Open Access
ARTICLE
Preparation of Optically Active Polyimides Based on 2,2´-diamino-1,1´-binaphthalene
1
Department of Polymers
2
Department of Organic Chemistry
3
Deparment of Physical
Chemistry, University of Chemistry and Technology, Technická 5, 166 28 Prague 6, Czech
Republic
4 Institute of Chemical Process Fundamentals of the AS CR, Rozvojová 2, 165 02
Prague 6, Czech Republic.
* Corresponding Author: e-mail:
Journal of Polymer Materials 2022, 39(3-4), 325-335. https://doi.org/10.32381/JPM.2022.39.3-4.10
Abstract
Linear polyimides based on both of the 2,2´-diamino-1,1´-binaphthalene enantiomers and their racemic mixture were synthesized and characterized. A two-step synthetic route, consisting of the preparation of the polyimide precursor followed by its thermal imidization at temperatures not exceeding 200°C, was employed. The products were analyzed by FTIR and 1 H NMR spectroscopy. All of the tested materials were nearly fully imidized after 25 h. The final polyimides possess high glass transition temperatures, are thermo-oxidatively stable and are soluble in common organic solvents as 1-methyl-2-pyrrolidone, tetrahydrofuran and chloroform; additionally, those prepared from the enantiomers show optical activity. The values of specific optical rotations for the polymers were higher than those for the corresponding monomers. However, the directions of their optical rotations were identical. Due to their rather low molar masses, the final polyimides form brittle self-standing films. Thin layers of these products on porous polyimide substrates were made under forming composite membranes.Keywords
There is an increasing academic and industrial demand for enantiomeric compounds because they play an important role not only in biological systems on Earth but also in a number of branches of contemporary human activity (e.g., in drug/pharmaceutical production and asymmetric catalysis). Enantiomers, typically those of amino acids, are essential building-blocks of living organisms and are quite common in nature. Synthetically, enantiomers can be obtained by either a direct enantioselective synthesis (asymmetric synthesis) or by the synthesis of a racemic mixture followed by a separation procedure (chiral resolution).[1]
A drawback of low-molecular-weight enantiomeric compounds is their inability to form self-consisting products. The only organic compounds capable of forming such integrated matter are polymers. Optically active polymers are most frequently prepared by the polymerization of chiral monomers or by the incorporation of a chiral selector into the polymer structure. Then, the chiral polymers can be employed, for example, as enantioselective membranes. The polymer membrane-based method is a very promising method among the various chiral resolution procedures (crystallization, chromatography, etc.) due to its energy savings and ecologically friendly character.[2]
One of the perspective polymers that is not only usable in the field of enantioselective membranes[3] but also in practice as a gas-separation membrane[4] are polyimides (PI). These rigid polymers, with a high glass transition temperature, show an overall stability in the very broad temperature range of − 150 to + 200 °C. They are commonly synthesized by a two-step process via a polyimide precursor [polyamic acid, (PAA)]. PAA is synthesized from an aromatic dianhydride and an aromatic diamine in an amidic solvent e.g., 1-methyl-2-pyrrolidone, (NMP) at room temperature. PAA is often transformed (imidized) into PI by heating in the solid state at temperatures up to 230-300°C. If the final PI is soluble, then chemical imidization at temperatures of 20-100°C or solution imidization at temperatures of 160-200°C can be employed in some cases.[5] As chiral monomers built into the main PI chain, compounds such as 1,2-diaminocyclohexane, ethyl lysine dihydrochloride, and 2,2′-diamino-1,1′-binaphthalene (BND) have been used.[3]
Binaphthyl-containing products show several attractive properties due to their bulky naphthyl moieties with restricted rotations. They have been studied for use as polymeric chiral catalysts or enantioselective sensors.[6] Both enantiomeric dianhydrides[7] and diamines[8] have been used as the building blocks for the preparation of optically active PI. While the optically active dianhydrides can be prepared using complex multi-step syntheses[7,9], BND is commercially available in both its racemic (BND) and enantiomeric forms [(R)-1,1′-binaphthalene-2,2′-diamine (R-BND) and (S)-1,1′-binaphthalene-2,2′-diamine (S-BND)]. Due to the voluminous naphthyl groups, the chain-packing of the final polymers is imprecise, and they are more soluble in common organic solvents, in comparison with traditional PI, without the loss of their very good thermal stabilities. An increased level of the intrinsic nano/micro-porosity is supposed if the chiral monomer, R-BND, has been used, which is due to the geometry of the polymer chains.[8] The porosity increase may have a favorable impact on the permeability–selectivity relationship of the separating membrane on its base. On the other hand, such monomers, whose functional groups are tightly bound to bulky molecules, suffer from a lower reactivity than that of common PI, and the final products traditionally show rather low molar masses.[8] Consequently, their mechanical stability is often poor, and they produce brittle films. Thus, their use as self-standing membranes is restricted.
