Open Access
ARTICLE
The Paraffin Crystallization in Emulsified Waxy Crude Oil by Dissipative Particle Dynamics
1 Power China Chongqing Engineering Co., Ltd., Chongqing, 400060, China
2 College of Electrical Engineering, Xinjiang University, Urumqi, 830017, China
3 Key Laboratory of Low-Grade Energy Utilization Technologies and Systems, Ministry of Education, School of Energy and Power Engineering, Chongqing University, Chongqing, 400030, China
* Corresponding Author: Jinchen Cao. Email:
(This article belongs to the Special Issue: Two-phase flow heat and mass transfer in advanced energy systems)
Frontiers in Heat and Mass Transfer 2024, 22(1), 129-139. https://doi.org/10.32604/fhmt.2024.047825
Received 19 November 2023; Accepted 14 December 2023; Issue published 21 March 2024
Abstract
With the advancement of oilfield extraction technology, since oil-water emulsions in waxy crude oil are prone to be deposited on the pipe wall, increasing the difficulty of crude oil extraction. In this paper, the mesoscopic dissipative particle dynamics method is used to study the mechanism of the crystallization and deposition adsorbed on the wall. The results show that in the absence of water molecules, the paraffin molecules near the substrate are deposited on the metallic surface with a horizontal morphology, while the paraffin molecules close to the fluid side are arranged in a vertical column morphology. In the emulsified system, more water molecules will be absorbed on the metallic substrate than paraffin molecules, which obstructed the direct interaction between paraffin molecules and solid surface. Therefore, the addition of water molecules hinders the crystallization of wax near the substrate. Perversely, on the fluid side, water molecules promote the formation of paraffin crystallization. The research in this paper reveals the crystallization mechanism of paraffin wax in oil-water emulsions in the pipeline from the microscopic scale, which provides theoretical support for improving the recovery of wax-containing crude oil and enhancing the transport efficiency.Keywords
Supplementary Material
Supplementary Material FileFuel fossils is the predominant energy source right now. With the continuous development of oil and gas resources, the crude oil produced in China has the characteristics of a high freezing point, high wax content, and high viscosity. Under the traditional temperature conditions of mining and transportation, the paraffin component in crude oil easily reaches its freezing point [1], which leads to the gradual precipitation of paraffin molecules from the crude oil component, and the mutual eutectic forms a large network structure attached and deposited on the inner wall of the pipeline [2]. This phenomenon will cause problems such as pipeline blockage and corrosion of pipeline equipment, which becomes one of the key issues to be solved in the crude oil production process.
Crude oil is composed of saturated alkanes, asphaltenes, paraffins, asphalt colloids, and other macromolecular organic compounds [3,4]. This complex structure brings severe challenges to the study of the precipitation process of paraffin crystals from crude oil. In addition, with the in-depth development of oilfields, the water content of the produced crude oil has increased year by year, and the multiphase mixed transportation technology of emulsified water-containing waxy crude oil has gradually become the main way onshore and offshore [5,6]. Most of the current research uses the classical single-phase crude oil wax molecular diffusion theory to explain the precipitation, crystallization and deposition process of paraffin crystals [7,8]. However, there are few studies on the mechanism of the existence of multiphase dispersed water droplets on the wax crystallization and deposition process, of which most reports on this problem mainly focus on experimental and theoretical models.
Haj-Shafiei et al. [9] explored the structure and growth morphology of paraffin crystals under different cooling rates by laser scanning confocal microscopy. Zheng et al. [10] investigated the effect of water droplets on the diffusion of wax molecules in water-in-oil dispersions by means of nuclear magnetic resonance. Paso et al. [11] observed the crystallization process of paraffin crystals and divided it into nucleation lag stage, supersaturated growth stage and equilibrium growth stage. Besides, with the development of computer science and technology, molecular simulation (MS) methods were applied to study the phase transition, aggregation, morphological structure and crystal morphology of paraffin molecules in waxy crude oil [12,13].
