples, and the flat and smooth surfaces were observed. It could be deduced that the cross-linking process was random and even. Fig. 4e shows the composite hydrogel after swelling. It could be observed that the surface was still relatively smooth after swelling and no obvious defect was found. Fig. 4f demonstrates that the surface of composite hydrogel after NEDs absorption. The samples also showed surfaces without defects. The obtained hydrogels can serve as the optical material of the artificial cornea. However, the hydrophilicity, drug loading and releasing of hydrogel should be studied further.
Figure 4: Photograph and surface morphology of (a, c) Sample 0, (b, d) Sample 2, (e) Sample 2 after swelling, (f) Sample 2 after NEDs absorption
Hydrophilicity is one of the important indexes for corneal contact lenses. The water contact angle of pHEMA and p(HEMA-co-NVP) hydrogel was measured to evaluate the water wettability. Fig. 5 shows the water contact angles of hydrogels. The results indicated that pHEMA hydrogel was hydrophobic. However, the copolymer hydrogel with NVP showed more excellent hydrophilicity. The contact angle of p(HEMA-co-NVP) hydrogel decreased as the NVP content increased. This result could be explained by the presence of the hydrophilic amide group in the NVP, where the oxygen atom was exposed and can interact with polar water molecules. This copolymerization interaction enhanced the combination of water and gel, and facilitated the formation of hydration groups on the hydrogel surface. The hydrophilicity of p(HEMA-co-NVP) hydrogel could lead to greater absorption and wettability of eye drops.
Figure 5: Water contact angle with different NVP content
The porosity determines the equilibrium water content (EWC), oxygen permeability and mechanical strength of the soft lens. High porosity increases the water content. Oxygen could be delivered via the water which is swelled in the cross-linked network. As a result, high porosity provides the feasibility that more oxygen is transferred from air to the cornea, resulting in that the comfortability for the wearer is improved. However, what could not be negligible is that the high porosity also leads to weak mechanical strength. The hydrogel with low porosity induces inferior water content and oxygen permeability, making the wearer feel uncomfortable due to hypoxia. Therefore, suitable porosity of hydrogel is important for the wide application of corneal contact lenses.
Fig. 6 shows the effect of NVP addition on the porosities of hydrogel. The results showed that the porosity increased with the NVP content. NVP usually showed low crystallinity and chemical groups were disordered and loose. In addition, when more NVP was added, the cross-linked network structure formed due to the reaction between NVP and HEMA. The disordered arrangement and network structure in the obtained copolymer led to the increased porosity, providing the possibility of improving the equilibrium water content, and drug loading of hydrogels.
Figure 6: Porosity with different NVP content
Equilibrium water content (EWC) is a key index which has an important effect on the comfortability and safety because water provides the media to transfer oxygen. It is crucial to choose a lens with appropriate EWC for the wearer. According to Chinese National Standard GB/T 11417.1-2012, the EWC greater than 10% in lens is recommended. Fig. 7 exhibits the EWC values of hydrogels with different NVP compositions. The result indicated that the EWC of the hydrogel increased with the NVP addition. This could be explained by the cross-linked porous structure and hydrophilic groups in NVP. As polymerization of NVP with HEMA took place, cross-linking of copolymer hydrogel became weak and high porosity was obtained as mentioned in Fig. 6. Furthermore, NVP is a hydrophilic monomer due to the presence of an amide group. The oxygen atom exposed in the amide group facilitated the adsorption of polar water molecules. The complex influence resulted in an increase of the equilibrium water content for the p(HEMA-co-NVP) hydrogel. The obtained hydrogel exhibited the appropriate EWC value which met the Chinese National Standard. The EWC values ranged from 36.2% to 50.8%, which was close to the public results of commercial contact lenses, 38.6% to 59%.
Figure 7: Water content with different NVP content
Manual operation is essential for corneal contact lens. So, adequate mechanical strength should be confirmed to withstand scratches, tears, etc. Fig. 8 compares the tensile strength of pHEMA and p(HEMA-co-NVP) hydrogel. The tensile strength decreased sharply with the increase of NVP addition. When no NVP was involved in the polymerization reaction, the pHEMA hydrogel contained a main chain originating from raw material HEMA monomer and the main chain provided the tensile strength. However, after NVP joined in the polymerization reaction, the branched chain related to the main chain, and occupied the space between adjacent main chains due to the presence of a five-member ring structure in NVP. Therefore, the degree of cross-linking of the main chain in the hydrogel decreased with the increasing of NVP content, resulting in a sharp decline in the tensile strength. In accordance with the Chinese National Standard, the tensile strength obtained in our work met the mechanical requirements for soft hydrophilic contact lenses.