The 1,1′-binaphthalene-2,2′diyl units of the enantiomeric monomers introduce a certain level of optical activity to the final PI. Nevertheless, the optical behavior of the final polymers can differ from the parent monomers due to a change in their mobilities after their incorporation into the polymer chains and/or their (partial) racemization during polymerization. The probability of racemization depends on the character of the reaction mixture and its temperature.[8] The extent of potential side reactions during chemical reactions usually increases with reaction temperature. Binaphthydiamine has showed little racemization up to 210 °C (based on a liquid chromatography analysis).[10] Ritter[8] supposes (based on an X-ray scattering measurements) the degree of racemization of about 5% at the preparation of optically active PI based on R-BND by using a one-step reaction conducted in m-cresol at 200 °C for 5h. However, these findings have not been supported by the measurement of specific optical rotations. PI based on (S)-1,1′-binaphthalene-2,2′-diamine (S-BND) has not yet been studied in detail.
Therefore, we have decided to study in detail the employment of relatively low-temperature (not exceeding 200 °C) synthetic procedures combined with an optical rotation analysis of the final PI for this purpose. We did not succeed in the preparation of fully imidized products by using of chemical imidization at temperatures from 25 to 80 °C. Finally, the thermal imidization in solution followed by the solids isolation at temperatures not exceeding 200 °C was used in this work for the preparation of PI from both racemic (BND) and enantiomeric BND (R-BND and S-BND). The molecular parameters, solubility, optical activity and thermal properties of the products were determined. With regard to the potential brittleness of the polymeric products, which contained 1,1′-binaphthalene-2,2′diyl units, their thin layers were cast on a porous, crosslinked PI support. The enantioselectivity of membranes based on PI prepared from these enantiomeric monomers will be tested in future work.
4,4′-(Hexafluoroisopropylidene)diphthalic anhydride (6FDA) (TCI, Japan) was heated to 160 °C for 5 h under vacuum before use. 1,1′-Binaphthalene-2,2′-diamine (BND) (Aldrich, Czech Republic) was used as received. (R)-1,1′-Binaphthalene-2,2′-diamine (R-BND) and (S)-1,1′-binaphthalene-2,2′-diamine (S-BND) were prepared according to Yamamoto′s procedure[11], and their enantiomeric purities were determined by HPLC.[12] 1-Methyl-2-pyrrolidone (NMP) (Merck, Czech Republic) was distilled under vacuum over phosphorus pentoxide. 1, 2-Dichlorobenzene (Merck) was distilled under vacuum prior to use.
The polyamic acids (PAA) were synthesized in a three-necked flask equipped with a magnetic stirrer, nitrogen inlet/outlet and dropping funnel. The procedure is specified below for the reaction of the 6FDA and BND monomers: At room temperature, BND (0.65 g, 0.00229 mol) was dissolved in 16 ml of NMP. 6FDA 1.01 g (0.00228 mol) and an additional amount of NMP (16 ml) were added. After the 6FDA had dissolved, the reaction mixture was stirred at room temperature for 24 h.
The apparatus containing the PAA solution was equipped with an azeotrope trap (filled with 1, 2-dichlorobenzene) bearing a condenser with a drying tube and thermometer. After the addition of 20 vol% of 1, 2-dichlorobenzene (relative to NMP), the reaction mixture was heated in an oil bath at 180 - 200°C (a maximum temperature of the oil bath was 200°C) for 16 - 48 h. The homogeneous reaction mixture was then cooled to room temperature and precipitated with methanol. The collected PI was dried to a constant weight at temperatures not exceeding 200°C.