In terms of micro- and nano-scale molecular simulations, many scholars have established simplified molecular dynamics models for paraffin system [14–16]. San-Miguel et al. [17] used the molecular dynamics (MD) method to study the deposition process of paraffin molecules (C28) on the surface of Fe2O3, and found that paraffin molecules on the (0001) crystal plane are easier to be deposited. Similarly in general, the method of microscopic simulation has the disadvantages of relatively small research system and short simulation time, and cannot establish a direct link between macroscopic experiments and simulation in terms of micro scale. However, the mesoscopic scale simulation can solve the above problems to a certain extent, making the waxy crude oil system closer to the macroscopic system in space and time, and then establishing the connection between the microscopic simulation and the macroscopic experiment. In recent years, the dissipative particle dynamics (DPD) method at the mesoscopic scale has been gradually developed and applied to the fields such as petroleum systems [18,19]. Bustamante-Rendón et al. [20] employed DPD to study the effects of cationic and anionic surfactants on oil recovery, and found that surfactants can effectively reduce the surface tension of the oil-water interface. Wang et al. [21] studied the effect of Janus nanoparticles on the oil-water interfacial tension. Moncayo-Riascos et al. [22] combined MD and DPD method to reveal the interaction of fluids and fluid/solid surfaces, and simulated the deposition of asphaltenes on hematite (Fe2O3) surfaces. McDonagh et al. [23] investigated the crystallization process of single-component and mixed-component paraffin linear alkanes and branched alkanes at 298 K. In general, the previous DPD reports mainly focus on oil-water interfacial tension and modification, polymer surfactants and aggregation of asphaltenes [24]. However, the phenomenon of paraffin crystallization in emulsified waxy crude oil and the action mechanism of dispersed water droplets on paraffin crystals in emulsified crude oil still need to be solved.
To this end, the DPD method is employed in this paper to simulate the crystallization process and morphology of single-component paraffin crystals in the emulsified waxy crude oil on the tube surface from the mesoscopic scale, by using open source software LAMMPS [25]. The mechanisms of water molecules on the crystallization, migration, growth and deposition of paraffin crystals is analyzed.
DPD was first proposed by Hooger-brugge and Koelman et al. and improved by Groot et al. [26,27]. Its basic principle is to use bead to replace one or several groups of molecules, or to use a large number of beads to represent a kind of fluid. This reasonable simplification of the interactions between the beads enables the simulations to greatly increase the time and space scales [19]. The force field parameters selected in this paper refer to the DPD force field parameters of Bray et al. [28]. Meanwhile, the 12-6 Lennard-Jones (L-J) interaction potential function is selected to depict the interaction between the beads and the metallic surface, See Appendix for details of the force field parameters (Appendix-A1 Table 1 to Table 3).
As displayed in Fig. 1, two models, paraffin molecule (n-C35)/metallic substrate and paraffin molecule (n-C35)/water molecule/metal wall, were established, respectively. FCC is the face-centered lattice, and the lattice constant is set to 3.67. The size of the simulation box is 30 × 30 × 250 DPD units. The height of the metal wall is 15 DPD units, and the density of the system composed of water beads is ρ = 3 DPD units. The interaction of the system is described by the mixed interaction potential, in which the interaction between paraffin beads and beads is described by DPD potential function, and the potential function between paraffin and metal wall is described by 12-6 Lennard-Jones potential function. The time step of the simulation is 0.001 DPD units, and the model is fully relaxed with a relaxation time of 300 × 104 steps. The NVT ensemble is used for the fluid, the metal wall is fixed, the simulation time is 400 × 104 steps, and the simulation temperature is T = 1 DPD units (reference temperature is 298.15 K), more details see about DPD units in Attachment (Appendix-A1 Table 4).
Figure 1: Models of the two systems (a) paraffin molecule (n-C35)/metal wall; (b) paraffin molecule (n-C35)/water molecule/metal wall
By simulating two paraffinic alkanes with different chain lengths and comparing with the literature [28], the accuracy of the simulated force field was verified (More information in Appendix-A2).
