Open Access
REVIEW
Types and Sources of Microplastics; The Ubiquitous Environment Contaminant: A Review
Professor, Department of Applied Chemistry, Delhi Technological University, Delhi-110042.
* Corresponding Author: e-mail:
Journal of Polymer Materials 2022, 39(1-2), 17-35. https://doi.org/10.32381/JPM.2022.39.1-2.2
Abstract
Accumulation of Microplastics (MPs) in diverse ecosystems is a growing global problem of our time. These are entering into the environment directly (micro-sized plastics manufactured purposefully) and by degradation of plastic products. Due to a diverse range of polymers and additives used to manufacture plastic products, the MPs of different chemical compositions are abundant in the environment. A detailed literature survey revealed that MPs -environment interaction is largely governed by these chemicals and other intrinsic properties of MPs viz. shape, size, density, surface charge, etc. The current investigation primarily aims to review different chemical and physical factors of MPs that potentially influence their interaction with diverse ecosystems. The effort justifies keeping these parameters at the centre of the future researches in the field of plastics and the health of the environment and humans. Also, the piece of work highlights various sources of MPs that would help to take necessary measures for the judicious use of plastic products.Keywords
Plastic- Environment Interaction; A big threat from tiny particles
The synthesis of polymer (Bakelite) in the laboratory by Baekeland in 1907 was a turning point for material science researchers. It introduced the plastic age[1] and opened new doors for the material scientists to explore and develop polymers as engineering materials. About 72 years back, Yarsley and Couzens predicted the potentiality of plastics to replace localized natural resources for almost every needs[2]. Undoubtedly, the prediction has come true. Today, polymers have substituted almost all the traditional engineering materials like wood, metals, alloys, etc. It is due to the tremendous flexibility in modification of their properties by alteration of structural architecture or by using it in combination with other polymers (polymer blends) or dissimilar materials (composites) and/or mixing various additives. Consequently, the commercial production of plastics has increased enormously from 1.5 MT in the 1950s to over 380 MT per year in 2015[3]. With the unremitting broadening of application-spectrum, it is estimated that the production of plastics will be doubled in the next 20 years[4]. Subsequently, the consumption of fossil-fuel as a raw material to manufacture different plastics will increase from 4% of global oil production at present to 20% by 2050[5].
Besides the extensive societal benefits of plastics[6], the accumulation of plastics waste (PW) in the environment is a serious cause of concern[7-10]. Lebreton and Andrady 2019, projected that in the next 25 years under a business-as-usual scenario, the world-wide municipal plastic waste generation would be increased from the current amount of approx. 200 MT to around 230 MT annually. It could increase to 300 MT annually by 2040 and 380 MT by 2060[4]. In addition to this, ever- increasing mismanaged plastic waste including inadequately disposed and littered plastic waste is a giant problem of our time[7].
Physical, chemical, and biological weathering make plastics brittle[8], leading to disintegration into small fragments or even microscopic particles[9]. Through water or air currents and the food-chain[11-13] these tiny plastic-particles enter into the diverse eco-systems and adversely influence its functioning and stability[14-18]. Oceans act as sinks for these particles. In the aquatic environment these particles potentially intervene in the predator-prey relationship, act as a medium to spread toxic chemicals and pathogens, and disturb its biological diversity and balance[7][19-24]. For instance, a huge amount of these particles gets adsorbed on the marine or freshwater microalgae chlorella, Scenedesmus[25], and Skeletonema costatum[26] and inhibit photosynthesis by blocking air and light. Thus, interrupt the food chain at the very first stage that may lead to a disbalance of the eco-system. However, Yokota et al. 2017, reported the contrary result confirming that on plastic particles, the photosynthetic activities of cyanobacteria increased[27]. Thus, plastic particles create net autotrophic hotspot in the oligotrophic sea and affect the carbon, nutrient, and energy dynamics in ocean[28].
Objective of the Current Review
Plenty of information is available on the abundance of plastic particles in the environment and their impact reviewed on humans and biota. This first review judiciously classifies plastic particles according to their intrinsic properties (size, shape, and surface properties, etc.) that significantly influence their interaction with different components of the environment. The review also throws light on the origin of the concept of the microplastics to describe plastic particles and summarizes their various sources. The intention of the current effort is to initiate the re-evaluation of the strategies concerning the production of plastics and Plastic Waste Management (PWM) globally.
The fate of some of the plastic wastes on beaches and their disappearance overtime was first discussed by Scott, 1972[29]. According to his hypothesis, the photodegradation and oxidation convert plastics brittle that can be broken down into tiny particles by very small pressure. These particles are rapidly absorbed by the environment. This theory was further reinforced by Gregory, 1983[30]. He postulated that photodegradation and oxidation degrade the plastic pellets completely and disperse as dust.
At the same time, several reports have confirmed the abundance of plastic particles in the marine ecosystem. Buchanan 1971 observed a significant amount of synthetic fibres and larger fragments in a water sample collected from the North Sea[31]. The presence of plastic pellets and fragments was also reported in 1972 by Edward and Smith in the western Sargasso Sea[14]. He found pellet- shaped plastic particles with a diameter ranging from 0.25-0.5 cm. Later in 1974, Colton et al. confirmed the presence of polystyrene spherules and polyethylene cylinders and disks with a diameter ranging between 0.2-2.5 mm and 1.7-4.9 mm respectively in surface water of the North-Western Atlantic[32]. Thompson 2004 has obtained granules and a large number of plastic fibres with size ∼20 μm in samples collected from sediments and North-East Atlantic around Plymouth, UK[19].
Long term Investigation conducted by Thompson through the 1960s and 2000 recognized a correlation between all these findings. He proposed the concept of microscopic plastics and coined the term ‘microplastics’ (MP)[19]. He identified microscopic plastics of nine widely used polymers in the ocean and sedimentary habitat. The occurrence of microplastics is attributed to the fragmentation of degraded plastic products by mechanical action[33] and also to the micro-sized plastic particles used as abrasives in some cleansing agents[34]. The study offered an acceptable explanation for the disappearance of plastic wastes from beaches overtime as reported by Scott in 1972 and Gregory in 1983.
In a specific area of study, the microscopic plastic particles occur in various sizes, shapes and types of polymers. National Oceanic and Atmospheric Administration, USA, in 2009 has standardised sampling and defined microplastics as a fragment with size less than 5mm, and the lower size limit was suggested 333 μm[35].
In 2011, Andrady[36] suggested three size- based categories of microscopic plastics considering physical characteristics of each set of particles and their interaction with biological systems (Table 1). He documented that the efficiency of particles to accumulate toxins from its surroundings increase with a decrease in particle size.
Eriksen (2014) estimated the total number of plastic particles floating in the world's oceans and categorized them into four size classes- two microplastics, meso- and macro-plastics[15] (Table 2).
