Open Access
ARTICLE
Synthesis and Characterization of Chitosan/Guar Protein Blended New Membrane with Enhanced Properties for Potential Applications
Tarsadia Institute of Chemical Science, Uka Tarsadia University, Surat, Gujarat, 394350, India
* Corresponding Author: Dharmesh R. Chejara. Email:
Journal of Polymer Materials 2024, 41(3), 179-190. https://doi.org/10.32604/jpm.2024.055887
Received 09 July 2024; Accepted 02 September 2024; Issue published 30 September 2024
Abstract
A chitosan and guar protein isolate blend conjugate (Ch/GPI-Glu) based membrane has been designed and studied for its possible interaction with this biopolymer mixture. The designed conjugate chitosan/guar protein isolate blended membrane was investigated for its structural features using Fourier Transform Infrared Spectroscopy (FTIR), Thermal Gravimetric Analysis (TGA), X-Ray Diffraction (XRD) and Scanning Electron Microscopy (SEM) analyses. IR Spectroscopic data suggested that chitosan and guar protein isolate contributed to a specific intermolecular interaction. The designed membrane was water-insoluble and it exhibited strong polymer–protein interaction. Further, the membrane was also found to have a smooth morphology which was studied from the SEM morphological analysis. Thermo-gravimetric analysis suggested the enhanced thermal behavior of the membrane. This approach of introducing guar protein to chitosan structure imparts hydrophobicity with enhanced mechanical strength. Designing chitosan-based materials using such proteins opens a new field of research for researchers working in macromolecular chemistry. Further, this study also value-adds the structural features of guar protein isolate to study its possible interaction with other polysaccharides to get materials with tailor-made applications.Keywords
Chitosan, a linear polysaccharide obtained from the deacetylation of chitin, is the second most famous biopolymer after cellulose [1]. Chitin can be found in nature as organized crystalline microfibrils that form structural elements in the cell walls of fungi and yeast as well as the exoskeleton of arthropods. Numerous other living things in the lower plant and animal kingdoms also manufacture it, and it is used for a variety of purposes when strength and reinforcement are needed. Even though chitin is found widely, crab and shrimp shells have been the primary commercial sources of chitin up until this point. Chitin is named chitosan when its degree of deacetylation reaches roughly 50% (depending on the polymer’s place of origin). At this point, the polymer is soluble in aqueous acidic environments. Protonation of the -NH2 function on the C-2 position of the d-glucosamine repeat unit causes the solubilization [2].
Natural polymer derived from the exoskeletons of crustaceans, chitosan is a deacetylated derivative of chitin. Chitosan, which is made up of glucosamine and N-acetylglucosamine units, is remarkably biocompatible, biodegradable, and non-toxic. Its medicinal uses, such as medication administration, wound healing, and tissue engineering, greatly benefit from these qualities. Chitosan’s usefulness in controlled release systems is increased by its capacity to form gels, films, and nanoparticles. Its ability to chelate metal ions and antibacterial activity also increase its application in food preservation, agriculture, and water treatment [3]. Chitosan-based materials in terms of composites, blends, and nanoformulations have been reported for their several applications and biological activity such as nontoxicity, biocompatibility, biodegradability, antimicrobial activity, and anticancer activity [4,5]. Chitosan has become a valuable candidate because of its applications in miscellaneous research, i.e., Food packaging, cosmetics, medicine, wastewater treatment adsorption, etc. Besides, chemical modification, physical blending, and crosslinking through reactive functional groups of chitosan are also employed to get some tailor-made applications of this biopolymer [6–8]. The cationic nature of chitosan permits ionic interactions with other ionic compounds, resulting in new materials. Chitosan is known to be a film-forming polymer possessing diverse properties such as mechanical, barrier, and antimicrobial properties [9,10]. The characteristics of chitosan-based film differ based on its source, solvents utilized, the methods incorporated for film preparation, the drying settings, also the amounts and types of plasticizers and/or copolymers implied [11]. Some studies have reported that films synthesized from mixtures appear to have improved their physicochemical properties, but further studies are yet to be done.
