Open Access
ARTICLE
Experimental Study on the Bubble Dynamics of Magnetized Water Boiling
1 Low-Carbon Energy Institute, Ludong University, Yantai, 264025, China
2 Key Laboratory of Efficient Utilization of Low and Medium Grade Energy, MOE, School of Mechanical Engineering, Tianjin University, Tianjin, 300350, China
* Corresponding Author: Yang Cao. Email:
(This article belongs to the Special Issue: Innovative Heat Transfer Fluids for Enhanced Energy Sustainability in Thermal Systems)
Frontiers in Heat and Mass Transfer 2024, 22(2), 675-685. https://doi.org/10.32604/fhmt.2024.051208
Received 29 February 2024; Accepted 08 April 2024; Issue published 20 May 2024
Abstract
Boiling heat transfer, as an efficient heat transfer approach, that can absorb a large amount of latent heat during the vaporization, is especially suitable for heat transfer occasions with high heat flux demands. Experimental studies show that the surface tension coefficient of pure water can be reduced sharply (up to 25%) when it is magnetized by a magnetic field applied externally. In this paper, magnetized water (MW) was used as the work fluid to conduct boiling heat transfer experiments, to explore the influence of magnetization on the boiling characteristics of pure water. The electromagnetic device was used to magnetize water, and then the MW was used as the work-fluid of boiling heat transfer experiments, the bubble dynamic behavior of the MW boiling was captured by a video camera, and the characteristics and mechanism were analyzed. It was found that at the same conditions, the boiling of MW can produce more vapor bubbles of smaller size than the water without magnetization, which leads to a higher heat-transfer efficiency. This indicates that magnetization can enhance the boiling heat transfer of pure water. Furthermore, the thermal conditions required by magnetized water when the boiling is started are lower than the non-magnetized water boiling, which means the earlier start of nucleate pool boiling when using the MW.Keywords
With the rapid development of social economics, the international energy situation has been not optimistic. The global annual fossil energy consumption is around 10 billion tons of standard coal, therefore, energy conservation and emission reduction have become an important research direction. In addition to the development of new energy sources, such as nuclear energy, wind energy, and solar energy, traditional energy conservation technologies are also essential, such as improving heat transfer efficiency. Boiling can absorb a large amount of latent heat when the liquid is vaporized, which makes it suitable for heat transfer occasions with high heat flux demands. With the rapid development of high-precision manufacturing technology, especially the use of micro-electromechanical systems (MEMS) and high-performance computing chips, the occasions demanding high heat flux heat transfer become more and more, hence the boiling heat transfer enhancement technology become a hot topic in the current research fields.
Water is the most common work fluid in heat transfer equipment, but its boiling characteristics and mechanism were still not very clear, even if it was one of the hot research topics in the past decades. The common methods for enhancing boiling heat transfer of water can be divided into two categories: active methods [1,2] and passive methods [3] depending on whether or not external power is introduced. Passive methods can be divided into two categories: changing structure or surface characteristics of boiling surface [4,5], this method requires changing the structure of existing equipment; the other is changing the physical properties of work-fluid by adding surfactant or nanoparticles into the work-fluid [6–11], such as the reduction of the surface tension, which causes changes of dynamic behaviors of the boiling bubbles and the variations in the boiling heat transfer characteristics. However, the way of adding surfactant or nanoparticles into the work fluid could lead to some problems [12,13], e.g., the corrosion or/and blockage of the flow channels, so some researchers tried to use the magnetized water as the work-fluid of boiling heat transfer because it was found that the pure water can show some variations in some of its physical properties after being magnetized by an external magnetic field.
Some studies on magnetized water focusing on the changes in its physical properties [14–17] were carried out. Cai et al. [18] found that water molecules after magnetic treatment had lower energy, higher activation energy, and better stability. The average size of water clusters increased, and the formation of hydrogen bonds increased. Huo et al. [19] found that the surface tension of tap water and deionized water decreased after magnetization. Cho et al.’s [20] experiment results showed that after repeated magnetizations, the surface tension of water decreased, and the surface tension of treated water was about 8% lower than untreated water. Pang et al. [21,22] conducted experiments with magnetized water made from ultrasonic degassed water. The results showed that the conductivity of degassed magnetized water was lower, infrared absorption was larger, and surface tension was reduced. He also found that the infrared absorption intensity of magnetized water increased with the increase of the magnetic field, and the intensity of the Raman scattering peak increased greatly. In our previous study [23], the surface tension coefficient of magnetized water was investigated thoroughly under various conditions of magnetic field (MF) intensity and magnetization time. An obvious decrease was found after the magnetization treatment, and the decrease ratio depends on the MF intensity and treatment time. The optimum MF intensity is 300 mT, and a weaker or stronger MF intensity other than 300 mT would weaken the effect of magnetization. There is an optimum treatment duration for each MF intensity, and it gets shorter as the MF intensity increases.