The microplastics are generally classified based on their origin in the environment and particle size. The literature survey revealed the dependency of microplastic-organism interaction and the toxicity profile of MPs on its size[37] and shape[38]. The size and shape of particles also significantly govern their distribution in ocean[39]. The surface charge of the microplastics influences their interaction with the biological system[25][40]. Recently, Machado et al. 2019 reported that the ability of microplastics to alter the biophysical properties of the soil and plant performance is mainly governed by their shape, size, and type of polymers[41]. With this background, I summarise herein, seven major classes of microplastics, in terms of:
a) Origin in the environment, b) Shape, c) Size, d) Colour, e) Type of polymer, f) Surface property (viz. charge), and g) Buoyancy of microplastics, with the following justification:
Class based on origin of MP in the environment
The two sub-categories of microplastics according to their origin in the environment are-primary and secondary microplastics. Primary Microplastics are micro-sized plastic objects manufactured purposefully for specific applications[20], viz ‘Microbeads’, Nurdles, and Plastic-based glitters. The origin of Secondary Microplastics in the environment is the fragmentation of larger plastic products by mechanical, chemical, radiation, or biological degradation[15, 19, 29, 30, 33, 42].
Fibrous MPs are among the most dominant types of microplastics observed in the natural environment[43-46]. Au et al. 2015, investigated the influence of the shape and texture of microplastics on its toxicity and absorption capability. He found polypropylene microfibres more toxic than spherical particles of polyethylene MP to the freshwater amphipod, Hyalella azteca. They concluded that fibres stay in the gut for a longer time causing serious harm to the organism[38]. Zubris and Richards 2005, considered synthetic fibres as rapid and semi-quantitative indicators of past sludge application[47]. Machado 2018, reported that the shape and size of polyester fibre are quite different from most naturally occurring soil components. Thereby strongly affect the biophysical environment of the common garden soil[48]. It is also recorded that polyester fibres and polyamide beads, with particle shape and size completely different from the naturally occurring soil particles, greatly affect the plant (Allium fistulosum) performance. The same paper revealed that the shape and size of cryomilled fragments of HDPE, PES, PP, and PS are similar to naturally occurring particles. Thus, instigate less effect in soil structure[41]. The General Ocean Turbulence Model (GOTM)- based investigation revealed that shape of MPs greatly affects their turbulent diffusivity; the fibres have the lowest velocity, followed by sheets and particles[49]. Based on the laboratory experiment Kowalski 2016, concluded that apart from the particle density, size and fluid density, the particles shape also influences the sinking rate of MPs of diverse size in fluid of different alkalinity[50].
These findings need more attention to assess the influence of microplastics' shape on its potentiality to impact the environment.
The MPs are classified based on their shape viz. Beads (or Spherules), Microspheres, Films, Irregular fragments, Cylinders (or Disks), and Fibres.
It is evident that the size of MPs plays a key role in their ingestion, bioaccumulation and toxicity profile in Biota and humans. Moore 2008, reported that lower trophic organisms ingest the particles, either synthetic or natural origin, of a specific size[51]. Lu et al. 2016 found that after 7-day exposure the polystyrene microplastic with size 20 μm accumulated in the gill and gut of zebra fish, while, smaller particles with a diameter size 5 μm entered in the fish liver also and demonstrated toxic effects[37].The cells of Fucus vesiculosus, restrict the dislocation of ∼20 μm polystyrene MP into the tissues because of its narrow passage[52]. These observations and work by Eriksen in 2014[15] emphasize the classification of MP based on their particle size as shown in Table 1 and 2.
MPs exhibit a variety of colours; from transparent or opaque to light (white, green, and yellow) or deep (blue, black, brown, tan, and red)[19, 53-56]. The investigations revealed varied results related to the colour of MPs and their distribution in test samples. It is well established now that the colour is an important parameter that facilitates the microplastics to enter into the food chain through visual predators. These organisms capture MPs by mistake due to resemblance with their food items. Carpenter et al. 1972 found that fish in coastal waters of southern New England ingested white, opaque PS spherules preferably over clear (crystalline) PS spherules[57]. Ding et al. 2019 isolated MPs from bivalve and fish of commercial value and found variation in colour related to the shape of MPs[58] (Table 3). The study, however, did not conclude that the test organisms were selective to microplastics' shape or colour or both.
Class based on the type of polymers
Thompson 2004, found micro-particles of 9 different polymers in 23 out of 30 samples and their occurrence was attributed to the fragmentation of larger items made of these polymers[19]. Since the existence of microplastics in the marine environment was discovered, their potentiality to adsorb and transport of toxic chemicals was known[14, 59-60]. Today, a good number of reports also confirmed the potentiality of microplastics to release toxic chemicals in different ecosystems. These chemicals, called additives (or process coadjutant), are mixed in polymers for manufacturing the final product (Table 4)[61].The selection of additives depends upon the type of polymer and the property required for the manufacturing process and final product as well. Most of the additives are not bonded chemically to the polymeric chain and therefore, released easily in the environment in which the plastic items are accumulated. Each additive has its own toxicity profile[62]. In addition to this, ample of papers are available demonstrating the degradation pattern of commodity polymers that share the largest market[63-71]. The rate and pattern of degradation of plastics are affected by various factors, like pH, light intensity, microbial attack, humidity, temperature, application conditions, additives, etc. producing an array of end-products and enhancing the contaminant spectrum in the system[72-73]. Machado et al., found that the type of polymer is a significant parameter that affects the ability of MPs to modify soil structure, plant traits, and bring in biogeochemical changes. For instance, the biogeochemical structure of soil and plant traits are less affected by the polymers composed of only carbon and hydrogen (HDPE, PE, PS, PET) as compared to the polymers having nitrogen in its basic structure, like polyamide[41].
This pre-existing knowledge can be used in two ways- Firstly, to predict the environmental condition of a specific area under investigation, and secondly, to provide the solution and treatment in time to neutralize the adverse effects of MPs. Thus, it is meaningful to classify the microplastics based on polymer type.
Currently, Knight et al. 2020 enlisted (Table 5) microplastics of 15 different types of polymers (including rubbers) based on scientific reports published during 2000-2019 on the related subject[74].
Class based on Surface Properties of Polymers
Just a decade ago, it has been revealed that the interaction between plastic particles and organisms is extensively governed by the surface properties of both of them. Bhattacharya et al. 2010 investigated the extent of affinity between model cellulose film, two algal species, Chlorella and Scenedesmus (containing cellulose in their cell walls) and positively and negatively charged PS beads of the size 20nm. The results established that i) positively charged PS beads were adsorbed preferentially by all the three substrates due to the slightly anionic nature of the cellulose surface. ii) the rough surface of the model cellulose film provided more binding sites causing the adsorption of an excessive amount of positively charged PS beads[25]. Nolte et al. 2017 also recorded the same observation. They confirmed that the cell wall of algae P. subcapitata has a stronger affinity to neutral or positively charged PS than negatively charged PS[40]. Sundbaek 2018 reported ∼94.5% sorption of positively charged PS-MP at the cut-site of Fucus vesiculosus due to the secretion of anionic polysaccharide-Alginate[52]. Mytilus galloprovincialis consumes more weathered microplastics preferentially over the virgin microplastics[75]. Hossain et al. 2018 found that the colonization of bacteria occurs more on eroded- PP disks than un-eroded PP surface[76]. Hydrophobic surface of plastic accumulates the hydrophobic persistent organic pollutants (POPs) from sea water[77]. Rochman 2015, reported the adsorption of heavy metals on the MPs surface[78]. Thus, MPs act as a means of transportation for diverse ‘adsorbed items’ to reach new locations (called rafting in the aquatic ecosystem).