Figure 8: Tensile strength as a function of NVP content
Fig. 9 shows the elongation at break as a function of the NVP addition. The elongation at break increased with the NVP percentage. When the addition of NVP was 30 wt%, the elongation at break reached the maximum of 651%. However, the high addition above 30 wt% resulted in the decrease of elongation at break. The conclusion could be explained by the stretch of main chains in the hydrogel. For pHEMA hydrogel without NVP, the main chains intertwined with each other, and tensile force did not destruct the twining. As a result, the hydrogel broke at low elongation at break. As the NVP content in the hydrogel increased, more NVP was grafted on the main chains and a steric hindrance effect appeared. As a result, the main chains were separated. In other words, the intertwined branches were unfastened and the cross-linking degree of the hydrogel decreased. Consequently, the main chains could be spun out by external force and the elongation at break was enhanced. However, the intertwined branches were fully stretched out at the high addition of NVP in the hydrogel, and the low cross-linking degree was obtained. Partial macromolecular chains lost their intertwining effect, resulting in the decreasing of elongation at break.
Figure 9: Effect of NVP content on elongation at break
Good transmittance is an essential index for polymer hydrogel when it is applied as the corneal contact lens. Fig. 10a illustrates the effect of NVP composition on the visible light transmittance. The glassy pHEMA hydrogel was colorless and transparent, and the transmittance of 92.6%~96.1% was obtained in the visible light region. The data exceeded 92% which was required in Chinese National Standard for uncolored soft contact lens. Nevertheless, the transmittance of p(HEMA-co-NVP) hydrogel slightly decreased as the NVP content. When the NVP greater than 30% was added in the hydrogel, the transmittance was below 92% in the wavelength of 450–500 nm. It could be attributed to the high addition of NVP in hydrogel. When more than 30% NVP was used, the equilibrium water content, about 50%, was obtained as shown in Fig. 5. However, excessive water content meant swelling and thickening of the hydrogel, thereby resulting in the decrease of transmittance. Fig. 10b shows the transmittance of the hydrogel after NEDs absorption. Although the transmittance was decreased after the NEDs with higher concentration were used, the above 92% transmittance was still obtained even when 0.05 mg/ml NEDs were adsorbed in the hydrogel. In other words, the hydrogel with NEDs met the transmittance requirement in Chinese National Standard.
Figure 10: Transmittance as functions of (a) NVP addition and (b) concentration of NEDs
The drug loading and releasing are important for corneal contact lenses because the lens-containing drugs could improve the therapy efficiency, especially for the ophthalmic disease. The element composition of the hydrogel was studied via XPS measurement. Fig. 11 shows the XPS diagram of the copolymer hydrogel before and after drug loading. The characteristic peaks of O 1s, N 1s, and C 1s were obvious for the hydrogel without drug loading. However, the hydrogel with NEDs showed different compositions, and two new characteristic peaks corresponding to Co 1s and P 1s were found, respectively. Co and P were the representative elements of B12 included in NEDs. Therefore, the ophthalmic drug was adsorbed by the obtained hydrogel successfully.
Figure 11: XPS results of HEMA-NVP hydrogel before and after drug loading
As a traditional ophthalmic drug, NEDs were used to measure the loading capacity of hydrogel. Fig. 12 shows the drug loading results as functions of NVP percentage and treatment time. As shown in Fig. 12a, the NEDs loading capacity of pHEMA hydrogel was 28.8 μg/g. The addition of NVP improved the drug loading, and it reached 80.7 μg/g when 50 wt% NVP was used in hydrogel. The low drug loading ability of pHEMA was attributed to the hydroxyl and ether bonds. The intermolecular interaction between pHEMA and drug molecules was weak, resulting in relatively low drug adsorption. The presence of NVP in hydrogel enhanced the drug loading efficiently due to the increase of hydrophilicity and porosity. The introduction of NVP, a hydrophilic monomer, increased the swelling rate of hydrogel, and thus improved its drug adsorption. Fig. 12b exhibited the drug loading result with treatment time. The loading capacity was 30.5 mg/g when 2 h were performed. It was increased with time and reached 80.7 mg/g after 10 h. However, the drug loading results remained stable even though the treatment time was prolonged. The drug adsorption reached saturation in 10 h, indicating that the quick drug loading was possible for HEMA-based hydrogel and was beneficial for the fabrication of contact lenses with drug.
