Open Access
ARTICLE
Optimizing Sustainability: Exergoenvironmental Analysis of a Multi-Effect Distillation with Thermal Vapor Compression System for Seawater Desalination
1 Laboratory of Biomaterials and Transport Phenomena, University of Medea, Medea, 26000, Algeria
2 Laboratory of Chemical Process Engineering, University of Ferhat Abbas, Setif, 19000, Algeria
3 Department of Environmental Engineering/Laboratoire de Recherche sur le Médicament et le Développement Durable (ReMeDD), University of Salah Boubnider Constantine 3, El Khroub, 25012, Algeria
4 Research Unit on Analysis and Technological Development in Environment (URADTE-CRAPC), Bou-Ismail Tipaza, BP 384, Algeria
5 School of Engineering, Merz Court, Newcastle University, Newcastle upon Tyne, NE1 7RU, UK
6 Univ Rennes, Ecole Nationale Supérieure de Chimie de Rennes, CNRS, ISCR–UMR6226, Rennes, F-35000, France
* Corresponding Authors: Hichem Tahraoui. Email: ; Abdeltif Amrane. Email:
(This article belongs to the Special Issue: Innovative Heat Transfer Fluids for Enhanced Energy Sustainability in Thermal Systems)
Frontiers in Heat and Mass Transfer 2024, 22(2), 455-473. https://doi.org/10.32604/fhmt.2024.050332
Received 03 February 2024; Accepted 25 March 2024; Issue published 20 May 2024
Abstract
Seawater desalination stands as an increasingly indispensable solution to address global water scarcity issues. This study conducts a thorough exergoenvironmental analysis of a multi-effect distillation with thermal vapor compression (MED-TVC) system, a highly promising desalination technology. The MED-TVC system presents an energy-efficient approach to desalination by harnessing waste heat sources and incorporating thermal vapor compression. The primary objective of this research is to assess the system’s thermodynamic efficiency and environmental impact, considering both energy and exergy aspects. The investigation delves into the intricacies of energy and exergy losses within the MED-TVC process, providing a holistic understanding of its performance. By scrutinizing the distribution and sources of exergy destruction, the study identifies specific areas for enhancement in the system’s design and operation, thereby elevating its overall sustainability. Moreover, the exergoenvironmental analysis quantifies the environmental impact, offering vital insights into the sustainability of seawater desalination technologies. The results underscore the significance of every component in the MED-TVC system for its exergoenvironmental performance. Notably, the thermal vapor compressor emerges as pivotal due to its direct impact on energy efficiency, exergy losses, and the environmental footprint of the process. Consequently, optimizing this particular component becomes imperative for achieving a more sustainable and efficient desalination system.Keywords
Nomenclature
Environmental impact rate (Pts/h) | |
B | Environmental impact per unit of exergy (Pts/GJ) |
Bm | Environmental impact associated with the production of material components (steel) exergy (Pts/kg) |
Exergy rate (kW) | |
F | Environmental factor (%) |
Mass flow rate (kg/s) | |
N | Annual operating hours (h) |
N | Lifetime (yr) |
T | Temperature (°C) |
Minimum separation work (kW) | |
Component-related environmental impact rate associated with LCA (Pts/h) | |
Y | Environmental impact of the component (Pts) |
Greek symbols | |
Efficiency | |
Density | |
Thickness | |
Subscripts | |
1,2,…..,i | System state points |
B | Brine |
con | Condenser |
D | Destruction |
ef | Effect |
elec | Electricity |
ex | Exergy |
F | Fuel |
in | Inlet |
j | jth stream |
k | kth component |
min | Minimum |
p | Pump |
out | Outlet |
Abbreviations | |
EIGW | Environmental impact of generated water |
GA | Genetic algorithm |
MEB | Multi effect boiling |
MED | Multi effect distillation |
MSF | Multi-stage flash |
MVC | Mechanical vapor compression |
RO | Reverse osmosis |
TVC | Thermal vapor compression |
Highlights
• Modeling and optimizing a multi-effect desalination system with thermal vapor compression are carried out.
• Exergoenvironmental analysis and multi-objective optimization are performed.
• Sensitivity analysis of effective parameters on the system performance is investigated.
• Significant improvements in both exergy efficiency and environmental impact are obtained.
Desalination technologies have emerged as a vital solution to address the ever-growing global demand for freshwater. They play a central role in satisfying the water needs of both residents and industries, providing essential potable water and strengthening economic activities. As the adoption of desalination systems continues increasing worldwide, it has become imperative to assess their environmental impact as well as their energy and economic performance. In this context, different desalination technologies, including membrane and thermal techniques, are widely used [1,2].