Class based on buoyancy (Density)
In the marine eco-system, MPs are omnipresent, from the surface water, throughout the water column to the sediment[3][79]. In general, the MPs with a density lower than the surrounding water (viz. EPS – expanded Polystyrene, PU-Polyurethane, PP – polypropylene, LDPE - low density polyethylene, and HDPE - high density polyethylene with density range 0.02-0.06 to 0.94-0.96 g cm-3) floats near the surface or are suspended in sub-surface water, while MPs with high-density (viz. PS – polystyrene, PVC - polyvinyl chloride, PET - polyethylene terephthalate with density range 1.04-1.11 to 1.38-1.40 g cm-3) sink to the benthic environment. Ballent et al. 2012 reported that the vertical distribution of MPs is the result of the net influence of extrinsic turbulence factors and the intrinsic characteristics of microplastics[80]. Moreover, the MPs exhibit dynamic density behaviour leading to cyclic pattern of distribution in aquatic environment. Micro organisms aggregate on the surface of MPs and develop a biofilm. Thereby, increase the density to an extent that MPs floating on the surface tend to sink[81,82]. For example, PE food bag starts to sink below the sea surface as a result of biofouling within a period of three weeks[83]. Chubarenko et al. 2016 investigated some physical and dynamical properties of microplastic particles in marine environment of Baltic sea. He linked the residence time of different MPs at the water surface to their surface area. For a given mass, the time of fouling of the MPs up to the water density is directly proportional to the characteristic length scale (radius of sphere, thickness of the film, or a radius of a fiber). He estimated that biofouling of plastic bag, polyethylene fibers and spherical particles (and plastic pieces) takes 3-4 months, 6-8 months and 10-15 years respectively to sink in Baltic sea environment[84]. De-fouling of MPs causes them to return to water surface[36]. This process plays a key role in distributing chemicals and organisms throughout the water system as well as makes different types of MPs available to benthonic zone.
Sources for Primary Microplastics
Primary microplastics are manufactured for specific purposes in different shapes. These MPs enter into the environment through post-use discharge or accidental spillage. The sources of various primary MPs are listed below:
Microbeads of polyethylene, polylactic acid (PLA), polypropylene, polystyrene, or polyethylene terephthalate are used as scrubbers in some cosmetic products, toothpastes, and facial cleansers as a replacement for the traditional natural products like almonds or walnut husk, microcrystalline cellulose, oatmeal, or pumice[34][85-86]. Some industrial cleaning products also contain microplastics as abrasives[20][34][87]. These microbeads pass into the aquatic ecosystem through house-hold discharge. Such products act as the direct source of microplastics. As per an estimation-from one tube of exfoliating facewash more than three hundred fifty thousand microbeads enter into the environment[88].
Rochman et al. 2015 calculated that the United States alone releases 8 trillion microbeads per day into aquatic ecosystem from 17 wastewater treatment plants[89]. Murphy et al. 2016 reported that one wastewater treatment works in Scotland discharges 65 million microbeads per day in water[90].
Nurdles or plastic resin pellets (cylindrical, diameter 1-5 mm) are other examples copiously used primary microplastics that contaminate the environment. These are also called as ‘mermaid tears’. Nurdles are the pre-processing form of nearly all plastic products. Nurdles can enter into the environment during their production, storage, or mishandling during transportation and also during the manufacture of final plastic items[16][91-93]. It is supposed that these microplastics are accumulating in the environment since the 1940s when the commercial production of plastic was started[71] [19] [36]. However, it gained worldwide attention after the scientific reports published in the 1970s[14][57][91]. Sherrington 2016 estimated that two hundred thirty thousand tons of pellets per year are accumulating in the environment globally[94]. The estimated concentration of 300,000 to 1 million nurdles / mile on Mustang and North Padre Island, Texas in September 2018 was attributed to the offshore spillage during transportation[16].
Plastic based Glitters (PBG) are tiny, colourful, highly reflective particles. The usage of glitters as cosmetics dates back to ancient civilizations. Natural glitters like mica flakes were used in cave paintings. Plastic-based glitter was invented by Henry Ruschmann in 1934[95]. It is a metalized polymer (usually Aluminium- Biaxially oriented polyethylene terephthalate; Al- BoPET) coated with colouring agent (TiO2 of different thickness is commonly used for different colours) with marketed sizes as tiny as 0.15 mm. PBGs are manufactured in various shapes and colours. Other polymers viz. acrylic, Poly(methyl methacrylate) (PMMA), Polyvinyl Chloride (PVC) plastic epoxy resin mixture, or melamine and phenolic resin mixture are also used for this purpose. Plastic-based glitters made its way to the glamour world as a cosmetics ingredient replacing glass-glitters during the period of World War II. Currently, its ever-expanding applications, viz. in glittering ink and adhesives, on clothes, jewellery, shoes, and in craft material, etc. resulted in its boom production. For the last many years, the impact of PBGs on the environment was overlooked, probably due to the limited section of users. Now, it is well-established that the Plastic- based glitters are the potential primary microplastic contaminant[96-98]. Yet, reliable data on quantitative assessment of glitters' impact on ecosystems are insufficient.
Besides stamped as an environmental pollutant, PBGs enjoy the position of the valuable informer in different investigative studies. For instance, these glitters more often shed away from the surface they are attached to and stick to other surfaces including skin or clothes, very easily. Thus, hold a strong evidential value in forensic investigations[99-101]. Alexander and Juliana, 2019 reported the use of PBGs as a ‘flag-item’ or marker to understand the dynamic of microplastics from source to sink owing to its glittering appearance[102].
Sources of Secondary Microplastics
Fragmentation of larger plastic items or trashes is the chief source of secondary microplastics. Assuming a definite size of all the plastic particles for four size classes (Table 2), Eriksen 2014, quantified the fragmentation pattern of larger particles into smaller particles in marine environment (Figure 1)[15].
Figure 1: Fragmentation estimation of larger plastic particles into smaller fragments, based on particle size assumptions (Eriksen, 2014)[15]
Several other major sources of secondary microplastics are listed below:
Synthetic Textile The long-term investigation by Thompson, 2004 confirmed the presence of fibrous microplastic of the size ∼20 μm in diameter in the marine environment[19]. Napper and Thompson, 2016 revealed that washing of synthetic clothing, such as polyester and nylon fleece, in washing machine pushes a huge amount of microplastic fibres (upto 1900 fibres per wash) in the aquatic environment[103]. The polymer ingredients of these textile fibres are mainly polyester, polyethylene, acrylic or elastane, and polyamide[104-105].
Wearand tear of vehicle tiers and brake wear
Tire wear particles are produced by the abrasion of tires against roads[106]. Their composition differs due to the varied formulation of original tire[107].These are recognized as ubiquitous microplastics by Sundt et al. 2014[108]. Vehicle (Car, truck, and airplane) tire wear and brake wear are the microplastics that collectively contribute a lot to environment contamination[109-110]. As per an estimation, the worldwide generation of tire wear is nearly 6,000,000 tonnes per year[109]. Microplastics of unidentified synthetic rubber[111] and styrene-butadiene rubber[112] were recorded in water- sample collected from Jinhae Bay, Korea and in marine sediment in Terra Nova Bay, Antarctica respectively. However, the transportation of these microplastics to water bodies and ocean from road surfaces is still a subject for investigation.