In recent times, chitosan-based blends and cross-linked derivatives with other biopolymers have been the point of focus for a growing number of studies reporting their impending usage in new materials [12]. The blending of these biopolymers generates tremendous opportunities to develop materials with enhanced properties. Blending with synthetic polymers imparts adjustable mechanical properties with optimum biocompatibility and biodegradability [13], compared to parent polysaccharides that frequently suffer from poor mechanical properties but good biocompatible and biodegradability. The blending of chitosan with blocks of proteins results in the formation of various interactions like hydrogen bonding, and hydrophobic connections that imparts novel properties to the chitosan. Proteins have poor mechanical properties and are susceptible to degradation due to proteolysis. Blending proteins with polysaccharides such as chitosan assists in improving in-vivo stability, thermal stability, and mechanical properties of proteins.
Several reports are there wherein, Collagen has been proven to be a potent candidate literature suggests [14], that chitosan-collagen-based blends are recommended for several medical applications such as regeneration of bone, cartilage repairs, etc., because of excellent cell adhesion, cell proliferation, and differentiation. Many chitosan/protein-based blends and crosslinked conjugates are reported for several applications. Protein-polysaccharide interaction augments physicochemical properties in native biopolymers [15]. The interaction of collagen, carrageenan, pea protein albumin, etc. with polysaccharides has been extensively studied. However, guar protein isolate contains similar peptides/amino acid contents when compared to other vegetable proteins, i.e., pea protein, and soya protein. Looking at the structural features of guar protein, there are no studies reported on its chemistry with polysaccharides so it was decided to use protein isolated from the new source, i.e., guar. The study on the interaction between guar protein and chitosan opened a new topic for researchers working on macromolecular chemistry and allows us to study further guar protein interaction with other polysaccharides to get materials with new applications. Guar protein isolate, extracted from a guar meal is the main by-product of guar gum production. It is a mixture of germs and hulls at a specific ratio of germs to hulls [16]. Guar meal is a protein-abundant material possessing about 50% protein, it is being used as a feed ingredient but may have to undergo processing to enhance palatability and eliminate antinutritional factors. Guar meal is considered to be an important protein supplement with good amino acid profiling; accordingly, it contains all the essential amino acids. Adding to it, relatively to a lesser extent research has been done in regard to guar protein. With this in view, Guar protein isolate was used as one of the new proteins to be used and see the possible interaction with chitosan and to get the resulted blend with possible applications.
Defatting of guar meal: Guar meal was extracted with hexane and dried in a current of air (25°C) to obtain the defatted guar meal.
Detoxifying of guar meal: The defatted guar meal was further processed to obtain the detoxified meal as detailed in the following. (a) Defatted guar meal were extracted using 80% isopropanol and dried in an oven overnight. (b) Extraction of the defatted guar meal with 1N HCl was carried out by steeping the guar meal in the acid for 30 min. The acid extract was decanted, and the residue was washed free of acid using tap water and testing the pH of the washings. The washed meal was dried in an oven. 0.25N HCl extraction of defatted guar meal was carried out at 60°C for 4 h and the residue was washed free of acid and dried as above. (c) The defatted guar meal was autoclaved at 1 kg/cm2 steam pressure (120°C) for 20 min and dried in an oven.
Guar protein isolation: The guar protein isolate extraction was carried from the detoxified and defatted guar meals by alkali extraction followed by isoelectric precipitation [17]. For the peptization, the guar meal was thoroughly suspended in 1N NaOH, and the suspension was stirred for 45 min. The suspension had a pH of 10. The suspension was centrifuged at 4000 RPM using a centrifuge (Neuation iFuge UC02R, Accumax Lab Devices Pvt. Ltd., Gandhinagar, Gujarat, India) to remove the residue, and the protein was precipitated at the isoelectric point (pH 4.5) by adjusting the pH of the centrifuged liquor with 1 N HC1. The neutralized proteinate was dried at ambient temperature and ground to pass through 0.355 mm mesh sieves. making it ready for utilization in the film-forming process.
