Open Access
ARTICLE
Experimental Study on Improving Performance and Productivity of Pyramid Solar Still Using Rotation Technique
School of Mechanical Engineering, Iran University of Science and Technology, Tehran, Iran
* Corresponding Authors: Sasan Asiaei. Email: ; Sayed Mostafa Hosseinalipour. Email:
(This article belongs to the Special Issue: Multiscale Heat and Mass Transfer and Energy Conversion)
Frontiers in Heat and Mass Transfer 2024, 22(3), 955-976. https://doi.org/10.32604/fhmt.2024.051532
Received 07 March 2024; Accepted 14 May 2024; Issue published 11 July 2024
Abstract
Globally, potable water scarcity is pervasive problem. The solar distillation device is a straightforward apparatus that has been purposefully engineered to convert non-potable water into potable water. The experimental study is distinctive due to the implementation of a rotational mechanism within the pyramidal solar still (PSS), which serves to enhance the evaporation and condensation processes. The objective of this research study is to examine the impact of integrating rotational motion into pyramidal solar stills on various processes: water distillation, evaporation, condensation, heat transfer, and energy waste reduction, shadow effects, and low water temperature in saline environments. Ultimately, the study aims to enhance the production of distilled water. An economic evaluation was undertaken in order to ascertain the extent of cost reduction. Experiments measuring freshwater productivity and thermal performance were conducted over a three-month period at the University of Science and Technology in Tehran. The entire pyramid structure was rotated using a direct current motor driven by a photovoltaic cell. The research methodology entailed the operation of a PSS with varying rotational speeds (0.125, 0.25, 1, and 1.5 rpm) and without rotation, from 9 am to 4 pm. The findings suggested that the productivity of the distillation apparatus in terms of distilled water increased as the rotation speed rose, with the most pronounced increase occurring at 1 rpm in comparison to the other conditions. The presence of turbulence in the water enhanced the heat transfer occurring between the absorber plate and the water. At 2:00 p.m. on an experimental day, this effect was observed when the absorber plate temperature reached 79.1°C at 1.5 rpm. In contrast, its temperature decreased to 78°C when not in a state of rotation, as the intensity of solar radiation was higher in the non-rotation state. At 1 rpm, the solar pyramid distiller achieved a 30.2% increase in output compared to its non-rotating state. At 1 rpm, the distiller achieved a 20.6% increase in output compared to 0.25 revolutions per minute. In addition to the control condition, the thermal efficiency of the solar still varied as follows: at 1, 1.5, 0.25, and 0.125 rpm, it was 46.2%; at 44.2%, 37.8%; at 35.3%; and at 36.6%, respectively. Furthermore, distilled water generated by a pyramid solar still with rotation (PSSR) is priced at $0.03 per liter, whereas it costs $0.0317 per liter when produced by a pyramid solar still without rotation (PSS without R).Keywords
Nomenclature
PSS | Pyramid solar still |
PSSR | Pyramid solar still with rotating |
PSS without R | Pyramid solar still without rotating |
˙m˙m | Hourly productivity from solar still, (kg/m2×m2× h) |
I(t)I(t) | Daily average solar radiation, (W/m2m2) |
$ | US dollars |
l | Liter, (L) |
hfghfg | latent heat of water vaporization, (kJ/kg) |
TwTw | Water temperature, (°C) |
ηdηd | Daily thermal efficiency % |
A | System area, (m2)(m2) |
AeffAeff | Effect area, (m2)(m2) |
T | Temperature, (°C) |
V | Wind speed, (m/s) |
t | Time in seconds |
WRWR | Uncertainty of the result % |
P | power |
a | Ambient |
b | Base |
w | Water |
d | Daily |
g | Glass |
The global water scarcity is a critical problem caused by an in equilibrium between the existing water resources and the increasing need for water. The fact that less than 1% of the Earth’s water is both potable and readily available to humans is concerning. Consequently, there is a genuine apprehension regarding an impending water scarcity in the future. By 2030, the United Nations forecasts that 40% of the world’s population will experience water scarcity. An initiative was launched to discover an efficient technique for producing water through the process of distillation, utilizing clean solar energy, with the objective of optimizing efficiency and sustainability. Desalination, the process of extracting salts and minerals from saltwater, has been employed to tackle this problem. Desalination methods encompass both thermal and non-thermal processes. Non-traditional thermal techniques such as Solar Distillation (SD) are currently under investigation, whereas traditional non-thermal techniques like Reverse Osmosis (RO) are already accessible for commercial use. Solar desalination (SD) is a process that utilizes solar energy to evaporate saline water in enclosed basins. The resulting vapor is then condensed, and the pure water is collected. Basin-type solar stills, which harness solar energy for desalination, provide a practical solution in water-scarce rural and desert regions where the need for drinkable water has risen due to rapid population growth and industrialization. The researcher’s primary objective was to enhance the efficiency of passive solar stills, particularly in regions lacking access to electricity. One of the primary research goals was to improve water productivity in solar stills. These solar stills are easily obtainable and reasonably priced, while also meeting the standards for water quality. The utilization of energy storing materials leads to a daily augmentation in water production, with the overall yield being impacted by the surrounding temperature and the depth of water. Solar stills offer a sustainable method for obtaining fresh water, but additional research and innovation are required to improve water productivity and encourage their widespread use. This conclusion is derived from the findings documented by Alheefi [1]. Solar stills are uncomplicated apparatuses that harness solar energy to vaporize salty water and subsequently convert it into fresh water through condensation. Currently, the majority of fresh water production methods through desalination rely heavily on fossil fuels. The objective was to investigate the influence of input variables, such as solar radiation, ambient temperature, water depth, and insulation thickness, on the efficiency of solar stills and their effect on water productivity. A research study was conducted by Rejeb et al. [2]. Researchers conducted an experimental study, led by Ling et al. [3], to desalinate water using reverse osmosis technology and harnessing tidal energy. Solar-powered desalination systems are acknowledged as a cost-effective and environmentally friendly method for producing potable water from saline sources. The researcher’s study also mentioned the utilization of solar stills in solar-powered water desalination systems, as discussed by Ghanim [4]. The solar still comprises a glass cover, an absorption plate for capturing solar radiation, a layer of water to be distilled, and an insulator that encloses the absorption plate. Rufuss et al. [5] investigated how various factors, such as water depth, glass angle, basin area, material type, insulation, flow rate, motion, water type, and plate characteristics, affect the performance of solar stills. The productivity of solar stills has been significantly enhanced through the reduction of heat loss to a minimum, cooling of the cover, and augmentation of insulation thickness. The various types of solar stills mentioned are: Single slope basin, Double-basin, Multi-basin, Rotating shaft, Hybrid, Wick and multi-wick type, Basin solar still with reflectors, and PV/T solar stills. The calculation of solar stills involves the careful consideration of sun angles and surface azimuth angles, as demonstrated in the studies conducted by Mousavi et al. [6] and Tiwari et al. [7]. An experimental and theoretical analysis was conducted on three single-trough solar stills that had a double slope design and varying glass cover angles. According to Dubey et al. [8], the findings suggest that productivity rises as the angle of the glass cover gets closer to the latitude value of the work site. Passive solar stills use solar energy to convert contaminated or saline water into pure