A large portion (about 42 %;146 million tonnes) of total plastics produced globally is used by the packaging sector[3]. Yet the literature regarding packaging materials as a source of MPs in the packaged material is scanty. The occurrence of microplastics in plastic and glass bottled drinking water only has been reported. Primarily the MPs of PET and polyesters were identified in plastic bottled water[113-116]. It was inferred that the bottle material was the source of these MPs. The high abundance of MPs in glass bottled water is attributed to the abrasion of plastic caps with the glass body[117]. Current effort identified a knowledge gap here and invites the attention of scientists to carry out more exhaustive research in the subject.
Chipping of road markings and coatings
Generally, thermoplastics and epoxy are used for road markings and paintings[11]. For anticorrosive or antifouling coating on marine vessels polyurethane, epoxy and vinyl paints are used. Chipping of these paints as a result of weathering and/or abrasion by running vehicles is a substantial source of MPs.
City Dust: It includes all the sources which do not contribute much individually but together they increase significantly the environment pollution specifically in urban regions. City dust consists of the MPs produced by abrasion of footwear soles, furniture, garden accessories, utensils, building coatings, and artificial turf etc[104].
Since the ‘plastic age’ is still young and advancing, we have to develop efficient mechanisms and infrastructure for the safe disposal of plastic wastes. Currently, there are three key options for Plastic Waste Management (PWM): recycle, incineration, and landfill. Recycle is one of the 5 Rs proposed from a waste management perspective[118]. It is usually the best option with the lowest global warming potential and energy use. Ali Chamas et al. estimated that only 18% of PW is recycled[63]. It is probably due to the drawbacks associated with process and recycled products. The second preferred option is incineration to dispose of 24% PW. The remaining 58% of PW is dumped in landfills. It is a simple but non-sustainable approach for PWM. Firstly, due to the limited capacity of landfills[119] and secondly, the persistence of plastics causes diverse environmental hazards. For instance, toxic chemicals like phthalates and Bisphenol A, released from degrading plastic products, contaminate air, dust, and the aquatic environment[120-124]. Addition to it, Microplastics entered in water of the polar region of the earth, sediment, and sea ice[125], and even in Arctic Sea ice cores[126]. Being small in size, longevity and buoyancy microplastics travel long distances from the place of their origin through air or water currents. Harriet Paterson, a professor at the University of Western Australia viewed that more than 2.25 billion nurdles spilled from a ship in Durban, South Africa in 2017, creeped their way to Australian shores in about 450 days[127]. The ubiquitous nature of microplastics, necessitates of worldwide determined approach to ban the use of microplastics in various products and safer PWM as well.
Widespread use of plastics led to rapid increase in global plastic production consequently resulted in an increase in the amount of plastic contaminant in the form of microplastics in the environment. It is evident that ‘today, the microplastics are everywhere, from the deep sea, land to the air; in our food, in our water, and in the products of personal care’. Based on sizes, shapes and other physical characteristics, MPs exert diverse impact on all the components of an ecosystem. It is now well established that as a carrier of toxic chemicals, microorganisms and pathogens the MPs are posing serious threat to health of environment. The current effort is aimed to classify MPs on the basis of their intrinsic properties to draw the attention of researchers to evaluate the impact of MPs correlating with their physical properties. The identification of different sources of MPs will certainly encourage the consumers for judicious use of plastic products.
Conflict of interest statement: Author states that there is no conflict of interest.
References
1. American Chemical Society, “Leo Hendrick Baekeland and the Invention of Bakelite,” American Chemical Society, 9 November, 1993. Online. Available: http://www.acs.org/content/acs/en/education/whatischemistry/landmarks/bakelite.html. Accessed 23 september, 2020. [Google Scholar]
2. V. E. Yarsley and E. G. Couzens, Plastics, Middlesex: Penguin Books Limited., 1945. [Google Scholar]
3. R. Geyer, J. R. Jambeck and K. L. Law, “Production, Use, and Fate of All Plastics Ever Made.,” Sci. Adv., Vol. 3, No. 7, No. e1700782., p. 52, 2017. [Google Scholar]
4. L. Lebreton and A. Andrady, “Future scenarios of global plastic waste generation and disposal,” Palgrave Communications, Vol. 5, No. 6, pp. 111, 2019. [Google Scholar]
5. World Economic forum, “The new plastics economy. Rethinking the future of plastics.,” World Economic Forum, Geneva, Switzerland, 2016. [Google Scholar]
6. A. L. Andrady and M. A. Neal, “Applications and societal benefits of plastics. Philos,” Philos Trans R Soc B, Vol. 364, no. 1526, p. 1977-1984, 2009. [Google Scholar]
7. J. R. Jambeck, R. Geyer, C. Wilcox, T. R. Siegler, M. Perryman, A. Andrady, R. Narayan and K. L. Law, “Plastic waste inputs from land into the ocean,” Science, Vol. 347, No. 6223, pp. 768771, 2015. [Google Scholar]
8. D. K. A. Barnes, F. Galgani, R. C. Thompson and M. Barlaz, “Accumulation and fragmentation of plastic debris in global environments,” Phil. Trans. R. Soc. B, Vol. 364, p. 1985-1998, 2009. [Google Scholar]
9. K. N. Fotopoulou and H. K. Karapanagioti, “Degradation of Various Plastics in the Environment,” in 1st edt. [Barcelo, D, Kostianoy, A. G.(ed.)] The Handbook of Environmental Chemistry, Springer, 2017, p. 41-53. [Google Scholar]
10. R. C. Thompson, S. H. Swan, C. J. Moore and F. S. vom Saal, “Our Plastic Age.,” Philos. Trans. R. Soc, B, Vol. 364, no. 1526, p. 1973-1976, 2009. [Google Scholar]