3.1 Crystallization and Deposition of Single-Phase Emulsified Crude Oil Paraffin
For the model of the system with metal wall and paraffin, at different temperatures including 278, 288, 298 and 313 K, the crystallization and deposition of paraffin molecules on the metal wall were simulated. The snapshots of the simulation process under different temperature are captured as shown in Fig. 2.
Figure 2: Snapshots of the crystallization and deposition of paraffin molecules on the metal wall at different temperature
With the increase of temperature, the orderly arrangement of paraffin molecules decreases, indicating that with the increase of temperature, the structure is transformed that paraffin crystals gradually change from the ordered structure of the solid phase to the disordered lattice of the liquid phase. In addition, it can be found that in the area far from the wall surface, the overall structure of the paraffin crystal lattice is arranged vertically, while in the near wall surface, the paraffin molecules adsorbed on the metal wall surface are arranged horizontally, as shown in Fig. 3.
Figure 3: Arrangement and distribution of paraffin molecules on the metal wall
Next, the ordered parameters S is employed to analysis the simulation. In the simulation of the system with paraffin and metal wall, the Z direction of the box is much longer than the X and Y directions, so the geometric center of the fluid area above the wall is taken as the origin, and the molecules, located in the cube whose centroid coordinates is 30 × 30 × 90 DPD units are selected as the calculation object. The specific selection criteria are shown in Fig. 4a, and nematic parameter S are displayed in Fig. 4b.
Figure 4: Nematic parameters S under different temperature. (a) selection criteria, (b) nematic parameter
As presented in Fig. 4b, it can be seen that with the continuous increase of the temperature of the paraffin molecules, the orientation and nematic parameters S of the wax crystals gradually increase, and the wax crystals gradually change from the amorphous state to the crystalline state. With the further decrease of the temperature, the value of the nematic parameter S gradually tends to 1.0 and reaches stability. In addition, it can also be found that the changes of the nematic parameter S generally show the same change trends, which indicates the transition time from the amorphous state to the crystalline state is relatively short, and a stable crystal structure can be achieved in a quick period of time. Zhao et al. [29] called the abrupt point of the directional nematic parameter at each temperature as the nematic-isotropic transition temperature, which indicated that within this time range and at the same temperature, the transition time of paraffin crystals from non-ordered state to ordered state is short, and the nematic parameter S changes abruptly. It can be found that the lattice arrangement of paraffin crystals far from the wall also presents a nematic structure. Paraffin crystals and rigid nematic liquid crystals are similar in crystal structure, and the change trend of the nematic parameter S of the two is also consistent, indicating that paraffin crystals and rigid liquid crystal structures are similar in crystal structure.
3.2 Crystallization and Deposition of Multiphase Aqueous Emulsified Crude Paraffin
For further investigate the effect of water molecules on the crystallization and deposition of paraffin on the metal tube wall, a structural model was established as shown in Fig. 1b. When the temperature is 283 K, the simulation snapshots are presented in Fig. 5.
Figure 5: Snapshots of the system with paraffin molecule (n-C35)/water molecule/metal wall
It can be seen from Fig. 5a that when the temperature at 298 K, the arrangement of paraffin molecules has gradually tended to an ordered crystalline structure from Fig. 5b, in the part close to the metal wall, which adsorbs more water molecules than paraffin molecules.
The density distribution curves of paraffin molecules and water molecules along the Z-axis direction were calculated and shown in Fig. 6. It can be found that the distribution of water molecules is mostly on the near-wall side (Z > 15), while the distribution of paraffin molecules is relatively uniform. This reason for that is when the temperature under 298 K, the paraffin molecules have already crystallized with each other, while for the water molecules, they are still in a liquid state, and thus easier to migrate to the wall. The water molecules are more easily absorbed on the wall than paraffin molecules.