Figure 12: Drug loading with (a) NVP percentage and (b) loading time
Sustained release can improve drug utilization, making it easier for patients to improve treatment compliance. The effects of NVP addition and treatment time on the release rate are shown in Fig. 13. In the first three hours, the drug released quickly, while the rate became slow after 4 h. Usually, the working time is 8~10 h during the day. So, the cumulative release for 10 h was tested in our study. About 70% cumulative release was obtained when the sustained releasing time reached 10 h and linear release was observed according to Fig. 13. What must be mentioned was that the NEDs cumulative release of pHEMA was higher than that of composite hydrogel with 10% NVP when 6 h were performed. In other words, the release of NEDs became slow for composite hydrogel. According to the above analysis, more NVP addition meant lower transmittance as mentioned in Fig. 8, and it did not meet the requirements of Chinese National Standard. So, the p(HEMA-co-NVP) with NVP less than 30 wt% was recommended.
Figure 13: Drug cumulative release with different NVP content
The linear release trend provides a possibility that the pHEMA-based hydrogel with NEDs could be used in clinical translation. However, the drug dosage is determined by doctor according to the state of patient’s illness. So, a series of hydrogels with different drug amounts should be obtained to meet the requirements of the patient. In other words, the procedure of drug loading is professional and distinctive. As a result, the daily disposable contact lens with different drug amounts is recommended. The lens should be prepared in a factory beforehand and used to treat ophthalmic disease subsequently.
Table 3 compared the properties of pHEMA-based hydrogel in our study and lasted references. It could be concluded that the hydrophilicity was essential for hydrogels. The equilibrium water content, 40%–50%, and transparency, above 90%, were also necessary for the pHEMA-based hydrogel. However, high initial burst release should be avoided because the sustained release would be helpful for the remedy of disease.
In this study, the hydrogel with good hydrophilicity and drug loading was obtained via the introduction of NVP into HEMA. SEM observation exhibited a smooth surface and FTIR measurement showed the copolymerization reaction took place. As the content of NVP increased, the water contact angle decreased, and the hydrophilicity of the copolymer was improved. Porosity and equilibrium water content were also increased, and more channels could be provided for oxygen transmission from air to the cornea. The obtained tensile strength met the requirements of corneal materials due to the cross-linked polymer network. When no more than 30 wt% NVP was used, the light transmittance exceeded 92% which was one of the indexes included in the Chinese National Standard. The addition of NVP to HEMA gel also improved drug loading capacity, reaching up to 80.7 μg/g. Furthermore, the hydrogel exhibited linear drug release for up to 10 h approximately, and the NEDs cumulative release of composite hydrogel was lower than that of pHEMA, implying the sustained release. The copolymer with NEDs met the daily wearing requirements for patients. The obtained hydrogel exhibited the prospect of clinical application and could be selected as an artificial corneal material used for drug release in ophthalmic treatment.
Acknowledgement: The authors would like to thank the Analytical & Testing Center of Tiangong University for Scanning Electron Microscope work.
Funding Statement: The authors received no specific funding for this study.
Author Contributions: The authors confirm contribution to the paper as follows: study conception and design: Xiaojuan He; data collection: Chanyuan Li, Shaokang Wang, Jingyi Zhu; analysis and interpretation of results: Xiaojuan He; draft manuscript preparation: Xiaojuan He, Yabin Zhang, Zhen Guo, Lihua Wu. All authors reviewed the results and approved the final version of the manuscript.
Availability of Data and Materials: The datasets generated during and analyzed during the current study are available from the corresponding author on reasonable request.
Ethics Approval: Not applicable.
Conflicts of Interest: The authors declare that they have no conflicts of interest to report regarding the present study.