Only a few researchers have explored the effect of magnetization on boiling heat transfer of water. Aminfar et al. [24] investigated the effect of magnetic nanofluid and external magnetic field on CHF of the subcooled flow boiling, and results showed that the CHF of subcooled flow boiling has been improved. Amirzehni’s research [25] showed that the departure diameter of vapor bubbles of the magnetized water is 5–10% smaller than that of non-magnetized water. Other studies related to the magnetization of fluids or Magnetohydrodynamics can also be found [26–29].
In summary, magnetization, as a relatively clean and environmentally friendly technology, has a great influence on the physical properties and boiling heat transfer characteristics of pure water, which shows the feasibility and necessity of magnetized water boiling research. Application of magnetized water as work-fluids to enhance boiling heat transfer might be a practical, reliable, and easy way to enhance the heat transfer, so in this paper, the magnetized water was obtained by an external magnetic field, and the boiling characteristic of the magnetized water was investigated and analyzed.
Magnetization could affect the physical properties of pure water, and its effect on bubble dynamics and boiling heat transfer characteristics is mainly achieved by varying the surface tension of the work fluid (pure water). Therefore, in this paper, the magnetization treatment on the pure water was first carried out by placing the pure water in a uniform and constant magnetic field (MF), as shown in Fig. 1. The pure water was filled in a beaker and then placed in the MF with a constant magnetic intensity generated by a solenoid coil, when the magnetization treatment was finished, the MW was put into the boiling container of the experimental system to conduct the boiling experiments.
Figure 1: The equipment of magnetization treatment
To investigate the MW boiling phenomena and mechanism, a visualization experiment was used to investigate the behavior characteristics of vapor bubbles first, the images of the boiling bubbles were captured by the camera and some important parameters of the bubble dynamics, including the departure frequency, bubble diameter, and nucleate density on the heating surface were obtained. The experimental configuration of MW boiling is shown in Fig. 2 and it was a transparent rectangular container (with the size of 165 mm × 110 mm × 80 mm) divided into 2 tanks by the wall in the middle, the 2 tanks would be filled with MW and non-magnetized water, respectively, so it is convenient to compare the boiling characteristic of the two different kinds of work-fluids. The polyimide (PI) heating films were used as a heating surface to generate the boiling bubble, the films were pasted to the walls and bottom of the container as shown in the figure. A DC power source was used to supply heat to the heating films, and the voltage and current were measured by a multimeter during the running of the boiling experiments, so the heat flux applied to the heating film could be calculated. The images of the MW and non-magnetized water boiling were captured at the same time by a camera.
Figure 2: The basic experimental configuration of MW boiling
The experiment was carried out at room temperature (20°C) and atmosphere pressure. The MW and non-magnetized water were filled into the two tanks, respectively, and heated by a pre-heater to about 85°C. When the temperature of the bulk water in the tanks reached a stable condition, the power of the heating film was turned on, as the increasing of the heating power, an over-heated liquid layer was gradually formed near the heating film, and then the vapor bubbles began to nucleate and grow up on the heating film. Since the heating surfaces of the two tanks are of the same area and belong to the same heating film supplied with a DC power source, the bubble behaviors of MW and non-magnetized water boiling can be investigated and compared at the same conditions except for the work fluids. Since the mechanism of bubble dynamics and heat transfer of magnetized water boiling will be explained by the variation of water surface tension after magnetization, the results of previous experimental studies on the physical properties (surface tension coefficient) of magnetized water were given in the supplementary part.