Figure 6: Density distribution of paraffin molecules and water molecules in the Z direction
It can be revealed from Fig. 7, near the wall, the kinetic energy of paraffin molecules increases firstly and then decreases along the Z direction. As the distance in the Z direction increases, the density distribution of paraffin molecules gradually presents a uniform state with some bubble-like distributions. Therefore, on the near-wall side, the paraffin molecules are mainly adsorbed on the water film or the metal wall, so the kinetic energy is relatively small. Since most of the water molecules are gathered in the area near the wall, and the kinetic energy of the water molecules is greater than that of the paraffin molecules, and the kinetic energy of the water molecules can be transferred to the paraffin molecules, and the speed of the paraffin molecules will increase near the wall. As the distribution number of water molecules gradually decreases along the Z direction, it is easier to form a stable crystalline structure between paraffin molecules, which leads to a gradual decrease in the kinetic energy of paraffin molecules. Additionally, the bubble-like velocity distribution on the side away from the wall is due to the increase of the kinetic energy of the local paraffin molecules caused by a small amount of water molecules.
Figure 7: Velocity distribution map of paraffin molecules along the Y and Z directions
In order to further investigate the effect of adding water molecules on the crystallization and deposition of paraffin on the metal wall, Fig. 8 shows the changes of the nematic parameter S of the system before and after adding water molecules.
Figure 8: Nematic parameters of paraffin molecules before and after the addition of water molecules
It can be found from Fig. 8 that for the two systems, at the temperature of 288 K. with the addition of water molecules, the nematic parameter S of paraffin crystals decreased significantly, and the fluctuation became severe.
The above results can indicate that water molecules inhibit the crystallization behavior of paraffin crystals to a certain extent, and hinder the formation of more ordered and stable crystal structures between paraffin molecules. This conclusion is in line with the results reported by Zheng et al. [30]. In addition, the reason for the fluctuation of the above curve can be explained by the velocity distribution in Fig. 7, that is, the water molecules transfer its kinetic energy to the paraffin molecules so that the paraffin molecules that should be crystallized at this temperature can generate a certain translation and rotation. Besides, since water molecules are adsorbed on the metal wall first, which causes paraffin molecules to be less likely to be deposited on the metal wall, the paraffin in the deposited layer is reduced. This conclusion is consistent with the previous experiments results, which is the wax content of the sedimentary layer decreases gradually with the increase of water content.
In this paper, the DPD method is used to analyze the crystallization process, structural characteristics and crystal morphology of single-component paraffin molecules in aqueous emulsified waxy crude oil. Then, the influence of water molecules on the crystallization and deposition of paraffin molecules on the metal wall was analyzed. The main conclusions are as follows:
(1) The morphology of single-component paraffin crystals deposited on the wall can be mainly divided into two types: on the near-wall side, paraffin crystals are distributed on the metal wall in a horizontal manner; On the fluid side (the side away from the wall surface), the distribution of paraffin crystals exhibits a nematic vertical distribution. Meanwhile, with the increase of temperature, the orderly degree of paraffin crystals decreased gradually.
(2) On the near-wall side, by comparing the changes of the nematic parameter of the system before and after the addition of water molecules, the results show that the addition of water molecules significantly reduces the crystallographic order of paraffin crystals. By studying the density distribution, it was found that the water molecules are more densely distributed on the near-wall side and adsorbed on the metal earlier than the paraffin molecules. This shows that in the paraffin deposition layer, on the one hand, a large amount of water is distributed near the metal wall surface, which weakens the interaction between paraffin molecules and the metal wall, and hinders the deposition of paraffin molecules to a certain extent. On the other hand, based on the velocity distribution, it is indicated that the near-wall water molecules exchange momentum with the paraffin molecules, which hinders the formation of a stable three-dimensional network crystal structure between the paraffin molecules.
(3) On the fluid side, the kinetic energy conversion between paraffin molecules and water molecules gradually weakens due to the gradual decrease in the content of water molecules, which explains the reason for the fluctuation of the system nematic parameters at this time. Meanwhile, the network crystal structure formed between the paraffin molecules surrounds the water molecules.
Acknowledgement: None.