water through evaporation and condensation. On the other hand, active solar stills employ mechanical or electrical systems to improve the evaporation and condensation process and increase water productivity. To improve water productivity in solar stills, a solution was found by combining the passive solar still with an active solar still through a straightforward technical modification. A study presented a novel design that incorporates a basic distillation apparatus within a cylindrical solar collector. A pre-heater system was simulated, and the results were compared to prior studies. The distillate amounts and instantaneous efficiencies were increased by approximately 31% and 40–80% respectively for passive and active targets. Bait and Si-Ameur conducted the research [9]. A study was conducted by researchers, including Easa et al. [10], to address the issue of limited availability of drinkable water. The researchers utilized a solar desalination unit that operates on the principle of humidification-dehumidification (HDH) and incorporates a high-speed rotary humidifier. Increased spraying velocity led to accelerated evaporation. The daily water production reached its maximum at approximately 17 kg when using a slot bore of 0.001 m and a spraying speed of 1200 rpm. A solar system comprising a passive solar water heater (SWH) and an active solar desalination plant for seawater (SW) was the subject of an experimental study. Koibakova et al. [11] combined these two installations into one solar system, resulting in a higher production of distillation outputs in SW. In light of recent research on effective water desalination techniques, multiple studies have been carried out to improve and maximize the efficiency of solar stills, specifically those in the shape of pyramids. A comparative study was conducted to evaluate three different configurations of solar stills: single slope, double slope, and pyramid-shaped covers. The pyramid solar still demonstrated the highest level of productivity. The pyramid solar still’s high productivity can be attributed to its capacity to receive additional and more concentrated sunlight, as well as its smaller volume. This was exemplified in an empirical investigation carried out by Ahmed et al. [12]. Research has focused on using pyramid stills due to the substantial influx of solar radiation into the solar still chamber. Moreover, the larger surface area of the pyramidal cover, in comparison to mono-tilted and bi-tilted solar stills, has played a role in this. Nevertheless, it has been noted that the functioning of pyramid stills is accompanied by heat dissipation caused by the enlarged glass surface area. There are two models of solar stills available: one with a double slope and another with a pyramid design. The pyramid solar still exhibited a marginally greater output, as observed in the study conducted by da Silva et al. [13]. An investigation was conducted on a new design of a multi-side-stepped square pyramid solar still featuring a four-side glass cover. The new design attained water yields of 2.035 liters per square meter on sunny days, as documented by Beik et al. [14]. Shareef et al. [15] conducted an experiment using basin absorber plates with slices along and a W-shaped glass cover. The results showed a 20% increase in distilled water production compared to using a standard absorber plate and pyramid glass cover. Singh et al. [16] discovered that using an octagonal glass cover for the solar still led to higher productivity compared to using a single-slope cover. Fadhil et al. [17] conducted a study that found a decrease in water production as the depth of the water in the basin increased. The study conducted by Nagarajan et al. [18] analyzed the triangular pyramidal solar still in order to enhance the productivity of distilled water. An analysis was conducted on the airflow directed over the upper surface of the glass to cool the glass cover and improve the production of fresh water. The yield improved by 104% when the wind speed increased from 0.5 to 4 meters per second. Sathyamurthy et al. [19] performed an empirical investigation on a solar still in the shape of a triangular pyramid. The study demonstrated that the utilization of paraffin wax resulted in an augmentation of freshwater generation. In a study conducted by Arunkumar et al. [20], the average daily efficiency of the still was found to be 15%. When a boosting mirror was used, the still yielded 2.9 L/m2 a day, compared to 1.52 L/m2 a day without the mirror. Multiple research studies have investigated the impact of photovoltaic solar cells on enhancing the effectiveness of solar stills, specifically pyramidal solar stills. These studies investigate different techniques, such as utilizing a fan to create an air current or employing a heater to heat water, among other methods. A study was conducted on a pyramid-shaped solar still to examine the impact of forced convection on the performance of the distillation device. The research conducted by Taamneh et al. [21] improved the rate of evaporation and resulted in an increase in the daily production of fresh water. A prior study was conducted that involved an exergy analysis on a tubular solar collector-assisted solar still for the purpose of desalinating saline water. The passive system exhibited an hourly exergy efficiency of 7% and a global exergy efficiency of 30%, whereas the active system demonstrated efficiencies of 11% and 41%, respectively. The simple solar still had a lower cost of distilled water (0.018 $/L) compared to the improved still (0.036 $/L). This research was conducted by Bait [22]. A study conducted by researchers Easa et al. [23] has shown that Stirling water dispensers provide a practical solution for producing desalinated water. They harness waste heat generated by the friction of pistons using water jackets, thereby enhancing energy efficiency. The impact of a fan placed inside the pyramidal solar still chamber on its energy efficiency performance was assessed using exergy analysis. The study conducted by Kianifar et al. [24] demonstrated an increase in exergy efficiency. Alawee et al. [25] developed a solar storage unit that consisted of four rotating cylinders incorporated into a pyramidal distillation basin, along with three electric heaters to increase water temperature. Research conducted by Nayi et al. [26] has determined that pyramid solar stills are more efficient and cost-effective when compared to conventional single slope single basin stills. An experiment was conducted to evaluate the performance of a solar still equipped with a rotating black jute belt, using various stopping times, as previously experimented by Abdullah et al. [27]. To effectively improve the water productivity of solar distillers in arid and semi-arid climates, field projects utilized a solar energy device equipped with a mirror. The proposed system successfully increased water production from 7.5 (L/day) to 24 (L/day), as evidenced by Mehdi Soltanian et al. [28]. The rotating components, including the fan, rotating wick, shaft, drum, and discs, disrupt the surface tension of the water, expand the area available for evaporation, and enhance the performance of distillation. Introducing rotating components has been discovered to result in substantial enhancements in the daily output of water. It is advisable to continue exploring solar stills with rotating components as a highly effective and cost-efficient method of supplying purified drinking water. Inducing turbulence inside the solar still chamber improves the efficiency of the evaporation and condensation process. Kabeel et al. [29] performed an empirical investigation to improve the efficiency of a basin-type solar still by incorporating a turbulence system powered by photovoltaic energy. Two solar stills were evaluated: one with a traditional design and another with a modified configuration featuring a vertically-mounted rotating propeller. The findings demonstrated that elevating the rotational velocity of the fan resulted in enhanced daily output, with a notable 25%. Additional empirical investigations were carried out to assess the efficacy of solar stills. These studies revealed that various factors influence their efficiency, including solar radiation, wind velocity, external temperature, water inflow rate, and the angle at which the glass cover is inclined. This research was carried out by Hichem [30].