2.2 Extraction of Guar Protein Isolate (GPI)
Defatting of guar meal: Guar meal were extracted with hexane and dried in a current of air (25°C) to obtain the defatted guar meal.
Detoxifying of guar meal: The defatted guar meal was further processed to obtain the detoxified meal as detailed in the following. (a) Defatted guar meal were extracted using 80% isopropanol and dried in an oven overnight. (b) Extraction of the defatted guar meal with 1N HCl was carried out by steeping the guar meal in the acid for 30 min. The acid extract was decanted, the residue washed free of acid using tap water and testing the pH of the washings. The washed meal was dried in an oven. 0.25N HCl extraction of defatted guar meal was carried out at 60°C for 4 h and the residue washed free of acid and dried as above. (c) The defatted guar meal were autoclaved at 1 kg/cm2 steam pressure (120°C) for 20 min and dried in an oven.
Guar protein isolation: The guar protein isolate extraction was carried from the detoxified and defatted guar meals by alkali extraction followed by isoelectric precipitation [17]. For the peptization the guar meal was thoroughly suspended into 1N NaOH, and the suspension was stirred for 45 min. The suspension had a pH of 10. The suspension was centrifuged at 4000 RPM using centrifuge (Neuation iFuge UC02R, Accumax Lab Devices Pvt. Ltd., Gandhinagar, Gujarat, India) to remove the residue, and the protein was precipitated at the isoelectric point (pH 4.5) by adjusting the pH of the centrifuged liquor with 1 N HC1. The neutralized proteinate was dried at ambient temperature and ground to pass through 0.355 mm mesh sieves. making it ready for utilization in the film forming process.
2.3 Characterization of the Guar Protein Isolate (Nitrogen estimation)
The obtained guar protein isolate was characterized using the Kjeldahl method [18,19]. The Kjeldahl method involves three main steps: Digestion: The sample is digested in concentrated sulfuric acid, converting nitrogen into ammonium sulfate. Distillation: The ammonium sulfate is then decomposed by adding a strong base (usually sodium hydroxide), releasing ammonia. Titration: The released ammonia is distilled and quantified by titration with a standard acid.
2.4 Crosslinking of Chitosan/Guar Protein Isolate Membrane (Ch/GPI-Glu)
The conjugate was prepared by dissolving 1.5–3 g of Chitosan (Ch) and 0.25–1 g of Guar protein isolate (GPI) into 100 mL of 10% aqueous acetic acid and 100 mL distilled water, respectively [20]. Separate clear solutions were prepared of the above-mentioned Ch and GPI and mixed to obtain a resulting solution. Later 1 mL of Glutaraldehyde (Glu) was added as a crosslinker to the film-forming solution, 1–1.6 mL of glycerol was added as a plasticizer. The above-mentioned film forming solution was prepared with stirring at RT at 1000 RPM. Finally, the film-forming solutions were spread on a glass plate and dried in the oven at 80°C.
2.5 Physico-Chemical/Mechanical Characterization
A detailed assessment was performed keeping in scope the visual and physical observations with an anticipation to use only membrane that were of uniform morphology, without ruptures or flimsy areas, with a smooth surface and with good flexibility. Films not possessing the aforementioned were discarded.
The above is plausible schematic representation (Fig. 1) of the interaction taking place between chitosan and guar protein isolate formed due to the native property of glutaraldehyde which is the designated crosslinker in the synthesis of the Ch/GPI-Glu conjugate [21–23].
Figure 1: Schematic representation of crosslinking chemistry
The moisture content of the membrane was calculated by using the following procedure [24]: The obtained membrane was placed in a glass plate and put into a circulating oven. The membrane was heated at 105°C for 1 h. The resultant material was then weight and calculated to obtain the moisture content percentage of the resultant membrane.
Film thickness was measured with a micrometer (ID-C112XBS micrometer, Mitutoyo Corporation, Tokyo, Japan) with a sensitivity of 0.001 mm. Results were reported as the average of at least five random locations for each film [25].