11. K. Duis and A. Coors, “Microplastics in the aquatic and terrestrial environment: sources (with a specific focus on personal care productsfate and effects.” Environmental Sciences Europe, Vol. 28, no. 1, p. 2, 2016. [Google Scholar]
12. E. L. Teuten, “Transport and release of chemicals from plastics to the environment and to wildlife,” Phil. Trans. R. Soc. B, Vol. 364, p. 2027-2045, 2009. [Google Scholar]
13. D. Santillo, K. Miller and P. Johnston, “Microplastics as contaminants in commercially important seafood species.” Integr. Environ. Assess. Manage., Vol. 13, p. 516-521, 2017. [Google Scholar]
14. E. J. Carpenter and K. L. J. Smith, “Plastics on the Sargasso Sea Surface.” Science, Vol. 175, no. 4027, pp. 1240-1241, 1972. [Google Scholar]
15. M. Eriksen, L. Lebreton, H. Carson, M. Thiel, C. Moore, J. Borerro, F. Galgani, P. Ryan and J. Reisser, “Plastic Pollution in the World's Oceans: More than 5 Trillion Plastic Pieces Weighing over 250,000 Tons Afloat at Sea,” PLoS ONE, Vol. 9, no. 12, pp. 111913-111927, 2014. [Google Scholar]
16. W. Jace, H. D. Kelly, P. S. Lindsay and M. S. Kathleen, “Measuring plastic pellet (nurdle) abundance on shorelines throughout the Gulf of Mexico using citizen scientists: Establishing a platform for policy-relevant research,” Marine Pollution Bulletin, Vol. 151, p. 110794, 2020. [Google Scholar]
17. P. Sarah, L. Anna, G. J. L. Martin, D. Rachid, B. Christina and L. Christian, “Identification and quantification of macro- and microplastics on an agricultural farmland,” Scientific Reports, Vol. 8, p. 17950, 2018. [Google Scholar]
18. T. K. Marie, O. Sonja, L. Matthias and G. Hans-Peter, “The Eukaryotic Life on Microplastics in Brackish Ecosystems,” Frontiers in Microbiology, Vol. 10, p. 538, 2019. [Google Scholar]
19. R. C. Thompson, Y. Oslen, R. P. Mitchell, A. Davis, S. J. Rowland, A. W. John, D. McGonigle and A. E. Russell, “Lost at Sea: Where Is All the Plastic?,” Science, Vol. 304, no. 5672, p. 838, 2004. [Google Scholar]
20. C. Matthew, L. Pennie, H. Claudia and S. Tamara, “Microplastics as contaminants in the marine environment: a review,” Marine Pollution Bulletin, Vol. 62, no. 12, pp. 2588-2597, 2011. [Google Scholar]
21. A. Andrady, “The plastic in microplastics: a review,” Mar. Pollut. Bull., Vol. 119, no. 1, p. 12-22, 2017. [Google Scholar]
22. J. Zalasiewicz, N. W. Colin, I. d. S. Juliana, L. C. Patricia, D. B. Anthony, C. Alejandro, E. Matt, G. Agnieszka, J. Catherine, L. Reinhold et al., “The geological cycle of plastics and their use as a stratigraphic indicator of the Anthropocene.” Anthropocene, Vol. 13, p. 4-17, 2016. [Google Scholar]
23. P. D. R. Moeller, K. R. Beauchesne, K. M. Huncik, W. C. Davis, S. J. Christopher, P. Riggs-Gelasco et al., “Metal complexes and free radical toxins produced by Pfiesteria piscicida,” Environ. Sci. Technol, Vol. 41, p. 1166-1172, 2007. [Google Scholar]
24. M. K. Virsek, M. N. Lovsin, S. Koren, A. Kr˙an and M. Peterlin, “Microplastics as a vector for the transport of the bacterial fish pathogen species Aeromonas salmonicida.” Mar. Pollut. Bull., Vol. 125, p. 301-309, 2017. [Google Scholar]
25. P. Bhattacharya, L. Sijie, P. T. James and C. K. Pu, “Physical adsorption of charged plastic nanoparticles affects algal photosynthesis.” J Phys Chem C, Vol. 114, p. 16556-16561, 2010. [Google Scholar]
26. C. Zhang, X. Chen, J. Wang and L. Tan, “Toxic effects of microplastic on marine microalgae Skeletonema costatum: interactions between microplastic and algae.” Environ Pollut, Vol. 220, p. 1282-1288, 2017. [Google Scholar]
27. K. Yokota, H. Waterfield, C. Hastings, E. Davidson, E. Kwietniewski and B. Wells, “Finding the missing piece of the aquatic plastic pollution puzzle: interaction between primary producers and microplastics.” Limnol. Oceanogr. Lett., Vol. 2, p. 91-104, 2017. [Google Scholar]
28. J. A. Bryant, T. M. Clemente, D. A. Viviani, A. A. Fong, K. A. Thomas, P. Kemp et al., “Diversity and activity of communities inhabiting plastic debris in the North Pacific Gyre.” mSystems, Vol. 1, no. 3, pp. e00024-16, 2016. [Google Scholar]
29. G. Scott, “Plastics packaging and coastal pollution,” International Journal of Environmental Studies, Vol. 3, p. 35-36, (1972). [Google Scholar]
30. M. R. Gregory, “Virgin plastic granules on some beaches of eastern Canada and Bermuda,” Marine Environmental Research, Vol. 10, p. 73-92, 1983. [Google Scholar]
31. J. B. Buchanan, “Pollution by synthetic fibres,” Marine Pollution Bulletin, Vol. 2, p. 23, 1971. [Google Scholar]
32. J. Colton, B. Burns and D. K. Frederick, “Plastic Particles in Surface Waters of the Northwestern Atlantic.” Science, Vol. 185, no. 4150, pp. 491-497, 1974. [Google Scholar]
33. P. P. Klemchuk, “Degradable plastics: A critical review,” Polym. Degrad. Stab, Vol. 27, no. 2, pp. 183-202, 1990. [Google Scholar]
34. M. R. Gregory, “Plastic ‘scrubbers’ in hand cleansers: a further (and minor) source for marine pollution identified,” Marine pollution bulletin, Vol. 32, p. 867-871, 1996. [Google Scholar]
35. C. Arthur, J. Baker and H. Bamford, “Proc. International Research Workshop on the occurrence, effects and fate of microplastic marine debris,” NOAA Technical Memorandum NOS-OR&R3O, 2009. [Google Scholar]
36. A. L. Andrady, “Microplastics in the marine environment.,” Marine Pollution Bulletin, Vol. 62, no. 8, p. 1596-1605, 2011. [Google Scholar]
37. Y. Lu, Y. Zhang, Y. Deng, W. Jiang, Y. Zhao, J. Geng, L. Ding and H. Ren, “Uptake and accumulation of polystyrene microplastics in zebrafish (Danio rerio) and toxic effects in liver.,” Environ Sci Technol, Vol. 50, no. 7, p. 4054-4060, 2016. [Google Scholar]
38. S. Au, T. F. Bruce, B. W. C. and S. J. Klaine, “Responses of Hyalella azteca to acute and chronic microplastic exposures,” Environ Toxicol Chem, Vol. 34, no. 11, p. 2564-2572, 2015. [Google Scholar]
39. Y. Li, Hua Zhang and C. Tang, “A review of possible pathways of marine microplastics transport in the ocean,” Anthropocene Coasts, Vol. 3, p. 6-13, 2020. [Google Scholar]
40. T. Nolte, N. Hartmann, J. Kleijn, J. Garnas, d. M. D. van, A. J. Hendriks and A. Baun, “The toxicity of plasticnanoparticles to green algae as influenced by surface modification, medium hardness and cellular adsorption,” Aquat. Toxicol., Vol. 183, p. 11-20, 2017. [Google Scholar]