References
1. Gote V, Sikder S, Sicotte J, Pal D. Ocular drug delivery: present innovations and future challenges. J Pharmacol Exp Ther. 2019;370(3):602–24. doi:10.1124/jpet.119.256933. [Google Scholar] [PubMed] [CrossRef]
2. Nguyen DD, Lai JY. Advancing the stimuli response of polymer-based drug delivery systems for ocular disease treatment. Polym Chem. 2020;11(44):6988–7008. doi:10.1039/D0PY00919A. [Google Scholar] [CrossRef]
3. Singh SR, Grossniklaus HE, Kang SJ, Edelhauser HF, Ambati BK, Kompella UB. Intravenous transferrin, RGD peptide and dual-targeted nanoparticles enhance anti-VEGF intraceptor gene delivery to laser-induced CNV. Gene Ther. 2009;16(5):645–59. doi:10.1038/gt.2008.185. [Google Scholar] [PubMed] [CrossRef]
4. Zhao C, Zhang Z, Chen L, Wang F, Xu D. Effectiveness of intravitreal injection of ranibizumab for neovascular age-related macular degeneration with serous pigment epithelial detachment. Med Sci Monit. 2016;22:833–9. doi:10.12659/MSM.895528. [Google Scholar] [PubMed] [CrossRef]
5. Tahara K, Karasawa K, Onodera R, Takeuchi H. Feasibility of drug delivery to the eye’s posterior segment by topical instillation of PLGA nanoparticles. Asian J Pharm Sci. 2017;12(4):394–9. doi:10.1016/j.ajps.2017.03.002. [Google Scholar] [PubMed] [CrossRef]
6. Yellepeddi VK, Palakurthi S. Recent advances in topical ocular drug delivery. J Ocul Pharmacol Ther. 2016;32(2):67–82. doi:10.1089/jop.2015.0047. [Google Scholar] [PubMed] [CrossRef]
7. Weng YH, Liu J, Jin SB, Guo WS, Liang XJ, Hua ZB. Nanotechnology-based strategies for treatment of ocular disease. Acta Pharm Sin B. 2017;7(3):281–91. doi:10.1016/j.apsb.2016.09.001. [Google Scholar] [PubMed] [CrossRef]
8. Dixon P, Chauhan A. Effect of the surface layer on drug release from delefilcon-A (Dailies Total1 ®) contact lenses. Int J Pharm. 2017;529(1–2):89–101. doi:10.1016/j.ijpharm.2017.06.036. [Google Scholar] [PubMed] [CrossRef]
9. Gulsen D, Li CC, Chauhan A. Dispersion of DMPC liposomes in contact lenses for ophthalmic drug delivery. Curr Eye Res. 2005;30(12):1071–80. doi:10.1080/02713680500346633. [Google Scholar] [PubMed] [CrossRef]
10. Mahaling B, Katti DS. Understanding the influence of surface properties of nanoparticles and penetration enhancers for improving bioavailability in eye tissues in vivo. Int J Pharm. 2016;501(1–2):1–9. doi:10.1016/j.ijpharm.2016.01.053. [Google Scholar] [PubMed] [CrossRef]
11. Kapoor Y, Thomas JC, Tan G, John VT, Chauhan A. Surfactant-laden soft contact lenses for extended delivery of ophthalmic drugs. Biomaterials. 2009;30(5):867–78. doi:10.1016/j.biomaterials.2008.10.032. [Google Scholar] [PubMed] [CrossRef]
12. Xu J, Li X, Sun F. Cyclodextrin-containing hydrogels for contact lenses as a platform for drug incorporation and release. Acta Biomater. 2010;6(2):486–93. doi:10.1016/j.actbio.2009.07.021. [Google Scholar] [PubMed] [CrossRef]
13. Pelusi L, Mandatori D, Mastropasqua L, Agnifili L, Allegretti M, Nubile M, et al. Innovation in the development of synthetic and natural ocular drug delivery systems for eye diseases treatment: focusing on drug-loaded ocular inserts, contacts, and intraocular lenses. Pharmaceutics. 2023;15(2):625. doi:10.3390/pharmaceutics15020625. [Google Scholar] [PubMed] [CrossRef]
14. Chen YH, Zhang SM, Cui QY, Ni JH, Wang XC, Cheng XB, et al. Microengineered poly(HEMA) hydrogels for wearable contact lens biosensing. Lab Chip. 2020;20(22):4205–14. doi:10.1039/D0LC00446D. [Google Scholar] [PubMed] [CrossRef]