3.1 The Characteristic of Bubbles on the Bottom Heating Film
The images of MW and non-magnetized water boiling at the moment of onset of nucleate boiling are shown in Fig. 3. The heat flux at this moment is 14.5 kW/m2 for MW boiling and 15.7 kW/m2 for non-magnetized water boiling, respectively, so the heat flux required for the onset of MW nucleate boing is a little lower than that of non-magnetized water, this means that it can make the starting of boiling earlier and easier to use the MW as the work-fluid. It can also be found by comparing the images in Fig. 3 that the bubble density of MW boiling is higher than the non-magnetized water boiling, this verified that the magnetization of water has a positive effect on the bubbles’ generation and boiling heat transfer.
Figure 3: The onset of nucleate boiling
As the increase of the applied heat flux, the number and size of the vapor bubbles gradually increased, because the superheat degree of the heating film was getting higher, this formed more and more evaporation nuclei on the film surface, and the superheat liquid layer around the heating surface becomes thicker and thicker, this also makes the vapor bubbles easier to grow up. The images of MW boiling and non-magnetized water boiling with the same heat flux of about 18 kW/m2 are shown in Fig. 4. Compared with the onset state of boiling shown in Fig. 3, the bubbles in Fig. 4 become more and larger for both the MW boiling and non-magnetized water boiling because the heat flux applied on the heating films is larger. From Fig. 4, it is very obvious that the MW boiling case has much more bubbles than the non-magnetized water boiling even if the applied heat flux is the same, this verifies again that the magnetization has an important effect on the bubble dynamical behaviors and boiling heat transfer characteristic. There are many small size bubbles in Fig. 4a, which are not found in Fig. 4b, this shows that the magnetization leads to the formation of small size bubbles, which can be explained by the surface tension of water being reduced steeply by the magnetization (for more please go to the supplement) and the smaller surface tension tends to form the bubbles of small size.
Figure 4: The vapor bubbles at a larger heat flux
Fig. 5 displayed the bubble images when the boiling bubbles depart from the heating surface regularly, the continuous repeat of the bubble life-cycle, including the nucleation, growth, departure, and collapse can be observed. The boiling process is very vigorous compared with those at the low heat flux conditions as shown in Figs. 3 and 4, both the size and the number of vapor bubbles are larger. By comparing the images in Fig. 5, it can be found that the number of vapor bubbles in MW boiling is still much more than that of non-magnetized water boiling, and the size of vapor bubbles in MW boiling is relatively smaller than that of non-magnetized water boiling, which is also the consequence of reduced surface tension of the magnetized water.
Figure 5: The vapor bubbles with regular departure
3.2 The Characteristic of Bubbles on the Back-Wall Heating Film
The images of vapor bubbles generated on the heating film of the back wall were given in Fig. 6. As mentioned in Section 2, the work fluid container of the experimental apparatus was divided into two tanks to compare the bubble behaviors of the water with and without magnetization, so the right tank was filled with magnetized water at a given condition (For images of Fig. 6, the magnetic field intensity was 300 mT, magnetization time was 15 min), and the left one was filled with non-magnetized pure water for comparison. The heating film is a rectangle-shaped polyimide film pasted on the back wall of the container and the heat was supplied by a DC power. As can be seen in the images, there are more bubbles on the right part of the heating film, and the average size of bubbles on the right is smaller than that of the left one.
Figure 6: Vapor bubbles on the back-wall heating films
To investigate the bubble characteristic and heat transfer feature of the MW boiling quantitatively, the numbers of vapor bubbles generated in the boiling experiments were counted, and the bubble size was also investigated quantitively, the bubble images captured by the camera were processed by using the Image Processing Toolbox of MATLAB, and the average equivalent diameter of vapor bubbles was also obtained. The numbers of vapor bubbles generated in the boiling of the non-magnetized water and 3 kinds of MWs were shown in Fig. 7, the detailed configuration (the intensity of applied magnetized field, the magnetization time) and property (surface tension coefficient) of these 3 MWs were given in Table 1. As can be seen, for all the heat flux conditions, the bubble number of 3 MWs is larger than that of the non-magnetized water boiling at the same heat flux. The boiling of the MW1(300 mT, 15 min) generates the most bubbles because the surface tension of water was reduced most at this magnetization condition (see Fig. S1), the bubble numbers of boiling of MW2 and MW3 are also more than the non-magnetized water boiling, but less than the MW (300 mT 15 min) case, because the surface tension was also reduced by the magnetization at these 2 conditions, but not as severe as that of MW1 (300 mT 15 min). From Fig. 7, it can be known that the more the surface tension of water is reduced, the more bubbles will be generated in the boiling.