41. A. A. d. S. Machado, C. W. Lau, K. Werner, B. Joana, J. B. Bachelier, E. Faltin, R. Becker, A. S. Gorlich and M. C. Rillig, “Microplastics Can Change Soil Properties and Affect Plant Performance,” Environ. Sci. Technol, Vol. 53, p. 6044-6052, 2019. [Google Scholar]
42. F. Galgani, H. G. S. Werner and D. L. Vrees, “Marine litter within the European marine strategy framework directive,” ICES J Mar Sci, Vol. 70, no. 6, p. 1055-1064, 2013. [Google Scholar]
43. R. Dris, J. Gasperi, M. Saad, C. Mirande and B. Tassin, “Synthetic fibers in atmospheric fallout: a source of microplastics in the environment?,” Mar Pollut Bull, Vol. 104, p. 290-293, 2016. [Google Scholar]
44. R. Dris, J. Gasperi, C. Mirande, C. Mandin, M. Guerrouache, V. Langlois and B. Tassin, “A first overview of textile fibers, including microplastics, in indoor and outdoor environments,” Environ Pollut, Vol. 221, p. 453-458, 2017. [Google Scholar]
45. M. Claessens, D. M. S., L. L. Van, K. De Clerck and C. Janssen, “Occurrence and distribution of microplastics in marine sediments along the Belgian coast.,” Marine Pollution Bulletin, Vol. 62, no. 10, p. 2199e2204, 2011. [Google Scholar]
46. M. Browne, P. Crump, S. Nivens, E. Teuten, A. Tonkin, T. Galloway and R. Thompson, “Accumulation of microplastics on shorelines worldwide: sources and sinks,” Environmental Science and Technology, Vol. 45, no. 21, p. 9175e9179, 2011. [Google Scholar]
47. K. A. V. Zubris and B. K. Richards, “Synthetic fibers as an indicator of land application of sludge,” Environ. Pollut., Vol. 138, p. 201-211, 2005. [Google Scholar]
48. A. A. de Souza Machado, C. W. Lau, J. Till, W. Kloas, A. Lehmann, R. Becker and M. C. Rillig, “Impacts of Microplastics on the Soil Biophysical Environment,” Environ. Sci. Technol, Vol. 52, no. 17, p. 9656-9665, 2018. [Google Scholar]
49. J. Reisser, B. Slat, K. Noble, K. du Plessis, M. Epp, M. Proietti, al., S. J. de, B. T. and C. Pattiaratchi, “The vertical distribution of buoyant plastics at sea: An observational study in the North Atlantic Gyre.,” Biogeosciences, Vol. 12, p. 1249-1256, 2015. [Google Scholar]
50. N. Kowalski, A. Reichardt and J. Waniek, “Sinking rates of microplastics and potential implications of their alteration by physical, biological, and chemical factors.,” Mar. Pollut. Bull., Vol. 109, p. 310-319, 2016. [Google Scholar]
51. C. J. Moore, “Synthetic polymers in the marine environment: a rapidly increasing, long-term threat,” Environmental Research, Vol. 108, no. 2, pp. 131-139, 2008. [Google Scholar]
52. K. B. Sundbak, W. K. I. Due, V. C. Greve, N. S. Rasmussen, H. S. Lovstad and N. B. Hartmann, “Sorption of fluorescent polystyrene microplastic particles to edible seaweed Fucus vesiculosus,” Journal of Applied Phycology, Vol. 30, no. 5, p. 2923-2927, 2018. [Google Scholar]
53. A.L.Lusher, M. McHugh and R.C.Thompson, “Occurrence of microplastics in the gastrointestinal tract of pelagic and demersal fish from the English Channel,” Marine Pollution Bulletin, Vol. 67, no. 1-2, pp. 94-99, 2013. [Google Scholar]
54. J. Bellas, J. Mart'ýnez-Armental, A. Mart'ýnez-C'amara, V. Besada and C. Mart'ýnez-G'omez, “Ingestion of microplastics by demersal fish from the Spanish Atlantic and Mediterranean coasts,” Mar. Pollut. Bull., Vol. 109, p. 55-60, 2016. [Google Scholar]
55. H. Li, L. Ma, L. Lin, Z. Ni, X. Xu, H. Shi, Y. Yan, G. Zheng and D. Rittschof, “Microplastics in oysters Saccostrea cucullata along the Pearl River estuary,” China Environ. Poll., Vol. 236, p. 619-625, 2018. [Google Scholar]
56. A. M. Young and J. A. Elliott, “Characterization of microplastic and mesoplastic debris in sediments from Kamilo Beach and Kahuku Beach, Hawai,” Marine Pollution Bulletin, Vol. 113, p. 477-482, 2016. [Google Scholar]
57. E. Carpenter, S. Anderson, G. Harvey, H. Miklas and B. Peck, “Polystyrene spherules in coastal waters,” Science, Vol. 178, no. 4062, p. 749-750, 1972. [Google Scholar]
58. D. Jinfeng, L. Jingxi, C. Sun, F. Jiang, J. Peng, Q. Lingyun, Z. Yifan and H. Changfei, “Detection of microplastics in local marine organisms using a multi-technology system,” Anal. Methods, Vol. 11, pp. 78-87, 2019. [Google Scholar]
59. J. G. B. Derraik, “The pollution of the marine environment by plastic debris: a review,” Marine Pollution Bulletin, Vol. 44, p. 842-852, 2002. [Google Scholar]
60. S. O., V. Fleming-Lehtinen and M. Lehtiniemi, “Ingestion and transfer of microplastics in the planktonic food web,” Environ Pollut, Vol. 185, p. 77-83, 2014. [Google Scholar]
61. A. Veronica, C. Cosimo, C. Pierfrancesco and M. Valentina, “Additives in Polymers Chapter: 4,” in Modification of Polymer Properties, William Andrew Publishing, 2016. [Google Scholar]
62. J. Hahladakis, C. Velis, R. Weber, E. lacovidou and P. Purnell, “An overview of chemical additives present in plastics: migration, release, fate and environmental impact during their use disposal and recycling,” J Hazard Mater, Vol. 344, p. 179-199, 2018. [Google Scholar]
63. C. Ali, H. Moon, Z. Jiajia, Q. Yang, T. Tarnuma, H. J. Jun, A.-O. Mahdi, L. S. Susannah and S. Sangwon, “Degradation Rates of Plastics in the Environment,” ACS Sustainable Chem. Eng., Vol. 8, p. 3494-3511, 2020. [Google Scholar]
64. L. G. Close, R. D. Gilbert and R. E. Fornes, “Poly(Vinyl Chloride) Degradation - A Review.” Polym. Plast. Technol. Eng. 1977, 8 (2p. 177-198, [Google Scholar]
65. J. Yu, L. Sun, C. Ma, Y. Qiao and H. Yao, “Thermal Degradation of PVC: A Review,” Waste Manage, Vol. 48, p. 300-314, 2016. [Google Scholar]
66. P. Gijsman, J. Hennekens and J. Vincent, “The Mechanism of the Low-Temperature Oxidation of Polypropylene,” Polym. Degrad. Stab., Vol. 42 , no. 1, p. 95-105, 1993. [Google Scholar]
67. H. J. Oswald and E. Turi, “The Deterioration of Polypropylene by Oxidative Degradation.,” Polym. Eng. Sci., Vol. 5, no. 3, p. 152-158, 1965. [Google Scholar]
68. D. J. Carlsson and D. M. Wiles, “The Photooxidative Degradation of Polypropylene. Part II. Photostabilization Mechanisms,” J. Macromol. Sci., Polym. Rev., Vol. 14, no. 2, p. 155-192, 1976. [Google Scholar]
69. I. C. McNeill, M. Zulfiqar and T. Kousar, “A Detailed Investigation of the Products of the Thermal Degradation of Polystyrene,” Polym. Degrad. Stab., Vol. 28, no. 2, p. 131-151, 1990. [Google Scholar]