This study presents a new method that involves adding a rotational mechanism to the pyramidal solar still in order to improve the evaporation and condensation processes. The conventional pyramidal solar distiller encounters challenges such as energy inefficiency, shading effects, and inadequate heating of saline water. Prior research employed solar reflectors and water heating systems, resulting in additional expenses. This study tackles these challenges by incorporating rotational speed into the design. By utilizing a direct current motor powered by a photovoltaic cell, the entire pyramid structure can be rotated to increase solar radiation gain. This, in turn, enhances the efficiency of evaporation and condensation processes. The act of rotating generates turbulence, which enhances the transfer of heat and augments the production of distilled water. It has been noted that rotation also contributes to the cooling of the edges and top of the glass pyramid, offering additional proof of its cooling impact. Furthermore, as the rotational velocity increases, the solar beam’s amplification is greater in comparison to slower rotational speeds, even when there is no rotation. A photovoltaic panel powers a DC motor, allowing it to rotate in a clockwise direction via a rotating shaft. The rotation led to heightened exposure to solar radiation, leading to an increase in solar radiation gain. The design of the flat absorption plate remained unaltered. The research study aims to examine the efficacy of a pyramidal solar still, both with and without rotation, in terms of performance. The primary objectives are to assess the impact of rotational velocity on the thermal efficiency and freshwater output of the system. The rotation speed is the dependent variable, while the independent variables consist of solar radiation intensity, wind speed, external temperatures, and inlet water temperature. The thermal efficiency of the solar still was determined for various rotational speeds (0.125, 0.25, 1, and 1.5 rpm) based on experimental data that was collected. Economic analysis demonstrates that costs can be reduced by eliminating the requirement for extensive reflectors and heating systems. The study examines the efficiency of the pyramidal solar still with and without rotation, assessing the impact of rotation speed on thermal efficiency and freshwater output. The research was carried out at the University of Science and Technology in Tehran from May to July 2023. The quantity of purified water accumulated at regular one-hour intervals was documented using a receptacle specifically designed for collecting water. The findings illustrate the advantages of incorporating rotational motion to improve the efficiency of the solar still and overcome its limitations.
2 Experimental Setup and Test Procedure
Details of the experimental setup are shown in Fig. 1. The design is a pyramidal solar still that operates both in rotating state (PSSR) and non-rotating state (PSS without R).
Figure 1: Details of the experimental setup: (a) pyramidal part, (b) complete system
1- Solar still operation: The solar still was operated from 9 am to 4 pm, using various rotational speeds (0.125, 0.25, 1, and 1.5 rpm) as well as no rotation, in order to assess their effect on performance. The data was recorded at hourly intervals.
2- The variables that affected the performance of the distillation apparatus were documented and assessed within the designated timeframe. The factors encompass solar radiation, wind speed, external temperatures, and rotation speed.
3- The design of the pyramidal solar still involves considering factors such as the surface area that is exposed to solar radiation. The dimensions of the design include an absorption plate that measures 70 * 70 cm, with sides that are 9 cm high in order to minimize the impact of sun shadows. This enhances the assimilation of solar radiation. The water basin is constructed from galvanized iron that is 1.5 mm in thickness, while the pyramid cover is composed of four transparent glass surfaces that are 4 mm thick. The solar still’s feeding system comprises a diminutive funnel that is linked to a buoyant water float located within the tank. This configuration is employed to control the water level and compensate for any reduction during tests. The still had external dimensions of 78 cm × 78 cm × 14 cm, with a wooden insulator that was 1.6 cm thick. The gap between the absorption plate and the wooden insulator was filled with multiple layers of fiberglass material. The table was designed with slightly larger dimensions to allow for the rotation of the picker, particularly at the corners. The feeding system, data logger, and fresh water collection jug are affixed to the outer periphery of the still. The brine tank was covered with transparent Plexi material to enhance the absorption of solar radiation and enhance the heating of the feedwater. Pure water was obtained via a dedicated conduit. The experiments utilized water from the Caspian Sea. The test-rig is furnished with rotating wheels and linked to a rotating shaft propelled by a (6 W) DC motor. A photovoltaic solar panel was employed to obviate the necessity for conventional energy sources. The solar still is equipped with a metal rod that connects the motor and the base, and has two ball bearings attached to it. The pyramid-shaped solar still adheres closely to the dimensions and specifications of previous research designs, including a glass cover tilt angle that is aligned with the local latitude.
4- Evaluating performance and productivity: The solar distiller’s performance was assessed by measuring its daily thermal efficiency and rate of distilled water productivity.
5- Analysis of data.
6- The results were evaluated and contrasted with the objectives and principles set forth in prior articles.
The specificities of the experimental configuration are illustrated in schematic Fig. 2.
Figure 2: A schematic diagram of the experimental test-rig for the improved pyramidal solar still
2.2 Work Layout: Experimental Procedures
The experiments were conducted at the University of Science and Technology in Tehran, at a latitude of 35.44° north and longitude of 51.41° east. The effect of the performance of the pyramidal solar still without rotation was first studied. Then the effect of adding different rotational speeds on performance was tested. Thermal performance of the system at different rotational speeds (0.125, 0.25, 1, and 1.5 rpm) were calculated using the collected experimental data under these conditions. The thermal performance of the solar still was also extracted for fixed mode operation (without rotation). The tests were conducted for three months (May, June, July) on clear days in the year 2023. The freshwater productivity was measured on an hourly basis and collected using a distilled water measuring jar to report the accumulated daily productivity of the solar still. During the experiment, the surface level of the saltwater in the solar still basin was maintained at a fixed depth of 2.5 cm.