Figure 7: Variation of bubbles numbers with heat flux
The average equivalent diameter of vapor bubbles generated in the boiling of the non-magnetized water and 3 kinds of MWs were obtained and shown in Fig. 8, as can be seen in the figure, for both the MW boiling and non-magnetized water boiling, the bubble average equivalent diameter increases as the applied heat flux increases, but the bubble average diameter of 3 MWs boiling is relatively smaller than that of non-magnetized water boiling at the same conditions, and the boiling of MW1 generates the smallest bubbles, the size of bubbles generated by other two MWs (500 mT 10 min and 750 mT 5 min) boiling was also smaller than the non-magnetized water case, but larger than that of MW1, so it can be known that the more the surface tension of water is reduced, the smaller the boiling bubbles will be. These also can be verified by observing the images obtained in the boiling experiments. The Young-Laplace Eq. (1) [30] can be used to explain why the reduced surface tension tends to make the bubble number more and the bubble size smaller.
Figure 8: Variation of bubbles average diameters with heat flux
Pin−Pout=2σr(1)
here, Pin and Pout are the fluid pressure inside and outside of the bubble, respectively, σ is the surface tension coefficient of the work-fluid, and r is the radius of the bubble. When the surface tension coefficient σ is reduced by the magnetization, the pressure Pin needed for generating the vapor bubble is smaller, so it means the easier nucleation of the new bubbles. On the other hand, if the inside and outside pressure, Pin and Pout, are given a fixed value when the surface tension coefficient σ is decreased, the bubble radius r will also be decreased.
In this paper, the boiling heat transfer characteristic of the magnetized water was investigated experimentally for the first time by using electromagnetic equipment. The conclusions obtained are as follows. At the same conditions, the boiling of MW can produce much more vapor bubbles than the water without magnetization, a better-boiling heat transfer rate can be achieved because more latent heat can be taken away from the heating surface by the extra bubbles. Furthermore, the average diameter of bubbles generated in MW boiling is smaller than that of non-magnetized water boiling, and the heat flux required by MW when the boiling is started is lower than that of the non-magnetized water, which means the earlier start of nucleate boiling can be achieved by magnetization. These indicate that magnetization has an obvious effect on the boiling characteristic of the water and an enhancement on the boiling heat transfer rate, and it can be applied in practical engineering heat exchange occasions.
Acknowledgement: The authors are grateful for the support of the Research Starting Program of Ludong University (Gran No. 221/20220045), and the valuable advice from Prof. Tomoaki Kunugi (Professor Emeritus of Kyoto University).
Funding Statement: This research is supported by the Research Starting Program of Ludong University (Gran No. 221/20220045).
Author Contributions: The authors confirm contribution to the paper as follows: study conception and design: Y. Cao; data collection: J. Liu; analysis and interpretation of results: J. Liu; draft manuscript preparation: Y. Cao, X. Meng. All authors reviewed the results and approved the final version of the manuscript.
Availability of Data and Materials: The data that support the findings of this study are available on request from the corresponding author, Y. Cao, upon reasonable request.
Conflicts of Interest: The authors declare that they have no conflicts of interest to report regarding the present study.