The swelling time is a very important parameter for determining the durability of films in an aqueous medium. The procedure [26] was performed by measuring the initial mass of the film and immersing it in distilled water at room temperature (25 ± 1°C) with low agitation. At pre-defined intervals, the film was removed, gently compressed on filter paper to remove excess surface water, weighed, and then returned to the aqueous medium. The total swelling time was determined when the mass of the film became constant. The degree of swelling was calculated as a function of the total initial mass.
The folding endurance was established by continuous folding of the membrane consistently at a fixed area until the membrane split. The obtained integer of multiples of the membrane folded without splitting is designated as the folding endurance value. The mean of an experiment conducted in triplicate performed on pieces membrane taken from various parts of the synthesized material was calculated and recorded as the conclusive folding endurance value [27].
2.6 Fourier Transform Infrared Spectroscopy (FT-IR)
FT-IR spectra’s of the membrane were analysed (Bruker Alpha II ATR FT-IR spectrometer, Bruker Scientific Pvt., Ltd., Billerica, MA, USA). Each film was placed on the sample stage of the ZnSe crystal of the ATR accessory and measured across a spectral range of 600–4000 cm−1, at an average of 23 scans was calculated for each sample.
2.7 Microstructure and Morphology
Surface morphology and microstructure of the film was studied using scanning electron microscopy (Hitachi-PU 10.0kV field emission scanning electron microscope, Hitachi High-Technologies Corporation, Tokyo, Japan). Vacuum oven dried samples were mounted on a sample holder and coated with gold via sputter coating (SPI Module™ Sputter Coater, SPI Supplies, West Chester, PA, USA) before analysis.
The thermal characteristics of the films and raw materials were evaluated with a TG-DT-DSC instrument (SDT Q600 V20.9 Build 20, TA Instruments, New Castle, DE, USA) using the ramp method. Film samples and raw material samples were placed into aluminium pans and then sealed, with a weight of ~7 mg. The samples were tested under a flowing air atmosphere at a heating rate of 10°C/min, with a temperature range of 30°C to 800°C.
2.9 X-Ray Diffraction Analysis
The powder X-ray diffraction properties were obtained using X-ray diffraction (Philips vertical scanning diffractometer type 42273, Philips Electronic Instrument, Mount Vernon, NY, USA). The samples were exposed to CuKh radiation under 35 kV and 20 mA under the 2-theta ranging from 5° to 90° at an increment of 0.5°. The diffraction profile for the prepared membrane was obtained. The membrane was ground into fine powder before analysis.
3.1 Physico-Chemical/Mechanical Characterization of Ch/GPI-Glu Membrane
The moisture content of the synthesized membrane was observed to be negligible (0.04%). Film thickness was recorded from five random cross-sections of the crosslinked membrane. The obtained results were reported as the average from the above-mentioned cross-sections to be 0.01 mm. The swelling study of the synthesized membrane was conducted for a total of 60 min to see any significant absorption of water. The membrane was weighed and recorded to be 0.021 g before being introduced into water. After 60 min the membrane was weighed again and recorded to be 0.023 g, which was considered to be negligible. Hence deducing that there was no water absorption found in the designed membrane. The reason the membrane does not absorb water was due to a higher amount of crosslinking and blending which introduced hydrophobicity to the chitosan backbone. The hydrophobic nature of the membrane led to the idea of the possibility of a hydrophobic film with certain changes to the methodology that can be more useful in sustaining wound healing, especially where the longer recovery time is applicable. Moreover, studies represent biomimetic chitosan/gelatin blend scaffolds for versatile tissue engineering [28–30] suggesting this membrane can be applied in such applications as well [31]. Visually the membrane was observed with no significant change in physical characteristics (Fig. 2).
Figure 2: Swelling study experimentation of designed membrane
The folding endurance was observed in range 40 to 50 folds. Results were recorded based on the experiment conducted in triplicate. The folding endurance when compared to the parent resulted to be enhanced in the designed membrane due to higher amount of crosslinking consequential in the dense network formation to the structural backbone.