Desalination systems are typically designed and optimized with a primary focus on energy and economic aspects, with exergy-based methods effectively facilitating the evaluation and enhancement of their performance. Among these technologies, Multi-Effect Desalination with Thermal Vapor Compression (MED-TVC) has garnered considerable attention due to its remarkable energy efficiency, simplicity, utilization of low-level thermal resources, and economic durability [3,4].
In comparing the MED-TVC system to the traditional seawater desalination methods in terms of energy efficiency, several factors come into play. MED-TVC systems typically exhibit higher thermal efficiency compared to traditional methods like Multi-Stage Flash (MSF) distillation or Reverse Osmosis (RO). This is due to their effective utilization of latent heat of vaporization by recycling it within the system, resulting in reduced energy consumption per unit of water produced. Furthermore, the integration of mechanical vapor compression in MED-TVC systems enhances the distillation process efficiency by compressing the vapor to higher temperature and pressure, thereby reducing the energy required for subsequent heating stages [5].
Additionally, MED-TVC systems can often maintain high efficiency across a wide range of capacities, making them suitable for decentralized or smaller-scale desalination applications where traditional methods may be less viable. Moreover, the integration of heat recovery mechanisms in MED-TVC systems allows for the capture and reuse of waste heat generated during the desalination process, further improving energy efficiency and reducing overall operating costs. Finally, while energy efficiency is crucial, it is also essential to consider the environmental impact. MED-TVC systems typically have lower carbon footprints compared to traditional methods, particularly if renewable energy sources are utilized for heat generation [6].
Numerous studies have investigated the MED-TVC performances through exergy analysis, which exposed critical information about the sources of exergy destruction within these systems. Rostami et al. [7] have explored the utilization of waste heat from wind turbines to drive a Multi-Effect Distillation Mechanical Vapor Compression (MED-MVC) desalination unit. This innovative approach aims to overcome challenges MED-TVC systems typically have lower carbon footprints compared to traditional methods, particularly if renewable energy sources are utilized for heat generation.
Related to high specific work consumption (SWC) when desalinating seawater from the waste heat of wind turbine generators. The MED-MVC unit, in this case, efficiently recovers brine rejected from a humidification-dehumidification (HDH) desalination unit, demonstrating promising results such as a significant decrease in SWC and an 18% increase in freshwater production at all wind speeds.
Hamed et al. [8] conducted an exergy analysis comparing a TVC system with conventional Multi-Effect Boiling (MEB) and mechanical vapor compression (MVC) systems. Among the three systems, the lowest exergy destruction was from the TVC unit. However, significant exergy destruction occurred within both the first effect and the thermo compressor integrated into the TVC system. Alasfour et al. [9] performed a parametric study on MED-TVC desalination systems. Their analysis showed that steam ejectors and evaporators were the primary sources of exergy destruction across three configurations. Lowering the top brine temperature led to a reduction in both exergy destruction and thermal energy consumption.
Energy and exergy analyses were conducted on a MED-TVC system, as performed by Choi et al. [10]. They found that the TVC and effects had the highest exergy losses. Binamer [11] conducted a comprehensive analysis of a MED-TVC system. They found that the first effect contributed significantly to exergy destruction. Expanding the number of effects and operating at lower brine temperatures notably reduced specific exergy destruction.
A mathematical model for predicting and optimizing the exergy efficiency of a multi-effect desalination system with thermal vapor compression was developed by Sadri et al. [12]. Their findings emphasized the importance of evaporators, identifying them as the main sources of exergy destruction. Menasri et al. [13] introduced a MED-TVC system model, employing comprehensive energy and exergy analyses. Their results highlighted that exergy destruction primarily occurred in the ejector and evaporators. They recommended operating at lower brine temperatures and reduced motive steam flow rates for improved overall efficiency. Abusorrah et al. [14] conducted energetic and exergetic analyses of multi-effect desalination using thermal vapor compression, highlighting the thermo-compressor’s high exergy destruction. Increasing effects and motive steam pressure improved some performance parameters but heightened irreversibility.
Almutairi et al. [15] developed a cogeneration plant model integrating a combined cycle power plant and a MED-TVC desalination system. Gas turbine engines and desalination unit effects were identified as the major sources of irreversibility. Al-Najem et al. [16] detailed advancements in thermal vapor compression (TVC) systems by Sidem (France), increasing interest in smaller capacity desalination plants due to reduced energy consumption, simpler water treatment, and cost-effectiveness. A parametric analysis using thermodynamics identified system deficiencies and potential improvement methods. Recent research has focused on assessing the economic efficiency of MED-TVC desalination systems through the application of the exergoeconomic approach.