References
1. Zonouzi, S. A., Aminfar, H., Mohammadpourfard, M. (2019). A review on effects of magnetic fields and electric fields on boiling heat transfer and CHF. Applied Thermal Engineering, 151, 11–25. https://doi.org/10.1016/j.applthermaleng.2019.01.099 [Google Scholar] [CrossRef]
2. Swarnkar, A., Lakhera, V. J. (2021). Ultrasonic augmentation in pool boiling heat transfer over external surfaces: A review. Proceedings of the Institution of Mechanical Engineers, Part C: Journal of Mechanical Engineering Science, 235(11), 2099–2111. [Google Scholar]
3. Cheng, L. X., Mewes, D., Luke, A. (2007). Boiling phenomena with surfactants and polymeric additives: A state-of-the-art review. International Journal of Heat and Mass Transfer, 50(13–14), 2744–2771. [Google Scholar]
4. Li, W., Dai, R., Zeng, M., Wang, Q. (2020). Review of two types of surface modification on pool boiling enhancement: Passive and active. Renewable and Sustainable Energy Reviews, 130, 109926. [Google Scholar]
5. Liang, G., Mudawar, I. (2019). Review of pool boiling enhancement by surface modification. International Journal of Heat and Mass Transfer, 128, 892–933. https://doi.org/10.1016/j.ijheatmasstransfer.2018.09.026 [Google Scholar] [CrossRef]
6. Khairul, M. A., Doroodchi, E., Azizian, R., Moghtaderi, B. (2017). Advanced applications of tunable ferrofluids in energy systems and energy harvesters: A critical review. Energy Conversion and Management, 149, 660–674. https://doi.org/10.1016/j.enconman.2017.07.064 [Google Scholar] [CrossRef]
7. Yang, Y. M., Maa, J. R. (2001). On the criteria of nucleate pool boiling enhancement by surfactant addition to water. Chemical Engineering Research and Design, 79(4), 409–416. https://doi.org/10.1205/026387601750282337 [Google Scholar] [CrossRef]
8. Mori, S., Utaka, Y. (2017). Critical heat flux enhancement by surface modification in a saturated pool boiling: A review. International Journal of Heat and Mass Transfer, 108, 2534–2557. https://doi.org/10.1016/j.ijheatmasstransfer.2017.01.090 [Google Scholar] [CrossRef]
9. Acharya, A., Pise, A. (2017). A review on augmentation of heat transfer in boiling using surfactants/additives. Heat and Mass Transfer, 53(4), 1457–1477. https://doi.org/10.1007/s00231-016-1905-3 [Google Scholar] [CrossRef]
10. Ciloglu, D., Bolukbasi, A. (2015). A comprehensive review on pool boiling of nanofluids. Applied Thermal Engineering, 84, 45–63. https://doi.org/10.1016/j.applthermaleng.2015.03.063 [Google Scholar] [CrossRef]
11. Fang, X., Chen, Y., Zhang, H., Chen, W., Dong, A. et al. (2016). Heat transfer and critical heat flux of nanofluid boiling: A comprehensive review. Renewable and Sustainable Energy Reviews, 62, 924–940. https://doi.org/10.1016/j.rser.2016.05.047 [Google Scholar] [CrossRef]
12. Moreira, T. A., Moreira, D. C., Ribatski, G. (2018). Nanofluids for heat transfer applications: A review. Journal of the Brazilian Society of Mechanical Sciences and Engineering, 40, 303–332. https://doi.org/10.1007/s40430-018-1225-2 [Google Scholar] [CrossRef]
13. Kamel, M. S., Lezsovits, F., Hussein, A. M., Mahian, O., Wongwises, S. (2018). Latest developments in boiling critical heat flux using nanofluids: A concise review. International Communications in Heat and Mass Transfer, 98, 59–66. https://doi.org/10.1016/j.icheatmasstransfer.2018.08.009 [Google Scholar] [CrossRef]
14. Ghauri, S. A., Ansari, M. S. (2006). Increase of water viscosity under the influence of magnetic field. Journal of Applied Physics, 100(6), 66–101. [Google Scholar]
15. Wang, Y., Wei, H., Li, Z. (2018). Effect of magnetic field on the physical properties of water. Results in Physics, 8, 262–267. https://doi.org/10.1016/j.rinp.2017.12.022 [Google Scholar] [CrossRef]
16. Chibowski, E., Szcześ, A., Hołysz, L. (2018). Influence of magnetic field on evaporation rate and surface tension of water. Colloids and Interfaces, 2(4), 68–82. https://doi.org/10.3390/colloids2040068 [Google Scholar] [CrossRef]
17. Seyfi, A., Afzalzadeh, R., Hajnorouzi, A. (2017). Increase in water evaporation rate with increase in static magnetic field perpendicular to water-air interface. Chemical Engineering and Processing: Process Intensification, 20, 195–200. [Google Scholar]
18. Cai, R., Yang, H., He, J., Zhu, W. (2009). The effects of magnetic fields on water molecular hydrogen bonds. Journal of Molecular Structure, 938(1–3), 15–19. [Google Scholar]