15. Akbari E, Imani R, Shokrollahi P, Jarchizadeh R, Keshel SH. Hydrogel-based formulations for drug delivery to the anterior segment of the eye. J Drug Deliv Sci Technol. 2023;81:104250. doi:10.1016/j.jddst.2023.104250. [Google Scholar] [CrossRef]
16. Croisfelt FM, Tundisi LL, Ataide JA, Silveira E, Tambourgi EB, Jozala AF, et al. Modified-release topical hydrogels: a ten-year review. J Mater Sci. 2019;54(16):10963–83. doi:10.1007/s10853-019-03557-x. [Google Scholar] [CrossRef]
17. Ruan L, Su MR, Qin XY, Ruan QT, Lang W, Wu MH, et al. Progress in the application of sustained-release drug microspheres in tissue engineering. Mater Today Bio. 2022;16:100394. doi:10.1016/j.mtbio.2022.100394. [Google Scholar] [PubMed] [CrossRef]
18. Xu JW, Xue YY, Hu GY, Lin TY, Gou JX, Yin T, et al. A comprehensive review on contact lens for ophthalmic drug delivery. J Control Release. 2018;281(Suppl. 1):97–118. doi:10.1016/j.jconrel.2018.05.020. [Google Scholar] [PubMed] [CrossRef]
19. Mun J, Mok JW, Jeong S, Cho S, Joo CK, Hahn SK. Drug-eluting contact lens containing cyclosporine-loaded cholesterol-hyaluronate micelles for dry eye syndrome. RSC Adv. 2019;9(29):16578–85. doi:10.1039/C9RA02858G. [Google Scholar] [PubMed] [CrossRef]
20. Lewis RA, Christie WC, Day DG, Craven ER, Walters T, Bejanian M, et al. Bimatoprost sustained-release implants for glaucoma therapy: 6-month results from a phase I/II clinical trial. Am J Ophthalmol. 2017;175(3):137–47. doi:10.1016/j.ajo.2016.11.020. [Google Scholar] [PubMed] [CrossRef]
21. Carvalho J, Moreira S, Maia J, Gama FM. Characterization of dextrin-based hydrogels: rheology, biocompatibility, and degradation. J Biomed Mater Res Part A. 2010;93A(1):389–99. [Google Scholar]
22. Cai K, Huang Y, Yang JL. Gelcasting of alumina with low-toxicity HEMA system. J Inorg Mater. 2003;18(2):343–7. [Google Scholar]
23. Lamm RJ, Pichon TJ, Huyan F, Wang X, Prossnitz AN, Manner KT, et al. Optimizing the polymer chemistry and synthesis method of poIySTAT, an injectable hemostat. ACS Biomater Sci Eng. 2020;6(12):7011–20. [Google Scholar] [PubMed]
24. Torres-Luna C, Hu N, Tammareddy T, Domszy R, Yang J, Wang NS, et al. Extended delivery of non-steroidal anti-inflammatory drugs through contact lenses loaded with Vitamin E and cationic surfactants. Cont Lens Anterior Eye. 2019;42(5):546–52. [Google Scholar] [PubMed]
25. Rueff EM. Visual discomfort and contact lens wear: a review. Cont Lens Anterior Eye. 2023;46(4):101872. [Google Scholar] [PubMed]
26. Kim TY, Lee GH, Mun JH, Cheong S, Choi I, Kim H, et al. Smart contact lens systems for ocular drug delivery and therapy. Adv Drug Deliv Rev. 2023;196:114817. [Google Scholar] [PubMed]
27. Tang L, Zhao CY, Wang XH, Li RS, Yang JR, Huang YP, et al. Macromolecular crowding of molecular imprinting: a facile pathway to produce drug delivery devices for zero-order sustained release. Int J Pharm. 2015;496(2):822–33. [Google Scholar] [PubMed]
28. Maulvi FA, Patil RJ, Desai AR, Shukla MR, Vaidya RJ, Ranch KM, et al. Effect of gold nanoparticles on timolol uptake and its release kinetics from contact lenses: in vitro and in vivo evaluation. Acta Biomaterialia. 2019;86:350–62. [Google Scholar] [PubMed]
29. Desai AR, Maulvi FA, Pandya MM, Ranch KM, Vyas BA, Shah SA, et al. Co-delivery of timolol and hyaluronic acid from semi-circular ring-implanted contact lenses for the treatment of glaucoma: in vitroandin vivoevaluation. Biomater Sci. 2018;6(6):1580–91. [Google Scholar] [PubMed]
30. Ross AE, Bengani LC, Tulsan R, Maidana DE, Salvador-Culla B, Kobashi H, et al. Topical sustained drug delivery to the retina with a drug-eluting contact lens. Biomaterials. 2019;217:119285. [Google Scholar] [PubMed]