Funding Statement: This work was sponsored by Natural Science Foundation of Xinjiang Uygur Autonomous Region, Grant No. 2023D01C197 and Performance Incentive Guidance Project of Chongqing Scientific Research Institutions (cstc2022jxjl20016).
Author Contributions: The authors confirm contribution to the paper as follows: study conception and design: Jinchen Cao, Tiantian Du; analysis and interpretation of results: Ruiqiong Wang; draft manuscript preparation: Jinchen Cao. All authors reviewed the results and approved the final version of the manuscript.
Availability of Data and Materials: Data will be available on reasonable request.
Conflicts of Interest: The authors declare that they have no conflicts of interest to report regarding the present study.
Supplementary Materials: The supplementary material is available online at https://doi.org/10.32604/fhmt.2024.047825.
References
1. Luo, P., Wang, X. Q., Gu, Y. A. (2010). Characterization of asphaltenes precipitated with three light alkanes under different experimental conditions. Fluid Phase Equilibria, 291(2), 103–110. [Google Scholar]
2. Aum, Y., Aum, P. T. P., da Silva, D. N. N., Braga, N. D., de Souza, C. D. R. et al. (2024). Effective removal of paraffin deposits using oil-in-water microemulsion systems. Fuel, 358, 130112. [Google Scholar]
3. Li, N., Mao, G. L., Shi, X. Z., Tian, S. W., Liu, Y. (2018). Advances in the research of polymeric pour point depressant for waxy crude oil. Journal of Dispersion Science and Technology, 39(8), 1165–1171. [Google Scholar]
4. Chala, G. T., Sulaiman, S. A., Japper-Jaafar, A. (2018). Flow start-up and transportation of waxy crude oil in pipelines—A review. Journal of Non-Newtonian Fluid Mechanics, 251, 69–87. [Google Scholar]
5. Ebrahimi, M., Willershausen, D., Ashaghi, K. S., Engel, L., Placido, L. et al. (2010). Investigations on the use of different ceramic membranes for efficient oil-field produced water treatment. Desalination, 250(3), 991–996. [Google Scholar]
6. Piroozian, A., Hemmati, M., Safari, M., Rahimi, A., Rahmani, O. et al. (2021). A mechanistic understanding of the water-in-heavy oil emulsion viscosity variation: Effect of asphaltene and wax migration. Colloids and Surfaces A: Physicochemical and Engineering Aspects, 608, 125604. [Google Scholar]
7. Zhai, S. B., Cao, G. S., Zuo, S. L., Bai, Y. J., Cheng, Q. C. et al. (2023). A molecular dynamics approach to revealing effect mechanism of asphaltene on wax deposition behavior. Journal of Dispersion Science and Technology, 2278490. https://doi.org/10.1080/01932691.2023.2278490 [Google Scholar] [CrossRef]
8. Zhu, H. R., Lei, Y., Li, C. X., Yao, B., Yang, F. et al. (2023). Experimental and mechanism investigation on flowability and wax deposition of waxy crude oil with dissolved CH4 by pressurized laboratory apparatus. Fuel, 343, 127907. [Google Scholar]
9. Haj-Shafiei, S., Workman, B., Trifkovic, M., Mehrotra, A. K. (2019). In-situ monitoring of paraffin wax crystal formation and growth. Crystal Growth & Design, 19(5), 2830–2837. [Google Scholar]
10. Zheng, S., Fogler, H. S. (2014). Fundamental investigation of wax diffusion characteristics in water-in-oil emulsion. Industrial & Engineering Chemistry Research, 54(16), 4420–4428. [Google Scholar]
11. Paso, K., Silset, A., Sorland, G., Goncalves, Marcelo de A. L., Sjoeblom, J. (2009). Characterization of the formation, flowability, and resolution of Brazilian crude oil emulsions. Energy & Fuels, 23(1), 471–480. [Google Scholar]