The pyramidal solar still utilized type K thermocouples. Thermocouples can be installed in various locations, such as the interior and exterior glass surfaces, entrained air inside the distillation chamber, brine in the absorption plate, absorption plate, thermal insulation, and the salt water feed tank. Pre-calibrated thermocouples were linked to a 12-channel digital thermometer. In addition, wind speed (V) and outside air temperature (Tamb.Tamb.) were measured. The measurement of the total solar radiation (I(t)) was also documented. The amount of purified water obtained every hour was measured at the solar still’s outlet and then transferred to the water collection container. The instrumentation comprises a data logger, an anemometer, an air thermometer, a solar meter, and a storage unit for collecting distilled water.
The minimum error (uncertainty) observed in an instrument is equal to the ratio between its lowest value and the minimum output measurement. The data uncertainty of the experiments was estimated using the method proposed by Holman [31]. Assuming that R is a function of “n” independent parameters, can write the statement as follows:
R=R(X1,X2,X3…,Xn)R=R(X1,X2,X3…,Xn)(1)
In this method, we represent the uncertainty of the experimental devices results using WRWR, while the uncertainties of the parameters are denoted as W1W1, W2W2, W3W3,..., Wn. W1W1, W2W2, W3W3,..., WnWn: They represent the errors or uncertainties associated with each independent parameter X1X1, X2X2, X3X3,..., XnXn. In Eq. (2), each partial derivative (∂R/(∂Xn)∂R/(∂Xn)) is multiplied by the error of the corresponding parameter and squared. Then, the sum of squares of these terms is calculated, and the square root is taken to obtain the overall uncertainty of R. They are represented as X1X1, X2X2, X3X3,..., XnXn in the equation. Accordingly, the estimation of WRWR is determined as follows:
WR=[(∂R∂X1×W1)2+(∂R∂X2×W2)2+…+(∂R∂Xn×Wn)2]12WR=[(∂R∂X1×W1)2+(∂R∂X2×W2)2+…+(∂R∂Xn×Wn)2]12(2)
The evaluation of daily efficiency, denoted as ηdηd, where ˙m is (hourly distillate)˙m is (hourly distillate) hfghfg, is (vaporization latent heat) AsystemAsystem (system area) and I(t) (daily average solar radiation) is performed using the following equation:
ηth=Σ˙m×hfgΣA×I(t)+MotorPowerηth=Σ˙m×hfgΣA×I(t)+MotorPower(3)
Both A, hfghfg and PMPM are constants, so:
ηth=f(˙m,IR,ΔTw−g)ηth=f(˙m,IR,ΔTw−g)(4)
where ˙m˙m is the hourly water productivity, ΔT(w−g)ΔT(w−g) represents the temperature difference between the water inside the absorption plate basin of the solar still and the temperature of the glass cover. The uncertainties of the instruments are presented in Table 1. Furthermore, the minimum error can be defined as the least count divided by the minimum value of the output parameter. Consequently, the productivity is influenced by the water depth inside the flasks, expressed as m=f(h)m=f(h), where h represents the height of water in the flask. Eq. (5) is used to calculate the uncertainty in the water production rate (WmWm) based on the uncertainty in measuring the water depth (WhWh) and the ability to sense changes and impacts of the water production rate to changes in water depth (∂m/∂h1∂m/∂h1). Hence, the uncertainty of the productivity can be determined as follows:
Wm=[(∂m∂h1×Wh)2]12Wm=[(∂m∂h1×Wh)2]12(5)
From Eq. (2), the uncertainty associated with efficiency is given by the following expression:
Wηth=[(∂ηth∂m×Wm)2+(∂ηth∂IR×WI(t))2+(∂ηth∂ΔTw−g)2]12Wηth=[(∂ηth∂m×Wm)2+(∂ηth∂IR×WI(t))2+(∂ηth∂ΔTw−g)2]12(6)
Consequently, the deviation in the daily distillate and efficiency amounts to approximately ±1.4% and ±3%, respectively.
Table 1 presents a comprehensive list of all measured values of the parameters tested, along with the uncertainties and errors that emerged due to the test environment and measurement devices throughout the testing process.
To improve the reliability of the experimental results, we conducted multiple experiments under both rotating and non-rotating conditions. A rotational velocity of 0.25 revolutions per minute was deliberately selected. Error bars were computed for a time span of four days using the chosen velocity. The highest error rate achieved did not surpass 4%, as indicated in Fig. 3.
Figure 3: Error bars for temperatures absorber plate of four days at rotational speed of 0.25 rpm
2.5 Thermal Efficiency of Pyramid Solar Still
The effect of rotational speed on daily efficiency can be described as follows:
The daily efficiency, denoted as ηdηd, is calculated by summing up the hourly condensate production (m), multiplied by the latent heat of vaporization (hfghfg), and dividing it by the average daily solar radiation (I(t)) across the entire area (A) of the device, as given by Kabeel et al. [29]. This calculation is given by:
ηd=∑˙m×hfgA.×I(t)×100% ηd=∑˙m×hfgA.×I(t)×100% (7)
In a conventional solar still, the entire area is considered to be the absorber projected area. However, in a solar still with rotation, the total area is determined by combining the absorber projected area with the projected area of the PV cell. The equation provided below represents the daily thermal efficiency of a distillation device with rotation, as referenced in sources, by Tiwari [32].
ηd(daily thermal efficiency)=∑˙m(hourly distillate)×hfg(vaporization latent heat)Asystem(system area)×I(t)(daily average solar radiation)+Motor Power×100(8)
A (System area)=solar still projected area (Still area) is the sum.(Solarstillprojectedarea+PVprojectedarea)(9)
Daily productivity rise, by Alawee et al. [25],
%=(dailypr.ofPSSR)−(dailypr.ofPSS without R))/(daily pr.of PSS without R)×100(10)
Besides, hfgis calculated based on the water temperature (Tw), by Emad et al. [33] as follows:
hfg=103×[2501.9−2.40706×Tw+1.192217×10−3×T2w−1.5863×10−5×T3w](11)
According to the data presented in Table 2, the highest daily thermal efficiency was attained at a rotation speed of 1 rpm. The subsequent highest efficiency was achieved at a velocity of 1.5 revolutions per minute. Nevertheless, the effectiveness diminishes at this velocity as a result of water droplets descending into the salt water reservoir. The thermal efficiency experiences the greatest decline at a rotational speed of 0.25 revolutions per minute (rpm), and is even lower when there is no rotation. The documented thermal efficiency of the solar still at different rotation speeds, specifically 1, 1.5, 0.25, 0.125 rpm, and no rotation, are 46.2%, 44.2%, 37.8%, 35.3%, and 36.6%, respectively. The variations in the mean daily thermal efficiency of pyramidal solar stills under various operating conditions are displayed in Table 2. The experimental results indicated that the speeds of 0.125 rpm and 0.25 rpm were found to be sluggish and ineffective in terms of productivity and solar radiation absorption. However, a speed of 1.5 revolutions per minute (rpm) had disadvantages, such as rapid droplet descent from the condenser glass surface into the saltwater basin and inability to flow into the distillation water collection path. Furthermore, there were unanticipated water waves present within the saltwater basin. The findings demonstrated that the distiller with rotation yielded a higher volume of fresh water distillate compared to the still without rotation (reference). A selection of data from five consecutive days was made for the purpose of comparison, specifically focusing on solar radiation, wind speed, and outside temperatures, which were found to be almost identical.