Samaké et al. [17] performed an exergoeconomic evaluation on a multi-effect desalination system that employed ejector vapor compression. The study consistently demonstrated that increasing the number of effects in both forward and parallel/cross configurations resulted in higher total required investment and elevated unit costs for producing fresh water. Elsayed et al. [18] investigated the exergoeconomic performances of various feed configurations within a MED-TVC desalination system, focusing on economic factors such as cost index, interest rate, and electricity cost. Their findings highlighted the parallel cross feed (PCF) configuration as the most cost-efficient with a production cost of 2.09 USD/m3.
Additionally, Elsayed et al. [19] developed an exergoeconomic model for a MED-MVC integrated multi-effect desalination plant, revealing a total water price of 1.63 USD/m3. Fergani et al. [20] conducted an exergoeconomic assessment of an MED-TVC desalination system, focusing on the economic effectiveness of the parallel/cross feed configuration. Their approach involved utilizing a numerical model to evaluate how operational parameters influenced the total water price. Their findings indicated a cost of 1.73 USD/m3 for producing distilled water, based on a production rate of 55.20 kg/s. The MED effects were identified as the most crucial component from an exergoeconomic standpoint. These findings highlighted the imperative need to optimize these components for achieving cost-effective desalination.
In contrast to the numerous studies exploring the performance of MED-TVC systems through exergy analysis, Vojdani et al. [21] proposed an innovative integrated energy system integrating a multi-effect desalination unit (MED) with a solid oxide fuel cell (SOFC) and a gas turbine (GT). The study investigated the system’s performance from energy, exergy, economic, and environmental perspectives. The proposed system demonstrates a remarkable 6.5% enhancement in system power generation, 8.42% improvement in exergy efficiency, and a 5.8% reduction in normalized emission compared to the standalone SOFC-GT system.
Additionally, the study conducted by Modabber et al. [22] on a trigeneration system in Qeshm Island serves as a practical case, evaluating the potentials and proposing diverse scenarios to enhance the performance of a system comprising gas turbine units, heat recovery steam generators, and MED-TVC desalination units. The work explores innovative schemes, such as integrating inlet air cooling systems and coupling existing MED desalination units with reverse osmosis (RO) systems, leveraging solar thermal collector fields for improved system efficiency using advanced exergy, exergoeconomic, and exergoenvironmental analyses.
It is essential to emphasize that MED-TVC has not been studied in detail in previous works, because it was considered as part of a larger systems rather than being the focal point of investigation. This research addresses this gap by providing an in-depth analysis specifically dedicated to the MED-TVC desalination system, contributing valuable insights to the field. From the concise overview, it is evident that exergy-based analyses offer promising avenues for evaluation. However, there have been few publications exploring the application of exergoeconomic analysis specifically within MED-TVC systems. Numerous studies have investigated the MED-TVC systems’ performance employing the exergy analysis methodology, which has pinpointed components such as the thermo-compressor and evaporators as primary contributors to exergy destruction. However, a comprehensive analysis of the environmental implications of these systems, through an exergoenvironmental perspective, remains relatively underexplored. Furthermore, to the best of the authors’ knowledge, there are no published works conducting exergoenvironmental analysis for MED-TVC systems.
The aim of the present work is to perform an exergoenvironmental analysis for a MED-TVC desalination system to estimate the environmental impact of the system’s operation and identify the components that contribute the most to its environmental footprint. Analyzing the system’s environmental performance offers decision-makers valuable insights to optimize efficiency and concurrently minimize the environmental impact. Additionally, a parametric study will be performed to evaluate the influence of key process parameters, such as top brine temperature, seawater temperature, motive steam flow rate, and the number of effects, on the environmental impact of the fresh water produced. A multi-objective optimization will then be carried out to define the optimum design conditions of the MED-TVC system.
This study introduces innovative advancements in seawater desalination through the exploration of a MED-TVC system. The research uniquely combines an energy-efficient approach with a comprehensive exergoenvironmental analysis, evaluating both energy and exergy aspects. Notably, the study identifies specific areas for improvement in the MED-TVC system’s design and operation by scrutinizing the distribution and sources of exergy destruction. The quantification of environmental impact provides crucial insights for assessing the sustainability of seawater desalination technologies. Significantly, the research highlights the pivotal role of the thermal vapor compressor in influencing energy efficiency, exergy losses, and environmental impact within the MED-TVC system, underscoring the need for targeted optimization to enhance overall sustainability and efficiency. In summary, this study contributes significantly to advancing our understanding and optimization of seawater desalination processes.