31. Wang TJ, Lin WP, Guo SP. Increased hydrophilicity and anti-fouling effect of orthokeratology lenses coated with NVP and PEGMA by plasma-enhanced chemical vapor deposition. J Coat Technol Res. 2023;21:737–45. [Google Scholar]
32. Sun Z, Li F, Shi K, Gao YY, Duan LJ, Gao GH. Hydroxyethyl methacrylate corneal contact lenses doped with N‐Vinylpyrrolidone and silane-modified silica nanoparticles for enhanced UV absorption. ACS Appl Nano Mater. 2024;7(1):705–14. [Google Scholar]
33. Peng YF, Ashley T, Huang MH. Synthesis and thermal investigation of phosphate-functionalized acrylic materials. Poly J. 2018;50:967–74. [Google Scholar]
34. Ahn J, Chung WJ, Pinnau I, Song JS, Du NY, Robertson GP, et al. Gas transport behavior of mixed-matrix membranes composed of silica nanoparticles in a polymer of intrinsic microporosity (PIM-1). J Membr Sci. 2010;346(2):280–7. [Google Scholar]
35. Dos Santos JF, Couceiro R, Concheiro A, Torres-Labandeira JJ, Cet AL. Poly(hydroxyethyl methacrylate-co-methacrylated-β-cyclodextrin) hydrogels: synthesis, cytocompatibility, mechanical properties and drug loading/release properties. Acta Biomater. 2008;4(3):745–55. [Google Scholar] [PubMed]
36. Zare M, Bigham A, Luo H, Luo HR, Ghomi ER, Ramakrishna S. pHEMA: an overview for biomedical applications. Int J Mol Sci. 2021;22(12):6376. [Google Scholar] [PubMed]
37. Tran NPD, Yang MC. Synthesis and characterization of soft contact lens based on the combination of silicone nanoparticles with hydrophobic and hydrophilic monomers. J Polym Res. 2019;26(6):143. doi:10.1007/s10965-019-1813-6. [Google Scholar] [CrossRef]
38. Tran NP, Yang MC. Synthesis and characterization of silicone contact lenses based on TRIS-DMA-NVP-HEMA hydrogels. Polymers. 2019;11(6):944. doi:10.3390/polym11060944. [Google Scholar] [PubMed] [CrossRef]
39. Ouyang T, Su SX, Deng HT, Liu YY, Cui LS, Rong JH, et al. Superhydrophilic poly(2-hydroxyethyl methacrylate) hydrogel with nanosilica covalent coating: a promising contact lens material for resisting tear protein deposition and bacterial Adhesion. ACS Biomater Sci Eng. 2023;9(10):5653–65. doi:10.1021/acsbiomaterials.3c00856. [Google Scholar] [PubMed] [CrossRef]
40. García-Millán ES, Koprivnik S, Otero-Espinar FJ. Drug loading optimization and extended drug delivery of corticoids from pHEMA based soft contact lenses hydrogels via chemical and microstructural modifications. Int J Pharm. 2015;487(1–2):260–9. doi:10.1016/j.ijpharm.2015.04.037. [Google Scholar] [PubMed] [CrossRef]
41. Li RC, Guan XP, Lin XL, Guan PY, Zhang X, Rao ZQ, et al. Poly(2-hydroxyethyl methacrylate)/β-cyclodextrin-hyaluronan contact lens with tear protein adsorption resistance and sustained drug delivery for ophthalmic diseases. Acta Biomater. 2020;110:105–18. doi:10.1016/j.actbio.2020.04.002. [Google Scholar] [PubMed] [CrossRef]
42. Tran NP, Ting CC, Lin CH, Yang MC. A novel approach to increase the oxygen permeability of soft contact lenses by incorporating silica sol. Polymers. 2020;12(9):2087. doi:10.3390/polym12092087. [Google Scholar] [PubMed] [CrossRef]
43. Wang Z, Li XH, Zhang XJ, Sheng RL, Lin Q, Song WL, et al. Novel contact lenses embedded with drug-loaded zwitterionic nanogels for extended ophthalmic drug delivery. Nanomaterials. 2021;11(9):2328. doi:10.3390/nano11092328. [Google Scholar] [PubMed] [CrossRef]
Cite This Article
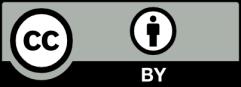
This work is licensed under a Creative Commons Attribution 4.0 International License , which permits unrestricted use, distribution, and reproduction in any medium, provided the original work is properly cited.