Open Access
ARTICLE
Study of Galvanic Charging-Discharging Properties of Graphene Nanoplatelets Incorporated Epoxy-Carbon Fabric Composites
1
Department of Electronics and Communication Engineering, Kalpataru Institute of
Technology, Tiptur, Tumkuru, Karnataka, India.
2
NITTE (Deemed to be University), Department of Electronics and Communication
Engineering, NMAM Institute of Technology, Nitte-574110, Karnataka, India.
3 Department of Electronics and Communication Engineering, The National Institute of
Engineering, Mysuru, Karnataka, India.
* Corresponding Author: e-mail:
Journal of Polymer Materials 2023, 40(1-2), 93-103. https://doi.org/10.32381/JPM.2023.40.1-2.8
Abstract
Polymer composites are increasing in demand in energy storage applications including in the electronic as well as electrical industries due to the ease of processing of these materials with associated advantages like light weight, corrosion resistance, and high mechanical strength. In this investigation, efforts are made to enhance the charging and discharging properties of epoxy/carbon fabric composite by the addition of graphene nanoplatelets (GNPs) into the epoxy/ carbon matrix. The performance of the composites with graphene platelets of 0.5 to 5 wt. % in epoxy were characterized and 1wt.% percolation threshold was observed poor performance in gravimetric charge and discharge characteristics were observed. Based on the percolation threshold Epoxy-Carbon fabric (EC) composites and Epoxy-Carbon fabric with 1 wt. % of GNP (ECG1) composites were fabricated using a vacuum-assisted resin transfer molding technique. The electrochemical performance was studied using the Cyclovoltammetry test and 0.1 M of Na2SO4 as a supporting electrolyte. The Galvanostatic Charge-Discharge characteristics were carried-out for EC and ECG1 at a scan rate of 80 sec, 300 sec, 4000 sec with 2 runs and 8 runs. The composite EC didn’t not show GCD characteristics, but the symmetry of charge-discharge characteristics observed for ECG1 and same are discussed in this paper. These highly effective, and excellent characteristics demonstrate that Epoxy-GNP-CF composites may be promising composites for practical energy storage applications.Keywords
Fiber-reinforced polymer composite materials are used in aerospace, marine and other transportation applications because of their superior specific strength, stiffness, resistance to corrosion, and fatigue and ease of manufacturing[1]. Graphene is the preferred material for the development of electrodes in electrochemical sensor applications because of its outstanding electrical, mechanical, and thermal characteristics in its platelet form, graphene has been widely used as filler for increasing the electrical conductivity and thermal properties of polymer composites. Epoxy-graphene composites as an advanced material are well established in automobile and aerospace applications[2].
With the growing demands for more efficient energy storage systems, batteries have been used for supplying power for most applications for the past long time. Supercapacitors (SCs), with high power density, fast charge/discharge rate, long-cycle life and environmentally friendly operation, hold great potential to compensate for the shortcomings of batteries and offer additional flexibility for the storage of electricity[3]. As a type of electrochemical energy storage device, supercapacitors have attracted considerable attention over the past decades. Owing to the higher power density and longer cycle life than secondary batteries and higher energy density compared to conventional capacitors, they are applicable in some systems such as hybrid electric vehicles, portable electronics, mobile communications, etc., and are believed to have the potential to fill the power/energy gap between traditional dielectric capacitors and batteries/fuel cells. The charge/discharge tests were also carried out at Galvanostatic Charge-Discharge (GCD) current densities. During the process of charge/discharge, the electrochemical redox reaction between the electrode and electrolyte could be illustrated[4].
Graphene was originally defined as a 2-dimensional (2D) monolayer sheet of sp2 - bonded carbon. These materials include double and few-layer graphene and chemically reduced graphene oxide [reduced graphene oxide (r-GO)][5]. It was reported that graphene or reduced graphene oxide (r-GO) nanocomposites can obtain a high dielectric constant at extremely low graphene loading[6].
From the literature, it is evident that epoxy composites with different conducting fillers have been widely explored for electrochemical sensing. Several materials have been used to construct electrochemical composite biosensor. Among them, carbon-based matrices are the most applied due to costs and their electronic conductivity[7]. In comparison with conventional polymeric materials, conducting polymer nanocomposites have garnered increasing interests due to their improved electrochemical behavior, facile synthesis, biocompatibility, large surface area, high electrical conductivity, elevated carrier transport, and unique optical behaviors[8]. Natural conducting polymers have low conductivity and are semi conductive. Therefore, it is essential to increase their conductivity, mainly for use in electrochemical sensor programs[9]. However, the use of aerospace-grade epoxy with carbon fabric reinforcement and graphene nanoplatelets has not been investigated for electrochemical sensing applications. Hence, in this investigation graphene nanoplatelets (GNPs) incorporated epoxy/carbon fabric composites are made and studied for their charging and discharging properties.