2 System Description and Modeling
Fig. 1 illustrates the schematic of the MED system integrated with the TVC unit, comprising four effects (evaporators), a steam ejector (thermos-compressor), a condenser, and pumps designated for managing seawater, brine, and distillate. In our study, we have opted for the PCF feed configuration in the MED-TVC desalination system. Seawater cools distillate in the condenser which increases the seawater feed temperature. The seawater leaving the condenser splits into two streams: one returns to the tank, the other is divided into four parts for the effects. Motive steam mixes with entrained steam in the ejector, compressing the combined vapor to fulfill the first thermal requirement. A portion of this vapor heats seawater in the condenser, producing distillate.
Figure 1: Schematic diagram of the MED-TVC desalination system [20]
Vapor from each effect moves to the next sequentially, while brine from each effect is directed to the subsequent ones until discharge as brine from the last effect. Condensate steam is split into two parts with one part feeding the steam cycle of a steam power plant, while the other joining the collected streams in the fresh water line. The system was analyzed through thermodynamic modeling in MATLAB software, employing mass, energy, and exergy equations and considering certain assumptions including: the system functions under steady-state conditions; the effects maintain a uniform temperature difference and a consistent feed-flow rate; both the distillate and vapor are devoid of salt, and an average pump efficiency of 75% was assumed. It should be noted that the authors have previously provided an extensive explanation about the calculation of seawater properties such as enthalpy, entropy, chemical potential, and density. We also presented and validated the developed thermodynamic model of the combined cycle in the MED-TVC desalination system in our prior work [13,20], therefore, this part of research is not reported here.
3 Exergoenvironmental Analysis and Methodology
The exergoenvironmental analysis involves assessing the environmental impacts of different components within a specific system, along with identifying the sources of these impacts. This approach integrates exergy analysis with a comprehensive environmental impact evaluation technique. To evaluate the environmental impacts of system components and their associated inputs, a thorough life cycle assessment (LCA) is conducted. In this study, the Eco-indicator 99 [23] method is chosen due to its extensive use.
The exergoenvironmental evaluation follows a similar allocation approach to the exergoeconomic evaluation, where environmental impact results are allocated to the exergy streams of the system. This allocation process parallels the one used in exergoeconomic evaluations for assigning costs to exergy streams [24]. Detailed in Table 1 are the balance equations for environmental impact rates formulated for operational MED-TVC unit. These equations are very similar to the exergetic cost rate equations presented in [20] for the same MED-TVC system, with the substitution of cost-related terms with terms related to environmental impact.
The environmental impact of each system component can be expressed as a balance:
where
where
The equations of environmental impact (
Exergoenvironmental analysis provides several key factors that play a significant role in developing strategies to enhance system environmental performance, such as:
• Environmental impact of exergy destruction [24]:
• Exergoenvironmental factor:
4 Multi-Objective Optimization
The MED-TVC presents a complex scenario with multiple conflicting objectives. Maximizing exergy efficiency and minimizing the environmental impact of produced water are paramount objectives. These objectives are addressed through a multi-objective optimization framework aimed at determining optimal design parameters and operational conditions.
In this study, a genetic algorithm (GA) implemented in MATLAB 2018b, is employed. This GA leverages selection, crossover, and mutation rules to iteratively evolve the population of solutions [26]. The optimization approach relies on the principles of Pareto optimality and dominance. By considering the trade-offs between exergy efficiency and environmental impact, the GA aims to generate solutions that form the Pareto front, showcasing the optimal compromises between the conflicting objectives.
The choice of objective functions and decision variables significantly influences the optimal conditions within the MED-TVC system. Exergy efficiency and the environmental impact of generated water are selected as objective functions. These objective functions can be expressed as:
• Exergy efficiency: the ratio between the minimum separation work (
• Environmental impact of generated water: the ratio between the sum of the environmental impact of outgoing water streams from the desalination unit and the quantity of desalinated water.
Additionally, specific decision variables are chosen for optimizing the MED-TVC system and they are top brine temperature, seawater temperature, and motive steam flow.