The composite membranes were prepared by casting a 5 wt% PI solution in NMP onto a polymeric substrate followed by solvent removal under controlled heating in an oven. The porous substrate (with a thin, dense covering layer, if necessary) was prepared from the PI Matrimid® by a method of phase inversion and crosslinking with 1,4-xylylenediamine.[13]
FTIR spectra were taken on a Nicolet 6700 spectrometer in reflective mode. 1H NMR spectra were recorded on a Bruker Avance III 500 MHz by using deuterated dimethyl sulfoxide (DMSO-d6) as the solvent. The molecular parameters of the materials were determined by a lab-made size-exclusion chromatograph (SEC) with an IR detector (tetrahydrofuran as a mobile phase, polystyrene standards) and an Ubbelohde capillary viscometer (NMP as a solvent, 25°C). Thermogravimetric analysis (TGA) was performed in air from 25 to 800°C with a temperature gradient of 10 °C min-1 using a TGA Q500 instrument. Differential scanning calorimetry (DSC) was performed in a nitrogen atmosphere from 25 to 400 °C with a temperature gradient of 10 °C min-1 using a DSC Q100 instrument. Microscopic analyses of the cross-sections of the materials were performed by using scanning electron microscopy (SEM, TESCAN). Specific optical rotations were measured at 880 nm in tetrahydrofuran at 20 °C on a JASCO P-2000 Polarimeter with a Peltier cell holder and a 0.1 m (= 1 dm) path length cell. The rotation values are given in deg cm3 g-1 dm-1 as an average value from 50 measurements with an error of ± 0.1-0.5 deg cm3 g-1 dm-1.
The main aim of this work was to determine the properties and, where applicable, potential usability as enantioselective membranes of polyimides (PI) based on racemic 1,1′-binaphthalene-2,2′-diamine (BND) and its enantiomeric forms, (R)-1,1′-binaphthalene-2,2′-diamine (R-BND) and (S)-1,1′-binaphthalene-2,2′-diamine (S-BND), prepared by thermal imidization, predominantly carried out in solution, at temperatures not exceeding 200 °C.
4,4′-(Hexafluoroisopropylidene)diphthalic anhydride (6FDA) was used as the dianhydride component. The hexafluoroisopropylidene groups [C(CF3)2], owing to their bulkiness, render the polymer chains sufficiently rigid and simultaneously reduce the interactions between chains, which results in an increased free volume that favorably impacts the transport of the selected species (e.g., gases) through the material.[14] In addition, this group has a beneficial effect on the reactivity of 6FDA towards diamine.[15]
The PI precursors are represented by the corresponding PAA based on 6FDA and racemic (BND) or enantiomeric (R-BND and S-BND) BND and are given as PAA(6FDA-BND), PAA(6FDA-R-BND), and PAA(6FDA-S-BND). To synthesize all of the PAAs, a dianhydride and diamine molar ratio of 1:1 was used with the aim of theoretically achieving the highest possible molar mass. The concentration of solids was 5 wt%. The transformation of PAA into the corresponding PI [PI(6FDA-BND) (Figure 1), PI(6FDA-R-BND), and PI(6FDA-S-BND)] was carried out by using thermal imidization in solution.[16]
Figure 1. Chemical structure of PI based on 6FDA and BND.
The initial tests were performed at 180-200 °C for 16 h. The reaction mixtures were homogeneous within the entire 16 h period. After precipitation with methanol, the products could be redissolved in NMP. However, it can be seen from examination of the FTIR spectra of the final products that imidization is not quite complete. The FTIR spectrum for PI(6FDA-R-BND) is shown in Figure 2. The band at 1675 cm-1, corresponding to the amide groups of the amide groups of the PI precursor (PAA), is still present in the PI spectrum. The absorption bands at 1785 and 1723 cm-1 (asymmetric and symmetric stretching of the ring carbonyl groups) and 1377 cm-1 (stretching of the ring C-N bond) characterize the formation of the imide structures.[16-17] The influence of prolonged heating at 180 - 200°C over a period of up to 48 h was monitored. All of the tested materials (separated from the reaction mixture; see Experimental part) were nearly fully imidized after 25 h, as shown by the FTIR spectrum of PI(6FDA-R-BND) in Figure 3. The characteristics of the spectra did not change as the materials were heated over an interval of 25 - 48 h.
Figure 2. FTIR spectrum of the product based on 6FDA and R-BND after thermal treatment at 180 - 200°C for 16 h.
Figure 3. FTIR spectrum of the PI(6FDA-R-BND) thermally treated at 180 - 200 °C for 25 h.