As the work date neared, specifically between mid-July and early August, the solar arc approached the point where sunlight reflected off the glass cover of the solar still at a zero angle. During this time, there was an increase in solar radiation due to the glass cover angle being specifically set to align with the latitude of the experimental work area. As a result, the reflection of light from the glass surface decreased, which led to more sunlight being able to pass through into the solar still chamber. The absence of rotation and low speeds were the most similar to the conditions of this time period. However, a speed of 1 rpm resulted in the highest level of productivity, surpassing slower speeds and even the absence of rotation. Although it is located at a greater distance from the time period characterized by intense solar radiation and has not achieved a complete angle of sunlight reflection. Fig. 4 illustrates that solar radiation intensity was highest in the stationary case, followed by the rotating case at a speed of 0.125 rpm, then 0.25 rpm, and lowest in the rotating case at 1 rpm.
Figure 4: Solar radiation at the test location
Table 3 demonstrates that the pure water productivity experiences a 30.2% increase when rotating at a speed of 1 rpm compared to no rotation. Similarly, rotating at a speed of 0.25 rpm yields an 8% increase in pure water productivity compared to no rotation. It is important to understand that the abbreviation “St.” is short for “Static” and refers to a “PSS without R”.
The Fig. 5 indicates that as the ambient temperature decreases, it has a noticeable effect on the glass temperature, causing it to decrease as well. Furthermore, the rotation combined with the temperature difference between the water and the glass, in comparison to the static condition, enhances the condensation process. Consequently, the rotation condition exhibits higher productivity compared to the non-rotation condition.
Figure 5: The ambient temperature at the test location
Fig. 6 displays the ambient wind speeds for the five cases, which exert a notable influence on the cooling of the glass. It was noted that in the case of non-rotation, the wind speed was higher compared to the cases of rotation. Nevertheless, it was observed that the side that experienced the greatest impact from the wind in this situation was the side that faced away from the direction of the wind. This observation suggests that the process of rotation contributes to the cooling of the edges and top of the glass pyramid, thereby providing additional evidence of its cooling effect.
Figure 6: Wind speed at test location
Fig. 7 demonstrates that a pyramidal solar still rotating at a speed of 1 rpm exhibits the greatest temperature variations per hour compared to slower speeds and when not rotating. This is because the droplets slide faster into the pure water collection stream, resulting in an increased impetus for the condensation process. As a result, the process of condensation between the water and the glass surface becomes more efficient. The vapor achieves enhanced contact with the glass cover, resulting in a more pronounced impact of the external air on the glass surfaces. These factors contribute to the highest productivity rate in comparison to the other four cases. The main cause of this phenomenon is the rise in solar radiation absorption and transmission through the glass.
Figure 7: Distilled water at the test location
Fig. 8 depicts the total production rate of solar still cases over time. The solar still’s production rates were as follows: 3.571 liters per day in the stationary state, 3.612 liters per day at a rotation speed of 0.125 rpm, 3.857 liters per day at a rotation speed of 0.25 rpm, 4.530 liters per day at a rotation speed of 1.5 rpm, and 4.653 liters per day in the rotating state 1 rpm. The pyramidal solar still rotating at a speed of 1 rpm achieved the highest production rate. Significantly, the production of the non-rotating solar still decreases after 15:00 in comparison to the rotating states. This text emphasizes the significance of rotation in overcoming solar shade and its direct influence on production. Productivity initially rises until 15:00 at a rate of 1 revolution per minute, and then declines in the absence of rotate.
Figure 8: Accumulated quantity of distilled water
Fig. 9 demonstrates that the temperature of the aquarium was solely elevated by direct exposure to sunlight. The basin temperatures for the five cases exhibited best performance with respect to rotational speed, particularly during the morning hours until noon and from noon until the end of the workday. This suggests that there is a levation in the amount of solar radiation received when the solar radiation is angled, resulting in a shadow being cast from the walls of the solar still onto the absorber plate. At noon, solar radiation is nearly perpendicular and approximately uniform in the five scenarios.
Figure 9: The basin temperatures for the five cases
Fig. 10 clearly demonstrates that the water temperatures achieved at rotational speeds of 1 rpm and 1.5 rpm show superior performance. This phenomenon can be ascribed to the enhanced heat transfer occurring between the absorber plate and the layer of water. The rotation causes a compelled motion within the water, thereby disturbing the surface connections between water molecules. Furthermore, it was noted that as the speed increased, there was improved heat transfer between the absorption plate and the water. Additionally, the presence of bubbles, which hindered heat transfer when there was no rotation, was successfully overcome.
Figure 10: The basin water temperatures for the five cases
4 Assessing Pyramidal Solar Still Costs
The cost estimation for various components used in the current solar distillers is dependent on the type of distiller and its individual components, as indicated in Table 4.
The fixed cost of the reference distiller is F = 85 per square meter (m2). In order to determine the average cost of production, we make the assumption that:
C=F+V(12)
where the variable cost is V, and the annual total cost is C. According to Abdullah et al. [27], let the annual variable cost equals 0.3 F per year, and the variable cost includes the cost of maintenance. Let the expected distiller life time be 10 years, then C = 85 + (0.3 × 85 × 10) = 340. Average daily productivity is about 3.57 (L/m2 a day), and that the distiller operates for 300 days a year, where the sun rises most days of the year in Iran with the distiller life at 10 years. The production during the life of the distiller is 3.57 × 300 × 10 = 10710 L. The cost of 1 L from a reference distiller = (340/(10710)) = 0.0317.