5.1 Exergoenvironmental Analysis
This section presents the results obtained from evaluating the environmental impacts of the MED-TVC unit. This evaluation is based on the comprehensive exergy analysis documented in [20]. The principal input parameters and their values used for modeling the MED-TVC system are detailed in Table 2. The integrity of the MED-TVC system’s thermodynamic model was confirmed through validation in our previous paper [20] using the data reported by Sadri et al. [12].
The exergoenvironmental characteristics with the exergy values at each state point of the MED-TVC unit are presented in Table 3 based on the formulations provided for environmental impact rates in Table 2, indicating a value of 14.13 Pts/h for the environmental impact of the produced fresh water.
Fig. 2 illustrates the environmental impact rates associated with the exergy destruction rates and their proportional contributions for each component of the MED-TVC system. It can be seen that the significant environmental impacts associated with exergy destruction are notably prominent within the condenser (22%) followed by the individual effects, namely effect 1 through effect 4, that exhibit distinct environmental impact, with effect 1 and effect 4 representing 20% and 18%, respectively, followed by effect 2 and effect 3 both at 14%, due to their significant exergy destruction. The thermo-compressor accounts for 8% of this impact, while the pumps show notably lower contribution in comparison.
Figure 2: (a) Environmental impact rates associated with the exergy destruction and (b) Proportional contribution of each component in percentages
In Fig. 3, the component-related environmental impact rates and their percentage-wise contribution for each component of the MED-TVC system are illustrated. According to the obtained results, the thermos-compressor demonstrates the highest component-associated environmental impact rate of 24%, followed by the condenser registering environmental impact rate of 18%, while the seawater pump accounts for 14% of the environmental impact. Moreover, effect 1 to effect 4 contribute significantly at 8%, 9%, 9%, and 9%, respectively, reflecting their collective impact on the system’s environmental impact. Additionally, considering the operational components, the product pump accounts for 3%, while other pumps collectively contribute 1% to the system’s environmental impact.
Figure 3: Component-related environmental impact rates (a) and their percentage (b)
Fig. 4 presents the exergoenvironmental factor (f) of the components. It can be seen that for all components, the value of f is lower than 30% which means that the environmental impact resulting from exergy destruction is the dominant source for environmental impact [20], so enhancing environmental performance should focus on minimizing thermodynamic inefficiencies.
Figure 4: Exergoenvironmental factor of system components
Parametric study is carried out to explore the effects of different variables on the environmental impact of generated water (EIGW) in the MED-TVC system. The principal parameters examined include top brine temperature, seawater temperature, motive steam flow, and effect number. It is worth noting that the effect of these parameters on exergy efficiency has been previously studied in detail, as documented in article [13].
Fig. 5 illustrates the variation of the environmental effect of generated fresh water with the top brine temperature. As shown in this figure, the environmental impact monotonically rises with the increase of the top brine temperature, the progression from 55°C to 65°C demonstrates a proportional increase in the environmental impact, as reflected in the values transitioning from 382 to 557.14 mPts/m3.
Figure 5: Effect of top brine temperature on the environmental impact of distilled water
Fig. 6 presents the environmental impact of generated fresh water vs. the seawater temperature. It shows that the environmental impact decreases with increasing the seawater temperature. The effect of the motive steam flowrate on the values of the environmental impact of generated fresh water is indicated in Fig. 7. The figure shows that as the motive steam flowrate increases, the environmental impact decreases. In Fig. 8, the variation of the environmental impact of generated fresh water with the number of effects is illustrated. As the number of effects increases the environmental impact decreases.
Figure 6: Effect of cooling seawater temperature on the environmental impact of distilled water
Figure 7: Effect of motive steam flow rate on the environmental impact of distilled water
Figure 8: Influence of the number of effects on the environmental impact of distilled water
Fig. 9 illustrates the Pareto front solution delineating the relationship between objective functions in the multi-objective optimization of the MED-TVC plant. The graphical representation vividly portrays the trade-off between the two objectives, resulting in the distinct Pareto front curve. As it can be observed in Fig. 9, the Pareto front curve represents three key optimal points that are selected based on different preference criteria: points A and B illustrate scenarios similar to single-objective function optimization, whereas point C represents the best compromise solution determined through fuzzy-based mechanism [27].
Figure 9: Pareto optimal front from multi-objective optimization of MED-TVC plant
Table 4 displays the objective function values and decision variable values corresponding to Pareto points A, B, and C, as well as the base case values. Point A exhibits the highest exergetic efficiency at 6.92% with an environmental impact of 560.6 mPts/m3. Point B, on the other hand, displays the best environmental impact at 376.1 mPts/m3 but with a slightly lower exergetic efficiency of 5.08%. Point C seems to strike a balance between the two objectives, showing an exergetic efficiency of 6.6% and an impact of 406.3 mPts/m3.