3.2 FT-IR Spectral Analysis of Ch/GPI-Glu Membrane
FT-IR spectroscopic analysis was performed to evaluate the physical interaction between chitosan and guar protein isolate. Fig. 3 exhibited the FT-IR spectra of the Ch/GPI-Glu membrane along with both parent polymers. In spectrum of designed membrane (Ch/GPI-Glu) the dominant signal was observed at 3272 cm−1 that corresponding to O-H stretching and intermolecular/intramolecular hydrogen bonds in to the membrane. Two sharp bands at 2925 cm−1 are characteristic of C-H stretching (-CH2).
Figure 3: FTIR Spectra of (a) Ch/GPI-Glu (b) Guar protein isolate and (c) Chitosan cross-linked membrane
The band near 1153 cm−1 is believed to originate from the glycosidic linkages in polysaccharides. C-O and C-N stretches were also observed at near about 1530 cm−1, corresponding to the amide-2 band of the protein. However, the bending and stretching vibrational bands of amide-1 and amide-2 are important for the determination of secondary structure of protein [32]. The spectral bands near to the 1060 and 1030 cm−1 present in protein were due to C-N stretch, which suggests the presence of primary amine groups confirms the blending of protein moiety. Rest all bands of respective functional groups were observed in the spectrum of membrane which were in line with that of chitosan and guar protein isolate [33–35] confirmed the physical blending of these two compounds.
3.3 Microstructure and Morphology of Ch/GPI-Glu Membrane
Scanning electron microscopy (SEM) image analysis was carried out on the optimized Ch/GPI-Glu membrane. Uniform organized structure and regularity in the structures are believed to increase the homogeneity in the film that was seen in the SEM analysis of this membrane. The SEM micrographs of various magnifications have been recorded which distinctly exhibited a uniform layered surface with lower pores observed along the whole Ch/GPI-Glu membrane strip whose morphology was shown in the SEM image of the film (Fig. 4). The unsusceptible nature of swelling of the membrane is inferred to be due to the insignificant amount of pores and none-to-minute pore size observed on the morphology of the film. Due to this fashion of morphology of the membrane, the hydrophobicity of the membrane is implied.
Figure 4: SEM micrographs of Ch/GPI-Glu membrane at different magnifications
3.4 Thermal Analysis of Ch/GPI-Glu Membrane
In order to study the thermal characteristics of the designed membrane compared with parent biopolymers, which were conducted in a flowing air atmosphere. In thermo gravimetric analysis, the first stage of weight loss was observed around 100°C in the both parent polymers while there was gradual mass loss was observed in the Ch/GPI-Glu membrane starting from the experiment. The initial stage of weight loss was possibly due to the evaporation of loosely bound water and bound solvent as a result of condensation during the initial phase of heating which was not present in significant amount in membrane can be confirmed from moisture analysis data. Further a noticeable and sharp weight loss was observed around a range of 270°C to 300°C in TGA of both biopolymers, i.e., Chitosan and guar protein isolate as compared to the Ch/GPI-Glu membrane showed gradual decrement in mass loss at this stage. This stage of weight loss in the membrane as well as parent polymers was possibly due to the decomposition of a few units (Fig. 5).
Figure 5: Thermo gravimetric analyses of (a) Chitosan, (b) Guar protein isolate and (c) Ch/GPI-Glu cross-linked membrane
3.5 X-Ray Diffraction Study of Ch/GPI-Glu Membrane
The profile in Fig. 6 elucidates the X-ray diffraction patterns for the prepared membrane alongside chitosan. The stereoregularity or symmetry present throughout the polymeric chain is essential for a polymer to be crystalline [36]. The crystallinity of the membrane film was clearly exhibited by their distinctive X-ray diffraction patterns. The X-ray diffraction shows evident crystalline peaks at 2θ values 20° similar to that of chitosan that became weak in the blend and the peak at 20° weakened and the intensity of the peak became low. This evidence suggested that a strong interaction occurred between chitosan and guar protein isolate A similar pattern was observed which exhibited that the backbone of chitosan remained intact. The little shift in the diffraction peaks and the diffraction pattern with a broad amorphous type peak indicated that there was molecular miscibility and interaction between the components [37]. The XRD results thus, provided supporting evidence to the FTIR and TGA results that some specific interaction between these two biopolymers existed in the blend.