The fixed cost of rotating pyramid solar still for (1 rpm) per 1 (m2) is about F = 105. Then C = 105 + (0.3 × 105 × 10) = 420. Average daily productivity is about 4.65 (L/m2 a day), and that the distiller operates for 300 days a year, where the sun rises most days of the year in Iran with the distiller life at 10 years. The production during the life of the distiller is 4.65 × 300 × 10 = 13950 L. The cost of 1 (L) from a rotation distiller (420/(13950)) = 0.03.
Table 4 presents the cost estimation for various components utilized in the current distillation system. The payback period of the system is determined by various factors, including fabrication costs, maintenance expenses, operating costs, and the cost of feed water. The estimated price for one liter of distilled water is $0.357 by Abdullah et al. [27], which corresponds to the current market rate for water in the local market.
The cost of a pyramid solar still (in dollars) for a PSS without R.
The cost of fabrication is $85.
The daily maintenance cost is $0.01.
The daily cost of distillate for PSS without R is calculated by multiplying the daily productivity by the cost of water per liter, which equals 3.5× multiplied by 0.357, resulting in $1.25.
The daily cost of water production is $1.25.
The net profit per day is calculated by subtracting the maintenance cost from the cost of water produced, which equals $1.25–$0.01 = $1.24.
The payback period is calculated by dividing the fabrication cost by the net profit per day. In this case, the payback period is 69 days, with a fabrication cost of 85 and a net profit per day = $1.24.
The cost of a pyramid solar still for the PSSR is given in dollars.
The cost of fabrication is $105.
The daily maintenance cost is $0.015.
The daily cost of distillate for PSSR at a speed of 1 rpm is calculated as 4.5 multiplied by 0.357, resulting in a cost of $1.61.
The daily cost of water production is $1.61.
The net profit per day is calculated by subtracting the maintenance cost from the cost of water produced, resulting in a value of $1.595.
The payback period is calculated by dividing the fabrication cost by the net profit per day, which results in 66 days.
The study found that the cost of distilled water for the traditional PSS without R system is $0.0317, and its payback period is 69 days. However, in the case of the PSSR, the price of distilled water is decreased to $0.03, and the projected time it takes to recoup the investment is 66 days.
6 Comparative Analysis of the Current Study and Previous Research
The findings of the present study were compared to those of prior studies documented in the literature in order to ascertain the degree of enhancement attained with the PSSR. The comparative findings are displayed in Table 5, emphasizing the originality and efficacy of the implemented alterations.
This comparative analysis distinguishes itself by introducing the novel concept of pyramidal solar stills, leading to enhanced thermal efficiency. The current research further validates the enhancement of solar radiation absorption and reduction of heat loss, which has played a significant role in the advancement of effective solar thermal systems. Hence, the outcomes will bolster ongoing advancements in the domain of pyramidal solar stills, facilitating the more efficient and sustainable utilization of solar stills. Prior research has primarily concentrated on enhancing the evaporation or condensation processes, incorporating supplementary heating systems, and refining engineering designs to optimize different aspects of the pyramidal solar still’s performance. The study’s novelty lies in improving the geometric design of pyramidal solar stills to maximize solar radiation capture, leading to higher energy production and enhanced overall efficiency. An innovative aspect introduced in this study is the complete rotation of the solar still, which includes both the glass cover and the base of the still, at different speeds. This novel methodology resulted in significant enhancements in thermal efficiency, improved thermal performance, and increased productivity of drinkable water. This has a significant influence on the widespread adoption of solar thermal technologies. This experimental study introduced a new method by adding a rotational mechanism to the pyramidal solar still. This mechanism improves the evaporation and condensation processes, resulting in increased overall efficiency and productivity. This was accomplished by creating turbulent motion within the water layer on the absorption plate. In addition, the augmentation of the rotation speed resulted in a greater acquisition of solar radiation in comparison to slower rotation speeds and the absence of rotation, leading to an enhanced transfer of heat and consequently improving the evaporation process. The condensation process demonstrated enhancement as well. It was facilitated the collision of water vapor with the inner surface of the glass. The rotation also aided the downward movement of water droplets, preventing them from combining into larger drops and allowing them to smoothly slide towards the path where clear water is collected. In addition, rotation played a role in the cooling of the glass cover, edges, and top of the glass pyramid. Due to the exposure of all surfaces of the pyramid solar still to external air currents, they underwent amplified cooling effects. As a result, the combination of evaporation and condensation led to a higher production of distilled water. The results indicate a substantial 30.2% boost in productivity when compared to the non-rotation condition. The results obtained were when the rotation speed was set at the best value of 1 revolution per minute (rpm). In addition, the solar still achieved an impressive thermal efficiency of 46.2% when operating at a rotation speed of 1 rpm. These findings enhance the comprehension of a cutting-edge method for creating sustainable water desalination and purification systems. Rotation causes forced movement and turbulence in the water, which disturbs the surface bonds between water molecules and enhances heat transfer. By increasing speeds, the obstacle of bubbles formed in the absence of rotation, was overcome, resulting in a further improvement in heat transfer. Enhancing the rotational velocity resulted in enhanced heat transfer between the absorption plate and water. The observed effect occurred at 2 PM, when the temperature rose to 79.1°C, while the rotation speed was 1.5 revolutions per minute. In contrast, in the absence of rotation, the temperature steadily decreased until it reached its minimum value of 78°C, coinciding with higher solar radiation intensity in the non-rotating condition. The productivity of the solar pyramid distiller increased by 30.2% when it was in the rotating state at a speed of 1 rpm, compared to when it was in the non-rotating state. The solar still’s thermal efficiency, when subjected to rotation speeds of 1 rpm, was measured to be 46.2%.
In this study, a conventional solar distiller was used for comparison. The distiller had the same material and an equivalent absorption plate basin area as the one being studied.
Table 6 below was used to compare the experimental findings with those of traditional hierarchical solar distillation devices mentioned in previous articles. The comparison was made with a conventional solar distiller in this study, which had an equivalent absorption plate basin area and was constructed using the same material.
In this study, the traditional solar distillation device used had a base dimension of 70 cm by 70 cm for the absorption plate basin. The structure was fabricated using galvanized iron, which had a thickness of 1.5 millimeters. When there was no circulation, the conventional solar distillation device produced 3.571 liters per square meter per day of distilled water for drinking purposes. When comparing the productivity of potable water, it was observed that the results are relatively similar. The thermal efficiency in the absence of rotation is 36.6%.