Comparing these optimized points to the base case, where the exergetic efficiency was 3.46% and the environmental impact at 475.9 mPts/m3, it is evident that the optimization process has made significant improvements. Point A more than doubles the base case exergy efficiency while marginally increases the environmental impact. On the other hand, point B shows a remarkable reduction in environmental impact (26.5%) at the cost of a moderate decrease in exergy efficiency compared to the base case. Point C appears to be a promising compromise, providing a substantial increase in exergy efficiency (47.5%) compared to the base case while maintaining a reasonable reduction in environmental impact (17.1%).
In this study, for the first time, exergoenvironmental analysis is applied to a parallel/cross MED-TVC desalination system. Subsequently, a comprehensive parametric study is conducted to investigate the impacts of various parameters on the system’s performance. Moreover, a multi-objective optimization strategy is implemented to enhance the system’s efficiency while considering environmental impact. According to the analysis, the following conclusions can be drawn:
• The TVC unit, effects, and condenser are identified as having the highest environmental impact among all system components. This underscores the importance of targeted optimization in these areas for overall sustainability improvement.
• Notably, our findings reveal that the environmental impact resulting from exergy destruction within all components of the MED-TVC plant is significantly greater than the component-related environmental impact. This insight emphasizes the potential for lowering the overall environmental impact by reducing exergy destruction within these components.
• The top brine temperature, seawater temperature, motive steam flow rate, and the number of effects, are identified as influential factors shaping the exergoenvironmental performance of the MED-TVC system. Understanding the impact of these parameters is critical for informed decision-making in system design and operation.
• Through the multi-objective optimization approach, our study achieves significant improvements in both exergy efficiency and environmental performance when compared with the base case of the MED-TVC system; with Pareto points A, B, and C in Fig. 9 exhibiting, respectively, exergetic efficiencies of 6.92%, 5.08%, and 6.6%, as well as environmental impacts of 560.6, 376.1, and 406.3 mPts/m3, representing significant advancements compared to the base case values of 3.46% exergetic efficiency and 475.9 mPts/m3 environmental impact. This signifies the practical benefits of considering environmental impact alongside efficiency in the optimization process.
Finally, the integration of exergoenvironmental analysis and the application of a multi-objective optimization framework constitute innovative contributions to the study of MED-TVC desalination systems. It is recommended that future works should not only continue to explore these novel methodologies but also incorporate dynamic studies. This dynamic perspective is crucial for adapting desalination systems to diverse operating conditions, ensuring an efficient response in real-world scenarios. This comprehensive approach will guide advancements in sustainable desalination technologies, fostering a more adaptable and environmentally conscious future for water resource management.
Acknowledgement: This work was supported in entire part by the Biomaterials and Transport Phenomena Laboratory Agreement No. 303 03-12-2003, at the University of Medea. The authors acknowledge and gratefully thank the financial support provided by DG-RSDT of Algeria.
Funding Statement: The authors received no specific funding for this study.
Author Contributions: Conceptualization, Zineb Fergani, Zakaria Triki, Rabah Menasri, Hichem Tahraoui, Abdeltif Amrane, Meriem Zamouche, Mohammed Kebir and Jie Zhang; Methodology, Zineb Fergani, Zakaria Triki, Rabah Menasri, Hichem Tahraoui, Abdeltif Amrane, Meriem Zamouche, Mohammed Kebir and Jie Zhang; Software, Zineb Fergani, Zakaria Triki, Rabah Menasri, Hichem Tahraoui, Abdeltif Amrane and Jie Zhang; Validation, Zineb Fergani, Zakaria Triki, Rabah Menasri, Hichem Tahraoui, Abdeltif Amrane, Meriem Zamouche, Mohammed Kebir and Jie Zhang; Formal analysis, Zineb Fergani, Zakaria Triki, Rabah Menasri, Hichem Tahraoui, Abdeltif Amrane, Meriem Zamouche, Mohammed Kebir and Jie Zhang; Investigation, Zineb Fergani, Zakaria Triki, Rabah Menasri, Hichem Tahraoui, Abdeltif Amrane, Meriem Zamouche, Mohammed Kebir and Jie Zhang; Resources, Zakaria Triki, Rabah Menasri, Hichem Tahraoui, Abdeltif Amrane and Jie Zhang; Data curation, Zineb Fergani, Zakaria Triki, Rabah Menasri, Abdeltif Amrane and Jie Zhang; Writing–original draft, Zineb Fergani, Zakaria Triki and Rabah Menasri; Writing–review & editing, Hichem Tahraoui, Abdeltif Amrane, Meriem Zamouche, Mohammed Kebir and Jie Zhang; Visualization, Zineb Fergani, Zakaria Triki, Rabah Menasri, Hichem Tahraoui, Abdeltif Amrane, Meriem Zamouche, Mohammed Kebir and Jie Zhang; Supervision, Zakaria Triki, Abdeltif Amrane and Jie Zhang; Project administration, Zakaria Triki, Hichem Tahraoui, Abdeltif Amrane and Jie Zhang.