70. E. Yousif and R. Haddad, “Photodegradation and Photostabilization of Polymers, Especially Polystyrene: Review,” Springer Plus, Vol. 2, no. 1, p. 398, 2013. [Google Scholar]
71. N. Grassie and G. Scott, Polymer Degradation and Stabilisation, Cambridge: Cambridge University Press, 1988. [Google Scholar]
72. P. Wexler and S. Gad, Encyclopedia of toxicology, New York: Academic Press, 1998. [Google Scholar]
73. J. Huff, P. Chan and R. Melnick, “Clarifying carcinogenicity of ethylbenzene.,” Regul Toxicol Pharmacol, Vol. 58, no. 2, p. 167-169, 2010. [Google Scholar]
74. J. K. Lydia, N. F. P.-J. Florence, A.-S.-C. Maya and C. T. Richard, “Tyre wear particles: an abundant yet widely unreported microplastic?,” Environmental Science and Pollution Research, Vol. 27, p. 18345-18354, 2020. [Google Scholar]
75. I. L. N. Br°ate, M. Bl´azquez, S. J. Brooks and K. V. Thomas, “Weathering impacts the uptake of polyethylene microparticles from toothpaste in Mediterranean mussels (M. galloprovincialis),” Sci. Total Environ., Vol. 626, p. 1310-1318, 2018. [Google Scholar]
76. R. H. Mohammed, J. Miao, W. QiHuo and G. L. Laura, “Microplastic surface properties affect bacterial colonization in freshwater,” J Basic Microbiol., Vol. 59, p. 54-61, 2019. [Google Scholar]
77. E. Teuten, et al., S. J.R., S. G. Tamara and T. R.G., “Potential for plastics to transport hydrophobic contaminants,” Environmental Science & Technology, Vol. 41, no. 22, p. 7759-7764, 2007. [Google Scholar]
78. C. Rochman, “The complex mixture, fate and toxicity of chemicals associated with plastic debris in the marine of chemicals associated with plastic debris in the marine, L. G. a. M. K. M. Bergmann, Ed., New York.: Springer: New York., 2015. [Google Scholar]
79. J.-P. Desforges, M. Galbraith, N. Dangerfield and P. Ross, “Widespread distribution of microplastics in subsurface seawater in the NE Pacific Ocean,” Mar. Pollut. Bull., Vol. 79, p. 94-99, 2014. [Google Scholar]
80. A. Ballent, A. Purser, P. de Jesus Mendes, S. Pando and L. Thomsen, “Physical transport properties of marine microplastic pollution.,” Biogeosci. Discuss., Vol. 9, p. 18755-18798, 2012. [Google Scholar]
81. A. Cozar, F. Echevarria, J. Gonzalez-Gordillo, X. Irigoien, B. Ubeda, S. Hernandez-Leon and et al., “Plastic debris in the open ocean.,” Proc. Natl. Acad. Sci. USA, Vol. 111, p. 10239-10244, 2014. [Google Scholar]
82. C. Moore, S. Moore, M. Leecaster and S. Weisberg, “A comparison of plastic and plankton in the North Pacific central gyre.,” Mar. Pollut. Bull., Vol. 42, p. 1297-1300, 2001. [Google Scholar]
83. D. Lobelle and M. Cunliffe, “Early microbial biofilm formation on marine plastic debris.,” Marine Pollution Bulletin, Vol. 62, no. 1, pp. 197-200, 2011. [Google Scholar]
84. C. I., B. A., Z. M. and E. E., “On some physical and dynamical properties of microplastic particles in marine environment,” Marine Pollution Bulletin, Vol. 108, no. 1-2, pp. 105-112, 2016. [Google Scholar]
85. I. E. Napper, A. Bakir, S. Rowland and R. C. Thompson, “Characterisation, quantity and sorptive properties of microplastics extracted from cosmetics,” Marine Pollution Bulletin, Vol. 99, no. 1-2, p. 178-185, 2015. [Google Scholar]
86. Z. H. Rana, M. S. A. Morog, M. M. Reem, G. Furtuna, E. Marim, F. Wajeeh, C. Nizamudeen, I. AbdelHamid, T. Thies and A. K. Ruwaya, “Analysis of microbeads in cosmetic products in the United Arab Emirates,” Environmental Pollution, Vol. 258, p. 113831, 2020. [Google Scholar]
87. V. Zitko and M. Hanlon, “Another source of pollution by plastics: Skin cleaners with plastic scrubbers.” Marine Pollution Bulletin, Vol. 22, p. 41-42, 1991. [Google Scholar]
88. Blake Kopcho, Center for Biological Diversity, 28 December 2015. Online Available: https://www.biologicaldiversity.org/news/press_releases/2015/plastic-microbeads-12-28-2015b.html. Accessed 17 September, 2020. [Google Scholar]
89. M. R. Chelsea, M. K. Sara, B. A. Jonathan, T. B. Michael, S. D. Emily, J. G. Stephanie, R. S. Ashley and V. Diogo, “Scientific Evidence Supports a Ban on Microbeads,” Environmental Science & Technology, Vol. 49, p. 10759-10761, 2015. [Google Scholar]
90. M. Fionn, E. Ciaran, C. Frederic and Q. Brian, “Wastewater treatment works (WwTW) as a source of microplastics in the aquatic environment,” Environmental Science & Technology, Vol. 50, no. 11, p. 5800-5808, 2016. [Google Scholar]
91. M. R. Gregory, “Accumulation and distribution of virgin plastic granules on New Zealand beaches,” N. Z. J. Mar. Freshwater Res., Vol. 12, no. 4, p. 399-414, 1978. [Google Scholar]
92. K. J. McDermid and T. L. McMullen, “Quantitative analysis of small-plastic debris on beaches in the Hawaiian archipelago.” Mar. Pollut. Bull., Vol. 48, p. 790-794, 2004. [Google Scholar]
93. T. Karlsson, L. Arneborg, G. Brostrom, B. Almroth, L. Gipperth and M. Hassellov, “The unaccountability case of plastic pellet pollution,” Marine Pollution Bulletin, Vol. 129, pp. 52-60, 2018. [Google Scholar]
94. C. Sherrington, “Plastics in the marine environment.” Report to European Commission, 1-13, Eunomia, 2016. [Google Scholar]
95. M. Aja, “Glitter: A Brief History,” New York, 4 October 2007. [Google Scholar]
96. Y. Meral, “Glitters as a Source of Primary Microplastics: An Approach,” Journal of Agricultural and Environmental Ethics, Vol. 32, p. 459-478, 2019. [Google Scholar]
97. Massey University, “Social scientist's call on plastic glitter ban goes global,” Massey University, 4 December, 2017. Online Available:https://www.massey.ac.nz/massey/aboutmassey/news/article.cfm?mnarticle_uuid=620D35A0-8897-46B0-9317-367CE309555F. Accessed 23 September, 2020. [Google Scholar]
98. W. Gael, “ESR,” 19 December, 2018. Online Available: https://www.esr.cri.nz/home/about-esr/media-releases/best-to-tone-down-the-tinsel/. Accessed 23 September, 2020. [Google Scholar]
99. M. C. Grieve, “Glitter particles—An unusual source of trace evidence,” Journal of the Forensic Science Society, Vol. 27, no. 6, p. 405-412, 1987. [Google Scholar]
100. B. Blackledge, “Research Gate,” August 2007. Online Available: https://www.researchgate.net/publication/262639620_GLITTER_as_Forensic_Evidence. Accessed 23 September 2020. [Google Scholar]