The resin Epon 828 and the curing agent Epicure W were supplied by Miller Stephenson Inc, USA. Exfoliated graphene fillers (GNP) in platelet form with an average thickness of 8 nm, a surface area of 140 m2/g and an average diameter of 25 µm were received from XG Sciences Inc., USA. Carbon fabric (3K-AS4-8HS) with an areal density of 372 g/m2, and a bulk density of 1.79 g/cm3 was received from Northern Composites, USA and was used as reinforcement to the epoxy resin.
2.2 Method of Fabrication of Composites
Composites with the reinforcement of carbon fabric were engineered by the hand-layup method with bagging followed by compression moldings, with and without GNP of 1 wt. %. The resin and the hardener were mixed mechanically using 500±20 RPM for 15±2 m and then subjected to vacuum at 60±2° C for 30±2 m. In the case of nanocomposites, 1 wt. % of GNP was first dispersed in the polymer matrix using the three-roll mill method[1,2]. The particulars of fabricated composites and their identification for discussions of results are shown in Table 1.
The cross-sectional morphological characteristics of the composites were carried out using the field emission scanning electron microscope (FESEM) model ULTRA 55-FESEM (Carl Zeiss).
The cyclic voltammetry (CV) investigations and sensing of thiourea in seawater at room temperature, Biologic SA multichannel potentiostat/galvanostat model VMP3 in the potential range of -0.8 to 1 V was used. For the heat dissipation analysis, model Synrad 48 was used. The device employed a tunable CO2 laser. The diameter of the beam was 3.5 mm and the measurements were carried out at a wavelength of 10.1µm. The power capability of the apparatus was 80 kW/m2. For imaging, the heat distribution on the surface infra-red camera FLIR SC5200 was used. It operated at a frame speed of 500 Hz was used. Assessment of the Heat dissipation capability of the composites was made under ambient conditions. The initial temperature of the specimens was 80°C and the rate of cooling were determined up to 30°C. It was also possible to map the temperature distribution of the surface.
3.1 Morphology of Composites using SEM
The SEM images of the composites are shown in Figure 1. To understand the interfacial interactions occurring between GNP, carbon fabric and the epoxy in the composites, SEM morphological study was undertaken. The cross-sectional morphology of the epoxy-GNP composites reveal that the epoxy structure is homogeneous and the incorporation of GNP particles lead to random distribution within the epoxy matrix.
Fig. 1: SEM images of the composites.
The SEM image of EC shows that the surfaces of the carbon fibers of the fabric are smooth and the individual fibers are visible indicating that the fibers are distinctly visible without being merged with the base epoxy, this fact demonstrates the poor adhesion between the Carbon fabric and the epoxy matrix. The cross-sectional morphology of ECG1 composite highlights the fact that 1 wt. % of GNP results in good reinforcement due to the binding provided by GNP to the epoxy and the carbon fabric[1, 2].
3.2 Galvanostatic Charge-Discharge (GCD)
Galvanostatic charge and discharge studies were carried out to investigate the performance and life cycle of the electric double-layer capacitors with ECG1 composite which showed good performance as an electrode material. The Galvanostatic charge/discharge studies were carried out using 1 mole (M) Na2SO4 as the electrolyte at constant current densities of 1mA/cm2. Carbon-based supercapacitors always show symmetrical triangular responses as a function of the time of the charge and discharge processes. It demonstrates the existence of an ideal supercapacitor dielectric property. Sometimes, there will be a change in the regular profile at the starting point of the discharge curve due to the IR drop and this is due to the resistance of the electrode material. The GCD characteristic of ECG1 is shown in Figure 2 for 80 sec.
Fig. 2: Galvanostatic Charge-Discharge characteristic of ECG1 showing the symmetry of charge discharge characteristics over 80 sec.
ECG1 electrode material exhibits ideal symmetrical shaped GCD characteristics in comparison to the EC electrode material (not shown in graph). Good linear voltage-time profiles are clear signs of the excellent performance of the materials as dielectrics for supercapacitors. The good linear-voltage -time profiles are indications of the excellent super-capacitive nature of the electrode material. In Figure 3, the charge-discharge characteristics are shown for an extended period of 300 sec. The GCD for extended for two runs and duration of 104 sec is shown in Figure 4 and 5 respectively, to highlight the consistency of the charge-discharge processes. Some distortions are observed during 2800 sec and beyond. The GCD for eight runs is shown in Figure 6 and the distortions were minimal in this period.