Figure 6: X-ray diffraction patterns of (a) Chitosan and (b) Ch/GPI-Glu cross-linked membrane
Physicochemical and morphological characterization of the chitosan/guar protein isolate-based blend has been designed in order to see the possible interactions and the applications of membranes containing different amounts of the two biopolymers.
The use of SEM technique for the morphology of the membrane blend exhibited increased hydrophobicity and roughness as both polymers were blended suggesting homogeneous blending between these polymers. The source and extent of the interaction between the polysaccharide and protein constituents of the blends was further studied using FTIR spectroscopy and X-ray diffraction patterns suggesting the most homogeneous membrane and possessing characteristics of both biopolymers. This approach of introducing guar protein to the chitosan backbone opens a new field of research to study further its interaction with other polysaccharides to get materials with newer applications.
Acknowledgement: I would like to acknowledge SHODH-Scheme of developing high quality research, Government of Gujarat for fellowship. Further I am thankful to Tarsadia Institute of Chemical Science and Uka Tarsadia University for providing the facility to carry out the research work, also Shivaji University, Kolhapur, Maharashtra for providing with the analysis assistance.
Funding Statement: The authors received no specific funding for this study.
Author Contributions: The authors confirm contribution to the paper as follows: study conception and design: Dharmesh R. Chejara; data collection: Anuraag Moturi; analysis and interpretation of results: Anuraag Moturi, Dharmesh R. Chejara; draft manuscript preparation: Anuraag Moturi, Dharmesh R. Chejara. All authors reviewed the results and approved the final version of the manuscript.
Availability of Data and Materials: Data will be made available on request.
Ethics Approval: Not applicable.
Conflicts of Interest: The authors declare that they have no conflicts of interest to report regarding the present study.
References
1. Kumar MNR. A review of chitin and chitosan applications. React Funct Polym. 2000;46(1):1–27. [Google Scholar]
2. Rao KM, Uthappa UT, Kim HJ, Han SS. Tissue adhesive, biocompatible, antioxidant, and antibacterial hydrogels based on tannic acid and fungal-derived carboxymethyl chitosan for wound-dressing applications. Gels. 2023;9(5):354. doi:10.3390/gels9050354. [Google Scholar] [PubMed] [CrossRef]
3. Atkar A, Sridhar S, Deshmukh S, Dinker A, Kishor K, Bajad G. Synthesis and characterization of sulfonated chitosan (SCS)/sulfonated polyvinyl alcohol (SPVA) blend membrane for microbial fuel cell application. Mater Sci Eng B Solid State Mater Adv Technol. 2024;299:116942. [Google Scholar]
4. Davis G, Song JH. Biodegradable packaging based on raw materials from crops and their impact on waste management. Ind Crops Prod. 2006;23(2):147–61. [Google Scholar]
5. Díaz-Montes E, Castro-Muñoz R. Edible films and coatings as food-quality preservers: an overview. Foods. 2021;10(2):249. [Google Scholar]
6. Mihindukulasuriya S, Lim LT. Nanotechnology development in food packaging: a review. Trends Food Sci Technol. 2014;40(2):149–67. [Google Scholar]
7. Otoni CG, Avena-Bustillos RJ, Azeredo HM, Lorevice MV, Moura MR, Mattoso LH, et al. Recent advances on edible films based on fruits and vegetables—a review. Compr Rev Food Sci Food Saf. 2017 Sep;16(5):1151–69. doi:10.1111/1541-4337.12281. [Google Scholar] [PubMed] [CrossRef]