19. Huo, Z. F., Zhao, Q., Zhang, Y. H. (2011). Experimental study on effects of magnetization on surface tension of water. Procedia Engineering, 26, 501–505. https://doi.org/10.1016/j.proeng.2011.11.2198 [Google Scholar] [CrossRef]
20. Cho, Y. I., Lee, S. H. (2005). Reduction in the surface tension of water due to physical water treatment for fouling control in heat exchangers. International Communications in Heat and Mass Transfer, 32(1–2), 1–9. [Google Scholar]
21. Pang, X., Deng, B. (2008). Investigation of changes in properties of water under the action of a magnetic field. Science in China Series G: Physics, Mechanics and Astronomy, 51(11), 1621–1632. https://doi.org/10.1007/s11433-008-0182-7 [Google Scholar] [CrossRef]
22. Pang, X., Deng, B. (2008). The changes of macroscopic features and microscopic structures of water under influence of magnetic field. Physical B: Condensed Matter, 403, 19–20. [Google Scholar]
23. Liu, J. S., Cao, Y. (2021). Experimental study on the surface tension of magnetized water. International Communications in Heat and Mass Transfer, 101, 105091. [Google Scholar]
24. Aminfar, H., Mohammad, M., Maroofiazar, R. (2014). Experimental study on the effect of magnetic field on critical heat flux of ferrofluid flow boiling in a vertical annulus. Experimental Thermal and Fluid Science, 58, 156–169. https://doi.org/10.1016/j.expthermflusci.2014.06.023 [Google Scholar] [CrossRef]
25. Amirzehni, R., Aminfar, H., Mohammad, M. (2017). Experimental study of magnetic field effect on bubble lift-off diameter in sub-cooled flow boiling. Experimental Thermal and Fluid Science, 89, 62–71. https://doi.org/10.1016/j.expthermflusci.2017.07.022 [Google Scholar] [CrossRef]
26. Nidhal, B., Zia, U., Mansoor, A., Cyrus, R. M., Sayed, M. E. (2024). Effect of Joule heating and MHD on periodical analysis of current density and amplitude of heat transfer of electrically conducting fluid along thermally magnetized cylinder. Ain Shams Engineering Journal, 15, 102374. https://doi.org/10.1016/j.asej.2023.102374 [Google Scholar] [CrossRef]
27. Hammad, A. S., Zia, U., Fethi, A., Asifa, I., Mussad, S. A. et al. (2024). Primitive and gravity modulation of periodical heat transfer along magnetic-driven porous cone with thermal conductivity and surface heat flux. Case Studies in Thermal Engineering, 53, 103866. https://doi.org/10.1016/j.csite.2023.103866 [Google Scholar] [CrossRef]
28. Ali, M., Tabrez, M., Liu, Khan, W. A. (2024). Magnetized bioconvective thermal efficacy of ferromagnetic nanoparticles involving appliance of radiation and viscous dissipation. Thermal Science and Engineering Progress, 47, 102314. https://doi.org/10.1016/j.tsep.2023.102314 [Google Scholar] [CrossRef]
29. Muhammad, T., Mehboob, A., Amjad, A. P., Salem, A., Waqar, A. K. et al. (2024). Bioconvection analysis for convectively heated radiative flow of Sutter by fluid involving efficacy of ferromagnetic nanoparticles. Thermal Science and Engineering Progress, 48, 102336. https://doi.org/10.1016/j.tsep.2023.102336 [Google Scholar] [CrossRef]
30. Carey, V. P. (2008). Liquid-vapor phase-change phenomena, 2nd Edition. Oxford, UK: Taylor &Francis Group. [Google Scholar]
Supplementary Materials
The physical properties of magnetized pure water were studied in our previous studies and the research results of surface tension variation after magnetization were already published [23]. Fig. S1 briefly shows the surface tension coefficient of pure water magnetized at various magnetic field intensities and magnetization time, as can be seen, the surface tension was found to be changed obviously after the magnetization treatment, the most severe case occurs at the conditions (Magnetic field intensity 300 mT, magnetization time 15 min), the surface tension coefficient of water dropped about 25% to 54 mN/m from the normal value of 72 mN/m of the pure water. For more information about the surface tension of magnetized water, please refer to the previous study [23].
Figure S1: The variations of water surface tension with magnetization time and magnetic field intensity
Cite This Article
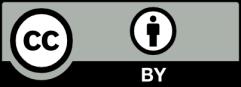
This work is licensed under a Creative Commons Attribution 4.0 International License , which permits unrestricted use, distribution, and reproduction in any medium, provided the original work is properly cited.