Availability of Data and Materials: Not applicable.
Conflicts of Interest: The authors declare that they have no conflicts of interest to report regarding the present study.
References
1. Curto, D., Franzitta, V., Guercio, A. (2021). A review of the water desalination technologies. Applied Sciences, 11(2), 670. https://doi.org/10.3390/app11020670 [Google Scholar] [CrossRef]
2. Ahmed, F. E., Khalil, A., Hilal, N. (2021). Emerging desalination technologies: Current status, challenges and future trends. Desalination, 517, 115183. https://doi.org/10.1016/j.desal.2021.115183 [Google Scholar] [CrossRef]
3. Mistry, K. H., Antar, M. A., Lienhard, V. J. H. (2013). An improved model for multiple effect distillation. Deasalin Water Treatment, 51, 807–821. https://doi.org/10.1080/19443994.2012.703383 [Google Scholar] [CrossRef]
4. Ophir, A., Lokiec, F. (2005). Advanced MED process for most economical sea water desalination. Desalination, 182, 187–198. https://doi.org/10.1016/j.desal.2005.02.026 [Google Scholar] [CrossRef]
5. Wazeer, I., Al-Mutaz, I. (2014). Current status and future directions of MED-TVC desalination technology. Desalina Water Treatment, 55, 1–9. [Google Scholar]
6. Li, Y., Chen, X., Xu, Y., Zhuo, Y., Lu, G. (2021). Sustainable thermal-based desalination with low-cost energy resources and low-carbon footprints. Desalination, 520, 115371. https://doi.org/10.1016/j.desal.2021.115371 [Google Scholar] [CrossRef]
7. Rostami, S., Ghiasirad, H., Rostamzadeh, H., Kalan, A. S., Maleki, A. (2023). A wind turbine driven hybrid HDH-MEDMVC desalination system towards minimal liquid discharge. South African Journal of Chemical Engineering, 44, 356–369. https://doi.org/10.1016/j.sajce.2023.03.007 [Google Scholar] [CrossRef]
8. Hamed, O. A., Zamamiri, A. M., Aly, S., Lior, N. (1996). Thermal performance and exergy analysis of a thermal vapour compression desalination system. Energy Conversion and Management, 37, 379–387. https://doi.org/10.1016/0196-8904(95)00194-8 [Google Scholar] [CrossRef]
9. Alasfour, F. N., Darwish, M. A., Amer, A. B. (2005). Thermal analysis of ME—TVC+MEE desalination systems. Desalination, 174, 39–61. https://doi.org/10.1016/j.desal.2004.08.039 [Google Scholar] [CrossRef]
10. Choi, H. S., Lee, T. J., Kim, Y. G., Song, S. L. (2005). Performance improvement of multiple-effect distiller with thermal vapour compression system by exergy analysis. Desalination, 182, 239–249. https://doi.org/10.1016/j.desal.2005.03.018 [Google Scholar] [CrossRef]
11. Binamer, A. (2015). Second law and sensitivity analysis of large ME-TVC desalination units. Desalin Water Treatment, 53, 1234–1245. [Google Scholar]
12. Sadri, S., HaghighiKhoshkhoo, R., Ameri, M. (2017). Multi objective optimization of the MED-TVC system with exergetic and heat transfer analysis. Energy Equipment System, 5, 419–430. [Google Scholar]
13. Menasri, R., Triki, Z., Bouaziz, M. N., Hamrouni, B. (2022). Energy and exergy analyses of a novel multi-effect distillation system with thermal vapour compression for seawater desalination. Desalin Water Treatment, 246, 54–67. https://doi.org/10.5004/dwt. [Google Scholar] [CrossRef]
14. Abusorrah, A. M., Mebarek-Oudina, F., Ahmadian, A., Baleanu, D. (2021). Modeling of a MED-TVC desalination system by considering the effects of nanoparticles: Energetic and exergetic analysis. Journal of Thermal Analysis and Calorimetry, 144, 2675–2687. https://doi.org/10.1007/s10973-020-10524-1 [Google Scholar] [CrossRef]