8. Vodnar DC, Pop OL, Dulf FV, Socaciu C. Antimicrobial efficiency of edible films in food industry. Not Bot Horti Agrobo. 2015 Dec 10;43(2):302–12. doi:10.15835/nbha43210048. [Google Scholar] [CrossRef]
9. Coma V, Martial-Gros A, Garreau S, Copinet A, Salin F, Deschamps A. Edible antimicrobial films based on chitosan matrix. J Food Sci. 2002;67(3):1162–9. doi:10.1111/j.1365-2621.2002.tb09470.x. [Google Scholar] [CrossRef]
10. Sebti I, Chollet E, Degraeve P, Noel C, Peyrol E. Water sensitivity, antimicrobial, and physicochemical analyses of edible films based on HPMC and/or chitosan. J Agric Food Chem. 2007 Feb 7;55(3):693–9. doi:10.1021/jf062013n. [Google Scholar] [PubMed] [CrossRef]
11. Cervera MF, Heinämäki J, Krogars K, Jörgensen AC, Karjalainen M, Colarte AI, et al. Solid-state and mechanical properties of aqueous chitosan-amylose starch films plasticized with polyols. AAPS PharmSciTech. 2004 Mar;5(1):109–14. doi:10.1007/BF02830583. [Google Scholar] [CrossRef]
12. Zhao Y, Zhang Y, Dong H, Wu W, Yang X, He Q. Functional biopolymers for food packaging: formation mechanism and performance improvement of chitosan-based composites. Food Biosci. 2023 Jul 14;54:102927. [Google Scholar]
13. Terzopoulou Z, Zamboulis A, Koumentakou I, Michailidou G, Noordam MJ, Bikiaris DN. Biocompatible synthetic polymers for tissue engineering purposes. Biomacromolecules. 2022 Apr 19;23(5):1841–63. [Google Scholar]
14. Silva CC, Lima CG, Pinheiro AG, Goes JC, Figueiro SD, Sombra AS. On the piezoelectricity of collagen-chitosan films. Phys Chem Chem Phys. 2001;3(18):4154–7. [Google Scholar]
15. Das A, Das A, Basu A, Datta P, Gupta M, Mukherjee A. Newer guar gum ester/chicken feather keratin interact films for tissue engineering. Int J Biol Macromol. 2021 Jun 1;180:339–54. [Google Scholar]
16. Lee JT, Connor-Appleton S, Haq AU, Bailey CA, Cartwright AL. Quantitative measurement of negligible trypsin inhibitor activity and nutrient analysis of guar meal fractions. J Agric Food Chem. 2004 Oct 20;52(21):6492–5. [Google Scholar]
17. Tasneem R, Ramamani S, Subramanian N. Functional properties of guar seed (Cyamopsis tetragonoloba) meal detoxified by different methods. J Food Sci. 1982 Jul;47(4):1323–8. doi:10.1111/j.1365-2621.1982.tb07678.x. [Google Scholar] [CrossRef]
18. Anderson RL. Ultracentrifugation and binding studies of acid-sensitive soybean proteins. Cereal Chem. 1974;51(6):707–17. [Google Scholar]
19. Wolf WJ. Physical and chemical properties of soybean proteins. J Am Oil Chemists’ Soc. 1977;54(2):A112–7. doi:10.1007/BF02912385. [Google Scholar] [PubMed] [CrossRef]
20. Mi FL, Shyu SS, Wu YB, Lee ST, Shyong JY, Huang RN. Fabrication and characterization of a sponge-like asymmetric chitosan membrane as a wound dressing. Biomaterials. 2001 Jan 15;22(2):165–73. doi:10.1016/S0142-9612(00)00167-8. [Google Scholar] [PubMed] [CrossRef]
21. Wahba MI. Glutaraldehyde-pea protein grafted polysaccharide matrices for functioning as covalent immobilizers. Sci Rep. 2023 Jun 5;13(1):9105. doi:10.1038/s41598-023-36045-z. [Google Scholar] [PubMed] [CrossRef]
22. Li Z, Lin Z. Recent advances in polysaccharide-based hydrogels for synthesis and applications. Aggregate. 2021;2(2):e21. doi:10.1002/agt2.21. [Google Scholar] [CrossRef]