1- The study findings demonstrate that the rotary solar still, operating at a rotational speed of 1 rpm, yields a 30.2% increase in productivity compared to the non-rotating pyramidal solar still. This improvement is attributed to the enhanced absorption of solar radiation, which leads to more efficient temperature distribution, evaporation, and distillation. Additionally, the rotary solar still mitigates the negative impact of shade, further contributing to its superior performance. Therefore, water productivity is increased without the requirement of an additional heating process.
2- The thermal efficiency of the rotary solar still at a rotation speed of 1 rpm was 46.2%, which was superior to the cases with rotation speeds of 0.125, 0.25, 1.5 rpm, and no rotation.
3- The mean expense of distilled water for a PSSR system is $0.03, in contrast to $0.0317 for a PSS without R system.
4- The investigation revealed that the water generated by the rotating pyramid solar still is uncontaminated and appropriate for consumption.
5- The system is cost-effective, utilizing a 40-watt photovoltaic panel and a low power DC electric motor.
6- The pyramidal solar still with rotation is ideal for travel and remote areas with limited services that experience a shortage of drinking water, as well as coastal areas.
These findings establish the foundation for future research to improve the production yield of solar desalination systems and enhance the physical processes involved in the rotation of pyramid solar stills. The goal is to increase their efficiency for practical applications and future advancements in the field. The authors put forward several suggestions for future improvements of the rotating pyramid, which encompass:
1- The application of nanofluid techniques and the integration of paint nanoparticles on the absorption plate can improve the heat transfer characteristics of rotating pyramid solar stills. The incorporation of nanoparticles into the saline water in the basin can greatly enhance the efficiency of heat transfer and evaporation. Mathematical modeling and computational fluid dynamics (CFD) simulations can be employed in solar still applications that involve a hierarchical structure and rotation, following the introduction of nanoparticles.
2- Utilizing a v-corrugated absorber plate is an efficient approach to enhance the absorption plate in rotating pyramid solar stills. This design enhances the surface area and minimizes the reflection of solar rays between the v-corrugated surfaces. Consequently, the reduction of heat losses and the maximization of solar radiation gain result in improved overall efficiency.
3- Future research should prioritize investigating the utilization of phase change materials (PCMs) to assess their influence on the efficiency of rotating pyramidal solar stills. More specifically, examining their capacity to enhance rotational movement and facilitate effective energy retention.
4- In order to promote condensation on the inner surface of the glass in the solar still without impeding the process, it is possible to apply a condensate-enhancing coating or a hydrophilic coating. These materials enhance the generation of water droplets on the glass surface and facilitate their uninterrupted and steady movement towards the condensation water collection pathway, preventing them from dropping into the saltwater basin. This greatly improves the efficiency of the condensation process in the solar still.
Acknowledgement: The authors express their gratitude to Babylon Provincial Council (babylonlocal@gmail.com) in Babylon, Iraq, for their support in conducting the present study.
Funding Statement: The authors received no specific funding for this study.
Author Contributions: The authors confirm their contribution to the paper as follows: study conception and design: Hosseinalipour, Asiaei and Baiee; data collection: Baiee; Experimental work: Baiee; analysis and interpretation of results: Baiee; draft manuscript preparation: Baiee. All authors reviewed the results and approved the final version of the manuscript.
Availability of Data and Materials: The data are available when requested.
Conflicts of Interest: The authors declare that they have no conflicts of interest to report regarding the present study.
References
1. Alheefi, T. (2019). Experimental and analytical study of water production of solar still. London: Brunel University. [Google Scholar]
2. Rejeb, O., Yousef, M. S., Ghenai, C., Hassan, H., Bettayeb, M. (2021). Investigation of a solar still behaviour using response surface methodology. Case Studies in Thermal Engineering, 24, 100816. https://doi.org/10.1016/j.csite.2020.100816 [Google Scholar] [CrossRef]
3. Ling, C., Lou, X., Zhong, Y. (2021). An experimental study on a new high-efficient supercharger for seawater reverse osmosis desalination driven directly by tidal energy. Frontiers in Heat and Mass Transfer, 16, 1–6. https://doi.org/10.5098/hmt.16.16 [Google Scholar] [CrossRef]
4. Ghanim, F. M. (2008). Mathematical modeling of A solar STILL (Thesis). Faculty of Engineering, Department of Mechanical Enigineering, University of Khartoum, Sudan. https://core.ac.uk/download/pdf/71674807.pdf [Google Scholar]
5. Rufuss, D. D. W., Iniyan, S., Suganthi, L., Davies, P. (2016). Solar stills: A comprehensive review of designs, performance and material advances. Renewable and Sustainable Energy Reviews, 63, 464–496. https://doi.org/10.1016/j.rser.2016.05.068 [Google Scholar] [CrossRef]
6. Mousavi Maleki, S. A., Hizam, H., Gomes, C. (2017). Estimation of hourly, daily and monthly global solar radiation on inclined surfaces: Models re-visited. Energies, 10(1), 134. https://doi.org/10.3390/en10010134 [Google Scholar] [CrossRef]
7. Tiwari, G., Sahota, L. (2017). Advanced solar-distillation systems: basic principles, thermal modeling, and its application. India: Springer. [Google Scholar]
8. Dubey, M., Mishra, D. R. (2019). Experimental and theroretical evaluation of double slope single basin solar stills: Study of heat and mass transfer. FME Transactions, 47(1), 101–110. https://doi.org/10.5937/fmet1901101D [Google Scholar] [CrossRef]
9. Bait, O., Si-Ameur, M. (2017). Tubular solar-energy collector integration: Performance enhancement of classical distillation unit. Energy, 141, 818–838. https://doi.org/10.1016/j.energy.2017.09.110 [Google Scholar] [CrossRef]