The results of elemental analysis show a good agreement between the expected polymer composition and experimental values (e.g., PI based on 6FDA and R-BND: calcd: C, 67.63%, H, 2.60%, N, 4.05%, found: C, 66.55%, H, 2.92%, N, 4.24%). The molar masses of the materials were determined by size-exclusion chromatography (SEC) with the use of tetrahydrofuran as the mobile phase and polystyrene standards for calibration. The values obtained are therefore only indicative because of the different chemical compositions of PI and polystyrene. All values of the number average molar mass, Mn, were on the order of thousands [and the corresponding intrinsic viscosities in the range of 10 - 20 cm3g-1 (in NMP at 25 °C)] and their polydispersities (Mw/Mn, where Mw is the weight average molar mass) were in the interval of 1.5 - 2.3. The achieved low molar masses result from the structure of the binaphthalenic diamines (a bulky molecule with tightly bound functional groups) and are close to the values that have been obtained by other authors.[8]
It is worth mentioning that the Mn of the PIs prepared by thermal imidization in solution were slightly lower than those prepared by thermal imidization in the solid state. McGrath[16] found that very low molecular mass fragments are temporarily formed during thermal imidization in solution and that their probability of reuniting depends on the accessibility of their functional groups. This opinion was supported by the 1H NMR analysis of the starting diamines (BND, R-BND and S-BND) and their corresponding polymers. The 1H NMR spectra of S-BND (Figure 4a) and PI(6FDA-S-BND) (Figure 4b) were taken in deuterated dimethyl sulfoxide. The signal at 4.62 ppm in the spectrum of S-BND belongs to its amino functional groups. The integration of the 4.62 ppm peak in comparison to the integration of the signals of the aromatic hydrogens in the region of 6.77 - 7.78 ppm indicates a sufficient purity for this compound. The insignificant signal at approximately 4.6 ppm is present in the spectrum of PI(6FDA-S-BNA). In addition to the signals of the aromatic hydrogens in the region of 7.10-8.03 ppm, a very weak, but remarkable, signal is seen in this spectrum at approximately 6.8 ppm. The authors[16] attribute this peak to the hydrogens of the terminal (i.e., unreacted) amino aromatic moieties.
Figure 4. 1H NMR spectra of S-BND (a top) and PI(6FDA-S-BND) (b bottom).
It is obvious from thermogravimetric analysis (TGA) that a corresponding weight loss of 10% lies for all materials in a similar temperature region. This weight loss occurred at 443 °C for PI(6FDA-S-BND) with a temperature gradient of 10 °C min-1 in an air atmosphere. The glass transition temperatures of the materials are all approximately in the temperature region of 300 - 310 °C.
A difference in the characteristics of the final products is observed from the values of their specific optical rotations [α]. Rotations were measured in tetrahydrofuran solutions (at 880 nm, 20°C) and were collected for both of the starting enantiomeric monomers (R-BND and S-BND) and their corresponding polymers [PI(6FDA-R-BND) and PI(6FDA-S-BND)] (Table 1). The values of [α] for the polymers were somewhat higher than those for the corresponding monomers. However, the directions of their optical rotations were identical. Opposite optical rotations of the polymers and their parent monomers can be explained by the predominant influence of the helical structure of these polymers over the optical rotation direction of the monomers.[18]
The solubilities of the products were monitored for their potential membrane preparation by solution casting. All of the products were found to be soluble in NMP, tetrahydrofuran and chloroform. Nevertheless, the self-standing membranes (i.e., those without any support) of each material, prepared from their corresponding solutions, were brittle. Handling the membranes was thus very difficult.
Therefore, we decided to cast solutions of these polymers onto a suitable substrate. A porous PI substrate (with pore sizes on the order of microns) based on the commercial product Matrimid® was chosen for this purpose. Matrimid® was crosslinked with p-xylylenediamine to be insoluble in NMP.[13, 17] NMP was used as the solvent because of its lower volatility compared to those of tetrahydrofuran and chloroform, which may contribute to the quality of the top layer. Figure 5 shows that the casting process provides a composite membrane with a relatively distinct porous substrate and a thin covering layer with a thickness of approximately 5 μm. However, further optimization of these composite membranes is necessary in future work.
Figure 5. SEM image of a composite membrane.