Fig. 3: Galvanostatic Charge-Discharge characteristic of ECG1showing the symmetry of charge-discharge characteristics over 300 sec.
Fig. 4: Galvanostatic Charge-Discharge characteristic of ECG1 showing the symmetry of charge-discharge characteristics over 4000 sec.
Fig. 5: Galvanostatic Charge -Discharge characteristic of ECG1 showing the symmetry of charge-discharge characteristics over two runs.
Fig. 6: Galvanostatic Charge-Discharge characteristic of ECG1 showing the symmetry of charge-discharge characteristics over eight runs.
The electrode materials used to characterize the GCD tests must be an electrical conductor. Epoxy is a pure insulator, when the wt. % of GNP reaches 1 wt. %, there is significant increase in dc conductivity (σdc) of the composite. At this threshold, percolation wt. % phase transition occurs which alters Epon matrix into a conductive medium. The introduction of GNP significantly increases the σdc at 1 wt. % (percolation threshold)[10]. The increase in σdc occurs due to the proximity of the graphene nano platelets. Charge transfer mechanism is involved either directly or by overcoming the barrier of the chains of the Epon 828 matrix. The transport of electrons includes both hoping and tunneling through the nanoplatelets of graphene. The threshold for electrical percolation depends on the wt. % of the nanoparticle, uniformity of dispersion of the fillers its orientation, and typical geometrical characteristics of the filler and the mechanism of charge transport.
Based on this percolation threshold value, EC and ECG1 were fabricated and characterized for GCD. No results were obtained for EC but ECG1 shows GCD results and are discussed in this paper. ECG1 improves the conductivity and the reason for this is takes place in the two directions; one across and the other perpendicular to the fiber orientation are nearly the same supporting the view that the composites are isotropic with good dispersion of the graphene nanoplatelets and their interconnectivity leading to the establishment of a good conducting network addition of 1 wt. % of graphene enhance the conduction in all the direction in the material. Due to the enhanced electron cloud of graphene from the
A typical IR drop observed during GCD is shown in Figure 7. The Columbic efficiency of the electrode materials determined from charge-discharge characteristics was observed to be in the order of 92 to 95 % for the two materials studied, with ECG1 having higher Columbic efficiency.
Fig. 7: Galvanostatic charge and discharge curve showing the IR drop.
The specific capacitance was determined to be of the order of 100 to 300 F/g in an aqueous medium for the two composites investigated, with ECG1 having higher specific capacitance and 120 to 300 F/g using 1M of Na2SO4 solution. The value of Rs was estimated from the relation:
In this equation, VIR is the voltage change when the current changes from the charging configuration to discharging process. This factor is observed to vary from 10 to 15 Ω in EC to 3 to 5 Ω on average, based on several experiments carried out in the case of ECG1.
3.3 Heat Dissipation Capability
Since higher thermal conductivity is achieved in composite EC and ECG1, an attempt was made to practically demonstrate the heat transfer capability of these composites. The composites were subject to direct heating in a convection chamber of 0.25 m3 to a pre-determined temperature of 130°C and the composite was then cooled under ambient conditions. The variations in the temperature of the composite as a function of time were recorded up to a period in which the composite reached the ambient temperature. The experiments were repeated using a LASER beam for heating the specimen. Even in this case, the time duration for fall in temperature from 80°C to ambient temperature was recorded.
The capability of the composites to dissipate the heat energy absorbed under ambient conditions is shown in terms of a decrease in temperature with time under RH of 55±5 % and temperature of 25± 2° C is shown in Figure 8 for both heating’s of the sample with a laser. In the case of heating with a laser beam, as soon as the specimen temperature reached a value of 80° C, the laser source was switched off and the composite was cooled to ambient conditions. The results of Figure 8 show that the composite ECG1 attains equilibrium with the room temperature of 30° C in an average time duration of 70 sec. All five specimens were investigated and the duration was within 70±1 sec.
Fig. 8: Variation of temperature with time duration of composite EC and ECG1.
The corresponding surface temperature mapping of the composite EC is shown in Figure 9 and the composite takes a longer duration of 89 sec for cooling down to 30°C. From the heat map shown in Figure 9, it is observed that at the beginning of cooling on exposure to ambient conditions, more than 80 % of the surface area is at temperatures between 79 to 80° C and the rest of the surface is at a temperature ranging from 75 to 77°C. It is further observed that within 2.3 sec, the temperature in the central portion reduces from 78 to 79°C whereas, across 85 % of the surface area, the temperature is reduced to 74 to 76°C. After 3.9 sec the temperature in the central portion is reduced to 76° C and towards the periphery it reduces from 70 to 61°C.