15. Almutairi, A., Pilidis, P., Al-Mutawa, N., Al-Weshahi, M. (2016). Energetic and exergetic analysis of cogeneration power combined cycle and ME-TVC-MED water desalination plant: Part-1 operation and performance. Applied Thermal Engineering, 103, 77–91. https://doi.org/10.1016/j.applthermaleng.2016.02.121 [Google Scholar] [CrossRef]
16. Al-Najem, N. M., Darwish, M. A., Youssef, F. A. (1997). Thermovapor compression desalters: Energy and availability— Analysis of single-and multi-effect systems. Desalination, 110, 223–238. https://doi.org/10.1016/S0011-9164(97)00101-X [Google Scholar] [CrossRef]
17. Samaké, O., Galanis, N., Sorin, M. (2018). Thermo-economic analysis of a multiple-effect desalination system with ejector vapour compression. Energy, 144, 1037–1051. https://doi.org/10.1016/j.energy.2017.12.112 [Google Scholar] [CrossRef]
18. Elsayed, M. L., Mesalhy, O., Mohammed, R. H., Chow, L. C. (2019). Performance modeling of MED-MVC systems: Exergy-economic analysis. Energy, 166, 552–568. https://doi.org/10.1016/j.energy.2018.10.080 [Google Scholar] [CrossRef]
19. Elsayed, M. L., Mesalhy, O., Mohammed, R. H., Chow, L. C. (2019). Transient and thermo-economic analysis of MEDMVC desalination system. Energy, 16, 283–296. [Google Scholar]
20. Fergani, Z., Triki, Z., Menasri, R., Tahraoui, H., Kebir, M. et al. (2023). Analysis of desalination performance with a thermal vapor compression system. Water, 15(6), 1225. https://doi.org/10.3390/w15061225 [Google Scholar] [CrossRef]
21. Vojdani, M., Fakhari, I., Ahmadi, P. (2021). A novel triple pressure HRSG integrated with MED/SOFC/GT for cogeneration of electricity and freshwater: Techno-economic-environmental assessment, and multi-objective optimization. Energy Conversion and Management, 233, 113876. https://doi.org/10.1016/j.enconman.2021.113876 [Google Scholar] [CrossRef]
22. Modabber, H. V., Manesh, M. H. K. (2021). Optimal exergetic, exergoeconomic and exergoenvironmental design of polygeneration system based on gas turbine-absorption chiller-solar parabolic trough collector units integrated with multi-effect desalination-thermal vapor compressor-reverse osmosis desalination systems. Renew Energy, 165, 533–552. [Google Scholar]
23. Goedkoop, M., Spriensma, R. (2000). The eco-indicator 99, a damage-oriented method for life cycle impact assessment. Amersfoort, The Netherlands: Methodology Report, PRé Consultants. [Google Scholar]
24. Meyer, L., Tsatsaronis, G., Buchgeister, J., Schebek, L. (2009). Exergoenvironmental analysis for evaluation of the environmental impact of energy conversion systems. Energy, 34, 75–89. https://doi.org/10.1016/j.energy.2008.07.018 [Google Scholar] [CrossRef]
25. Antipova, E., Boer, D., Cabeza, L. F., Guillén-Gosálbez, G., Jiménez, L. (2013). Multi-objective design of reverse osmosis plants integrated with solar Rankine cycles and thermal energy storage. Appl Energy, 102, 1137–1147. https://doi.org/10.1016/j.apenergy.2012.06.038 [Google Scholar] [CrossRef]
26. Holland, J. H. (1992). Adaptation in nature and artificial systems: an introductory analysis with applications to biology, control and artificial intelligence. Massachusetts: MIT Press. [Google Scholar]
27. Abido, M. A. (2003). Environmental/economic power dispatch using multiobjective evolutionary algorithms. IEEE Transactions on Power Systems, 18, 1529–1537. https://doi.org/10.1109/TPWRS.2003.818693 [Google Scholar] [CrossRef]
Cite This Article
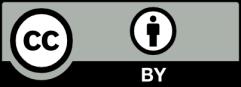
This work is licensed under a Creative Commons Attribution 4.0 International License , which permits unrestricted use, distribution, and reproduction in any medium, provided the original work is properly cited.