10. Easa, A. S., Mohamed, S. M., Barakat, W. S., Habba, M. I., Kandel, M. G. et al. (2023). Water production from a solar desalination system utilizing a high-speed rotary humidifier. Applied Thermal Engineering, 224, 120150. https://doi.org/10.1016/j.applthermaleng.2023.120150 [Google Scholar] [CrossRef]
11. Koibakova, S., Kenzhetaev, G., Syrlybekkyzy, S., Tarasenko, G., Suleimenova, B. et al. (2021). Experimental studies of the efficiency of a solar system, including a passive water heater and an active seawater distiller. Heliyon, 7(2), 1–8. [Google Scholar]
12. Ahmed, H. M., Alshutal, F. S., Ibrahim, G. (2014). Impact of different configurations on solar still productivity. Journal of Advanced Science and Engineering Research, 3(2), 118–126. [Google Scholar]
13. da Silva, K. S.,de Brito, Y. J. V., Silva, C. B., de Lima, G. G. C., de Medeiros, K. M. et al. (2023). Study of solar still in groundwater treatment in Brazilian northeast. Desalination and Water Treatment, 293, 14–26. https://doi.org/10.5004/dwt [Google Scholar] [CrossRef]
14. Beik, A. J. G., Assari, M. R., Tabrizi, H. B. (2020). Passive and active performance of a multi-side-stepped square pyramid solar still; experimental and modeling. Journal of Energy Storage, 32, 101832. https://doi.org/10.1016/j.est.2020.101832 [Google Scholar] [CrossRef]
15. Shareef, A. S., Kurji, H. J., Hamzah, A. H. (2024). Modifying performance of solar still, by using slices absorber plate and new design of glass cover, experimental and numerical study. Heliyon, 10(1), 1–18. [Google Scholar]
16. Singh, B. K., Ramji, C., Ganeshan, P., Mohanavel, V., Balasundaram, T. et al. (2023). Performance analysis of solar still by using octagonal-pyramid shape in the solar desalination techniques. International Journal of Photoenergy, 2023, 1–9. [Google Scholar]
17. Fadhil, O. T., Abed, W. M. (2024). Experimental investigation on a pyramid solar still under Iraq climate conditions. AIP Conference Proceedings, Anbar-Iraq, AIP Publishing. https://doi.org/10.1063/5.0190411 [Google Scholar] [CrossRef]
18. Nagarajan, P., El-Agouz, S., Arunkumar, T., Sathyamurthy, R. (2016). Theoretical approach on the effect of cover cooling in triangular pyramid solar still. International Journal of Ambient Energy, 6, 1–17. https://doi.org/10.1080/01430750.2016.1159609 [Google Scholar] [CrossRef]
19. Sathyamurthy, R., Nagarajan, P., Kennady, H., Ravikumar, T., Paulson, V. et al. (2014). Enhancing the heat transfer of triangular pyramid solar still using phase change material as storage material. Frontiers in Heat and Mass Transfer, 5(1), 1–5. https://doi.org/10.5098/hmt.5.3 [Google Scholar] [CrossRef]
20. Arunkumar, T., Jayaprakash, R., Prakash, A., Suneesh, P., Karthik, M. (2010). Study of thermo physical properties and an improvement in production of distillate yield in pyramid solar still with boosting mirror. Indian Journal of Science and Technology, 3, 879–884. https://doi.org/10.17485/ijst/2010/v3i8/29901 [Google Scholar] [CrossRef]
21. Taamneh, Y., Taamneh, M. M. (2012). Performance of pyramid-shaped solar still: Experimental study. Desalination, 291, 65–68. https://doi.org/10.1016/j.desal.2012.01.026 [Google Scholar] [CrossRef]
22. Bait, O. (2019). Exergy, environ-economic and economic analyses of a tubular solar water heater assisted solar still. Journal of Cleaner Production, 212, 630–646. https://doi.org/10.1016/j.jclepro.2018.12.015 [Google Scholar] [CrossRef]
23. Easa, A. S., Khalaf-Allah, R. A., Al-Nagdy, A. A., Tolan, M. T., Mohamed, S. M. (2022). Experimental study of saving energy and improving Stirling water dispenser performance using the waste heat of both pistons friction. Applied Thermal Engineering, 213, 118727. https://doi.org/10.1016/j.applthermaleng.2022.118727 [Google Scholar] [CrossRef]
24. Kianifar, A., Heris, S. Z., Mahian, O. (2012). Exergy and economic analysis of a pyramid-shaped solar water purification system: Active and passive cases. Energy, 38(1), 31–36. https://doi.org/10.1016/j.energy.2011.12.046 [Google Scholar] [CrossRef]
25. Alawee, W. H., Mohammed, S. A., Dhahad, H. A., Abdullah, A., Omara, Z. et al. (2021). Improving the performance of pyramid solar still using rotating four cylinders and three electric heaters. Process Safety and Environmental Protection, 148, 950–958. https://doi.org/10.1016/j.psep.2021.02.022 [Google Scholar] [CrossRef]
26. Nayi, K. H., Modi, K. V. (2018). Pyramid solar still: A comprehensive review. Renewable and Sustainable Energy Reviews, 81, 136–148. https://doi.org/10.1016/j.rser.2017.07.004 [Google Scholar] [CrossRef]
27. Abdullah, A., Alarjani, A., Abou Al-sood, M., Omara, Z., Kabeel, A. et al. (2019). Rotating-wick solar still with mended evaporation technics: Experimental approach. Alexandria Engineering Journal, 58(4), 1449–1459. https://doi.org/10.1016/j.aej.2019.11.018 [Google Scholar] [CrossRef]
28. Soltanian, M., Hoseinzadeh, S., Astiaso Garcia, D. (2024). Proposal of a reflector-enhanced solar still concept and its comparison with conventional solar stills. Water, 16(2), 355. https://doi.org/10.3390/w16020355 [Google Scholar] [CrossRef]
29. Kabeel, A., Hamed, M. H., Omara, Z. (2012). Augmentation of the basin type solar still using photovoltaic powered turbulence system. Desalination and Water Treatment, 48(1–3), 182–190. [Google Scholar]
30. Boutebila, H. (1987). A free flow flat plate solar still. UK: Loughborough University. [Google Scholar]
31. Holman, J. (2012). Experimental methods for engineers. New York: McGraw-HiD. Inc. [Google Scholar]
32. Tiwari, G., Tiwari, A. (2016). Handbook of solar energy. India: Springer. [Google Scholar]
33. Ghandourah, E., Panchal, H., Fallatah, O., Ahmed, H. M., Moustafa, E. B. et al. (2022). Performance enhancement and economic analysis of pyramid solar still with corrugated absorber plate and conventional solar still: A case study. Case Studies in Thermal Engineering, 35, 101966. https://doi.org/10.1016/j.csite.2022.101966 [Google Scholar] [CrossRef]
34. Hammoodi, K. A., Dhahad, H. A., Alawee, W. H., Omara, Z. M. (2023). Enhancement of pyramid solar still productivity through wick material and reflective applications in Iraqi conditions. Mathematical Modelling of Engineering Problems, 10(5), 1548–1556. [Google Scholar]
Cite This Article
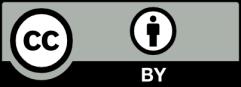
This work is licensed under a Creative Commons Attribution 4.0 International License , which permits unrestricted use, distribution, and reproduction in any medium, provided the original work is properly cited.