101. S. Gross, K. Igowsky and E. Pangerl, “Glitter as a source of trace evidence.,” Journal of the American Association of Trace Evidence Examiners, Vol. 1, no. 1, p. 62-72, 2010. [Google Scholar]
102. S. Alexander and A. d. S. Juliana, “Is this your glitter? An overlooked but potentially environmentally-valuable microplastic,” Marine Pollution Bulletin, Vol. 146, pp. 50-53, 2019. [Google Scholar]
103. I. Napper and R. Thompson, “Release of synthetic microplastic plastic fibres from domestic washing machines: effects of fabric type and washing conditions,” Marine Pollution Bulletin, Vol. 112, no. 1-2, p. 39-45, 2016. [Google Scholar]
104. R. Essel, L. Engel, M. Carus and R. Ahrens, “Sources of microplastics relevant to marine protection in Germany,” http://www.umweltbundesamt.de/publikationen/sources-of-microplastics-relevant-to-marine, 2015. [Google Scholar]
105. J.-Q. Jiang, “Occurrence of microplastics and its pollution in the environment: a review.,” Sustain Prod Consum, Vol. 13, p. 16-23, 2018. [Google Scholar]
106. M. Kreider, J. Panko, B. McAtee, L. Sweet and B. Finely, “Physical and chemical characterization of tire-related particles: comparison of particles generated using different methodologies.,” Sci Total Environ, Vol. 408, p. 652-659, 2010. [Google Scholar]
107. S. Wagner, T. Huffer, P. Klockner, M. Wehrhahn, T. Hofmann and T. Reemtsma, “Tire wear particles in the aquatic environment - a review on generation, analysis, occurrence, fate and effects.,” Water Res, Vol. 139, p. 83-100, 2018. [Google Scholar]
108. P. Sundt, P. Schulze and F. Syversen, “Sources of microplastic-pollution to the marine environment. Mepex. Norwegian Environment,” Mepex. Norwegian Environment Agency, 2014. [Google Scholar]
109. J. K. Pieter, J. L. Ansje, G. A. J. V. B. Frank and M. J. R. Ad, “Wear and Tear of Tyres: A Stealthy Source of Microplastics in the Environment,” Int J Environ Res Public Health, Vol. 14, no. 10, p. 1265, 2017. [Google Scholar]
110. B. Baensch-Baltruschat, B. Kocher, F. Stock and G. Reifferscheid, “Tyre and road wear particles (TRWP) - A review of generation, properties, emissions, human health risk, ecotoxicity, and fate in the environment,” Sci Total Environ, Vol. 733, p. 137823, 2020. [Google Scholar]
111. Y. Song, S. Hong, M. Jang, M. G. Han and W. J. Shim, “Occurrence and distribution of microplastics in the sea surface microlayer in Jinhae Bay, South Korea.,” Arch Environ Contam Toxicol, Vol. 69, p. 279-287, (2015). [Google Scholar]
112. C. Munari, V. Infantini, M. Scoponi, E. Rastelli, C. Corinaldesi and M. Mistri, “Microplastics in the sediments of Terra Nova Bay (Ross Sea Antarctica),” Mar Pollut Bull, Vol. 122, p. 161-165, 2017. [Google Scholar]
113. S. A. Mason, V. G. Welch and J. Neratko, “Synthetic polymercontamination in bottled water.,” Front. Chem., Vol. 6, p. 407, 2018. [Google Scholar]
114. B. E. Ossmann, G. Sarau, H. Holtmannspotter, M. Pischetsrieder, S. H. Christiansen and W. Dicke, “Small-sized microplastics and pigmented particles in bottled mineral water,” Water Res., Vol. 141, p. 307-316, 2018. [Google Scholar]
115. D. Schymanski, C. Goldbeck, H. U. Humpf and P. Furst, “Analysis of microplastics in water by micro-Raman spectroscopy: Release of plastic particles from different packaging into mineral water,” Water Res., Vol. 129, p. 154-162, 2018. [Google Scholar]
116. P. Zuccarello, M. Ferrante, A. Cristaldi, C. Copat, A. Grasso, D. Sangregorio, M. Fiore and G. O. Conti, “Exposure to microplastics (<10 μm) associated to plastic bottles mineral water consumption: Thefirst quantitative study,” Water Res., Vol. 157, p. 365-371, 2019. [Google Scholar]
117. W. Uhl, M. Eftekhardadkhah and C. Svendsen, “Mapping microplastic in Norwegian drinking water,” Norwegian Water BA, 2018. [Google Scholar]
118. J. Hopewell, R. Dvorak and E. Kosior, “Plastics recycling challenges and opportunities.,” Phil. Trans. R. Soc. B, Vol. 364, p. 2115-2126, 2009. [Google Scholar]
119. E. Defra, S. Wilson and M. Hannan, “Review of England's waste strategy.,” Environmental report under the ‘SEA’ directive UK: DEFRA., London, 2006. [Google Scholar]
120. R. A. Rudel, J. G. Brody, J. C. Spengler, J. Vallarino, P. W. Geno, G. Sun and A. Yau, “Identification of selected hormonally active agents and animal mammary carcinogens in commercial and residential air and dust samples,” J. Air Waste Manag. Assoc., Vol. 51, p. 499-513, 2001. [Google Scholar]
121. R. A. Rudel, D. E. Camann, J. D. Spengler, L. R. Korn and J. G. Brody, “Phthalates, alkylphenols, pesticides polybrominated diphenyl ethers, and other endocrine disrupting compounds in indoor air and dust.” Environ. Sci. Technol., Vol. 37, p. 4543-4553, 2003. [Google Scholar]
122. J. D. Meeker, S. Sathyanarayana and S. H. Swan, “Phthalates and other additives in plastics: human exposure and associated health outcomes,” Phil. Trans. R. Soc. B, Vol. 364, p. 2097-2113, 2009. [Google Scholar]
123. J. Oehlmann and et al., “A critical analysis of the biological impacts of plasticizers on wildlife,” Phil. Trans. R. Soc. B, Vol. 364, p. 2047-2062, 2009. [Google Scholar]
124. J. Prata, “Airborne microplastics: consequences to human health,” Environ Pollut, Vol. 234, p. 115-126, 2018. [Google Scholar]
125. R. Obbard, “Microplastics in Polar Regions: The role of long range transport,” Current Opinion in Environmental Science & Health, Vol. 1, pp. 24-29, 2018. [Google Scholar]
126. R. W. Obbard, S. Sadri, Y. Q. Wong, A. A. Khitun, I. Baker and R. C. Thompson, “Global warming releases microplastic legacy frozen in Arctic Sea ice,” Earth's Future, Vol. 2, no. 6, pp. 315-320, 2014. [Google Scholar]
127. E. H. Schumann, F. C. MacKay and N. A. Strydom, “Nurdle drifters around South Africa as indicators of ocean structures and dispersion,” South African Journal of Science, Vol. 115, no. 5/6, 2019. [Google Scholar]
Cite This Article
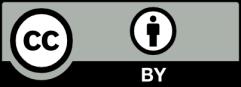
This work is licensed under a Creative Commons Attribution 4.0 International License , which permits unrestricted use, distribution, and reproduction in any medium, provided the original work is properly cited.