23. Francolini I, Galantini L, Rea F, Di Cosimo C, Di Cosimo P. Polymeric wet-strength agents in the paper industry: an overview of mechanisms and current challenges. Int J Mol Sci. 2023 May 25;24(11):9268. [Google Scholar]
24. Hu F, Sun T, Xie J, Xue B, Li X, Gan J, et al. Functional properties of chitosan films with conjugated or incorporated salicylic acid. J Mol Struct. 2021 Jan 5;1223:129237. [Google Scholar]
25. Siripatrawan U, Harte BR. Physical properties and antioxidant activity of an active film from chitosan incorporated with green tea extract. Food hydrocolloids. 2010 Nov 1;24(8):770–5. [Google Scholar]
26. Abdeen Z. Swelling and reswelling characteristics of cross-linked poly(vinyl alcohol)/chitosan hydrogel film. J Dispers Sci Technol. 2011;32(9):1337–44. [Google Scholar]
27. Gilhotra R, Mishra D. Alginate-chitosan film for ocular drug delivery: effect of surface cross-linking on film properties and characterization. Die Pharmazie-An Int J Pharm Sci. 2008;63(8):576–9. [Google Scholar]
28. Bakopoulou A, Georgopoulou Α, Grivas I, Bekiari C, Prymak O, Loza K, et al. Dental pulp stem cells in chitosan/gelatin scaffolds for enhanced orofacial bone regeneration. Dent Mater. 2019 Feb 1;35(2):310–27. doi:10.1016/j.dental.2018.11.025. [Google Scholar] [PubMed] [CrossRef]
29. Kumari S, Singh BN, Srivastava P. Effect of copper nanoparticles on physico-chemical properties of chitosan and gelatin-based scaffold developed for skin tissue engineering application. 3 Biotech. 2019 Mar;9(3):102. [Google Scholar] [PubMed]
30. Liu Y, Lv Y, An M, Li F, Lu Y, Song J. Characterization of chitosan-gelatin blend scaffolds. J Macromol Sci B. 2019 Jul 3;58(7):634–44. doi:10.1080/00222348.2019.1615688. [Google Scholar] [CrossRef]
31. Xu W, Wang Z, Liu Y, Wang L, Jiang Z, Li T, et al. Carboxymethyl chitosan/gelatin/hyaluronic acid blended-membranes as epithelia transplanting scaffold for corneal wound healing. Carbohyd Polym. 2018 Jul 15;192(2016):240–50. doi:10.1016/j.carbpol.2018.03.033. [Google Scholar] [PubMed] [CrossRef]
32. Trigui I, Zarai Z, Chevance S, Cheikh-Rouhou S, Attia H, Ayadi MA. Physicochemical properties, antioxidant activity and in vitro gastrointestinal digestion of purified proteins from black cumin seeds. Int J Biol Macromol. 2019 Apr 1;126:454–65. doi:10.1016/j.ijbiomac.2018.12.198. [Google Scholar] [PubMed] [CrossRef]
33. Pawlak A, Mucha M. Thermogravimetric and FTIR studies of chitosan blends. Thermochim Acta. 2003 Feb 5;396(1–2):153–66. doi:10.1016/S0040-6031(02)00523-3. [Google Scholar] [CrossRef]
34. Zheng H, Du Y, Yu J, Huang R, Zhang L. Preparation and characterization of chitosan/poly(vinyl alcohol) blend fibers. J Appl Polym Sci. 2001 Jun 24;80(13):2558–65. doi:10.1002/app.1365. [Google Scholar] [CrossRef]
35. Kweon H, Um IC, Park YH. Structural and thermal characteristics of Antheraea pernyi silk fibroin/chitosan blend film. Polymer. 2001 Jul 1;42(15):6651–6. doi:10.1016/S0032-3861(01)00104-5. [Google Scholar] [CrossRef]
36. McCrum NG, Buckley CP, Bucknall CB. Principles of polymer engineering. Oxford, UK: Oxford University Press; 1997. [Google Scholar]
37. Zhang QP, Xia WS. Physico chemical properties of chitosan based films. Food Technol Biotechnol. 2008;46(3):262–9. [Google Scholar]
Cite This Article
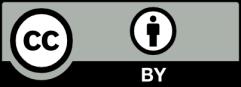
This work is licensed under a Creative Commons Attribution 4.0 International License , which permits unrestricted use, distribution, and reproduction in any medium, provided the original work is properly cited.