The values of the specific optical rotation of PI based on 6FDA and BND enantiomers have been determined. Their values (compared to those for the corresponding monomers) support our opinion that under selected reaction conditions the binaphthalenic moieties incorporated in polymer chains do not racemize. From this point of view, they can be considered as potential enantioselective materials. Nevertheless, it was shown in this work that the use of thermal imidization in solution has several difficulties in the preparation of binaphthyl-containing PIs. Prolonged heating for at least 25 h at 180 – 200 °C is needed to reach complete imidization. The combination of the monomers of 6FDA and either racemic or enantiomeric BND results in low molar mass products, which influences their considerable brittleness. A composite membrane composed of a porous substrate and a thin covering layer was successfully prepared. The enantioselectivity of such membranes, for example, towards 1,1′-binaphthalene-2,2′-diol (binol)[19], will be studied in future work.
Funding:: This work was supported by the Grant Agency of the Czech Republic under Grant 17-00089S.
References
1. R. Xie, L-Y. Chu and J-G. Deng, Chem. Soc. Rev. 37 (2008): 1243. [Google Scholar]
2. C. Fernandes, M. E. Tiritan and M. M. M. Pinto, Symmetry, 9 (2017): 19 pp. [Google Scholar]
3. C. Kang, J. Yan and L.Gao, Prog. Chem. 27 (2015): 59. [Google Scholar]
4. Al. Rybak, Au. Rybak, W. Kaszurawa, S. Awietjan, P. Sysel and Z. J. Grzywna, Mater. Letters, 208 (2017): 14. [Google Scholar]
5. D-J. Liaw, K-L. Wang, Y-C. Huang, K-R. Lee, J-Y. Lai and C-S. Ha, Prog. Polym. Sci. 37 (2012): 907. [Google Scholar]
6. A. Shockravi, A. Javadi and E. Abouzari-Loft, RSC Adv. 3 (2013): 6717. [Google Scholar]
7. Q. Mi, L. Gao and M. Ding, Macromolecules, 29 (1996): 5758. [Google Scholar]
8. N. Ritter, I. Senkovska, S. Kaskel and J. Weber, Macromol. Rapid Commun. 32 (2011): 438. [Google Scholar]
9. Q. Mi, L. Gao, Y. Ma, X. Zhang and M. Ding, J. Polym. Sci., Part A: Polym. Chem. 35 (1997): 3287. [Google Scholar]
10. D. C. Patel, R. M. Woods, Z. S. Breitbach, A. Berthod and D. W. Armstrong, Tetrahedron: Asymmetry, 28 (2017): 1557. [Google Scholar]
11. Y. Yamamoto, A. Sakamoto, T. Nishioka, J. Oda and Y. Fukuzawa, J. Org. Chem. 56 (1991): 1112. [Google Scholar]
12. R. Holakovsky, M. Marz and R. Cibulka, Tetrahedron: Asymmetry, 26 (2015): 1328. [Google Scholar]
13. V. Martin-Gil, W. Dujardin, P. Sysel, G. Koeckelberghs, I. F. J. Vankelecom and V. Fila, Sep. Pur. Technol. 210 (2019): 616. [Google Scholar]
14. W. Qiu, L. Xu, C-C. Chen, D. R. Paul and W. J. Koros, Polymer, 54 (20136226. [Google Scholar]
15. P. Sysel, M. Sochurek and P. Holler, Polymer, 39 (1998): 4751. [Google Scholar]
16. Y. J. Kim, T. E. Glass, G. D. Lyle and J. E. McGrath, Macromolecules, 26 (1993): 1344. [Google Scholar]
17. P. Sysel, D. Maly, J. Vysohlid, K. Friess, K. Pilnacek, M. Lanc and O. Vopicka, Polym. Eng. Sci. 57 (2017): 1367. [Google Scholar]
18. J. Zhi, Y. Guan, J. Cui, A. Liu, Z. Zhu, X. Wan and Q. Zhou, J. Polym. Sci.: Part A: Polym. Chem. 47 (2009): 2408. [Google Scholar]
19. X. Weng, J. E. Baez, M. Khiterer, M. Y. Hoe, Z. Bao and K. J. Shea, Angew. Chem. Int. Ed. 54 (2015): 11214. [Google Scholar]
Cite This Article
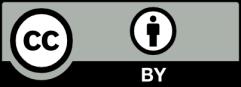
This work is licensed under a Creative Commons Attribution 4.0 International License , which permits unrestricted use, distribution, and reproduction in any medium, provided the original work is properly cited.