Fig. 9: Surface temperature mapping of epoxy-carbon fabric composite at different durations from starting temperature of 80°C
The decrease in temperature is consistent and in 18.5 sec, the average temperature is reduced to about 50°C. Thus, the ability of the epoxy-carbon fabric composite due to the presence of GNP platelet results in the efficient transfer of heat to the surrounding. The corresponding results of the base composite it is observed that 1wt. % of GNP leads to a more than two-fold improvement in the heat transfer capability of the epoxy-carbon fabric composite since the thermal conductivity measurements of the base epoxy is observed to improve from 0.14W/m K to 0.7 and 0.72 W/mK in EC and ECG1. Thus, ECG1 can dissipate heat in an efficient manner which can be exploited for applications in device electronics. The larger surface area of the GNP and its uniform dispersion coupled with the connectivity it provides to the carbon fabric layers is responsible for faster thermal dissipation.
Thus, when the composite is used in a device as a heat sink, on the device heating up due to many reasons, it will take only 70 s for the temperature to be reduced to 30°C, which is a reasonably good dissipation rate. The rate of fall in temperature in EC is 0.62°C/s and in ECG1 it is 1.88°C/s, though the difference in thermal conductivity is 0.03 W/mK.
Some of the salient features of this investigation are the following:
1. The performance of the composites with graphene platelets of 0.5 to 5 wt. % in the epoxy matrix did not show satisfactory performance.
2. An 8-ply carbon fabric with the incorporation of 1 wt. % of graphene in the epoxy matrix was used to improve the electrochemical performance of the composite
3. Composites EC and ECG1 exhibit excellent CV response and ECG1 is relatively better among the two. The limit of detection observed was 0.012 mM at 5 mV/s for TU detection.
4. ECG1 is an excellent electrode material and even lower concentrations of TU has been detected and it possesses outstanding catalytic activity in terms of thiourea oxidation.
5. The galvanostatic charge and discharge characteristics have shown high consistency of the charge-discharge processes and the series resistance of the equivalent circuit was estimated to be in the range of 3 to 5Ω.
6. The heat dissipation capability of ECG1 attains equilibrium at the room temperature of 30°C in an average time duration of 70 sec.
References
1. B. P. Chang, A. K. Mohanty and M. Misra. (2020). RSC adv. 10: 17955. [Google Scholar]
1. Kazi A Imran and Kunigal N Shivakumar. (2017). J. Reinforced Plastics and Com. pp. 1–16. [Google Scholar]
2. Hadimani Shivakumar, N. M. Renukappa, Kunigal Shivakumar, and J Sundara Rajan. (2020). IEEE Sens. J. 20: 21. [Google Scholar]
3. Storage Fei Shen, Dmitry Pankratov and Qijin Chi. (2017). Current Opinion in Electrochem. 17: pp. 1–27. [Google Scholar]
4. Haihan Zhou, Gaoyi Han, Yaoming Xiao, Yunzhen Chang, Hua-Jin Zhai. (2014). J. Power Sources 263: 259–267. [Google Scholar]
5. Fei Liu, Chul Wee Lee, and Ji Sun Im. (2013). J. Nanomater. 642915: 1–11. [Google Scholar]
6. Kai Wu, Chuxin Lei, Weixing Yang, Songgang Chai, Feng Chen and Qiang Fu. (2016). Comp. Sci. Tech. 16: 1–26. [Google Scholar]
7. Eliana and N. Bojorge. (2011). InTech (Metal, Ceramic and Polymeric Composites for Various Uses597–620. [Google Scholar]
8. Christopher Igwe Idumah. (2021). Synthetic Metals, 273: 116674. [Google Scholar]
9. Sara A. Alqarni, Mahmoud A. Hussein, Aisha A. Ganash and Anish Khan. (2020). BioNano Science, 1–14. [Google Scholar]
10. Chao Zheng, Zhuangjun Fan, Tong Wei and Guilian Luo. (2009). J. Applied Polymer Science, 113: 1515–1519. [Google Scholar]
11. Mitra Yoonessi, James R. Gaier, Muhammad Sahimi, Tyrone L. Daulton, Richard B. Kaner and Michael A. Meador. (2017). ACS Appl. Mater. Interfaces, 9: 43230–43238. [Google Scholar]
Cite This Article
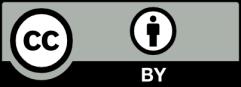
This work is licensed under a Creative Commons Attribution 4.0 International License , which permits unrestricted use, distribution, and reproduction in any medium, provided the original work is properly cited.