12. Li, Q., Liu, C., Zhang, Z. (2014). Prediction of solubility of sulfur in hydrogen sulfide based on molecular dynamics simulation. Asian Journal of Chemistry, 26(4), 1041–1043. [Google Scholar]
13. Zhao, Q. Y., Zheng, L. C., Dong, Y., Jin, H., Wang, Y. C. et al. (2023). Molecular dynamics simulation of heavy oil dissolution in supercritical water and multi-component thermal fluid. Sustainable Energy & Fuels, 7(17), 4094–4109. [Google Scholar]
14. Gan, Y., Cheng, Q., Chu, S., Wang, Z., Luan, G. et al. (2021). Molecular dynamics simulation of waxy crude oil multiphase system depositing and sticking on pipeline inner walls and the micro influence mechanism of surface physical-chemical characteristics. Energy & Fuels, 35(5), 4012–4028. [Google Scholar]
15. Li, Q., Deng, X., Liu, Y., Cheng, Q., Liu, C. (2021). Gelation of waxy crude oil system with ethylene-vinyl acetate on solid surface: A molecular dynamics study. Journal of Molecular Liquids, 331, 115816. [Google Scholar]
16. Wang, S., Zhang, L., Liu, C., Liu, Z., Lan, S. et al. (2021). Techno-economic-environmental evaluation of a combined cooling heating and power system for gas turbine waste heat recovery. Energy, 231, 120956. [Google Scholar]
17. San-Miguel, M. A., Rodger, P. M. (2003). Wax deposition onto FeO surfaces. Physical Chemistry Chemical Physics, 5(3), 575–581. [Google Scholar]
18. Jin, J. H. Y., Pak, A. J., Durumeric, A. E. P., Loose, T. D., Voth, G. A. (2022). Bottom-up coarse-graining: Principles and perspectives. Journal of Chemical Theory and Computation, 18(10), 5759–5791. [Google Scholar] [PubMed]
19. Español, P., Warren, P. B. (2017). Perspective: Dissipative particle dynamics. Journal of Chemical Physics, 146(15), 150901. [Google Scholar]
20. Bustamante-Rendón, R. A., Pérez, E., Gama Goicochea, A. (2020). Comparing the efficiency of pure and mixed cationic and nonionic surfactants used in enhanced oil recovery by mesoscopic simulations. Fuel, 277, 118287. [Google Scholar]
21. Wang, D., Zhu, Y. L., Zhao, Y., Li, C. Y., Mukhopadhyay, A. et al. (2020). Brownian diffusion of individual janus nanoparticles at water/oil interfaces. ACS Nano, 14(8), 10095–10103. [Google Scholar] [PubMed]
22. Moncayo-Riascos, I., de Leon, J., Garcia-Martinez, J. A., Garcia-Cruz, I., Lira-Galeana, C. (2019). Multiscale simulation of asphaltene deposition in pipeline flows. Journal of Petroleum Science and Engineering, 183, 106376. [Google Scholar]
23. McDonagh, J. L., Shkurti, A., Bray, D. J., Anderson, R. L., Pyzer-Knapp, E. O. (2019). Utilizing machine learning for efficient parameterization of coarse grained molecular force fields. Journal of Chemical Information and Modeling, 59(10), 4278–4288. [Google Scholar] [PubMed]
24. Zhang, S. F., Sun, L. L., Xu, J. B., Wu, H., Wen, H. (2010). Aggregate structure in heavy crude oil: Using a dissipative particle dynamics based mesoscale platform. Energy & Fuels, 24(8), 4312–4326. [Google Scholar]
25. Thompson, A. P., Aktulga, H. M., Berger, R., Bolintineanu, D. S., Brown, W. M. et al. (2022). LAMMPS-a flexible simulation tool for particle-based materials modeling at the atomic, meso, and continuum scales. Computer Physics Communications, 271, 08171. [Google Scholar]
26. Groot, R. D., Warren, P. B. (1998). Dissipative particle dynamics: Bridging the gap between atomistic and mesoscopic simulation. Journal of Chemical Physics, 107(11), 4423–4435. [Google Scholar]
27. Groot, R. D. (2003). Electrostatic interactions in dissipative particle dynamics-simulation of polyelectrolytes and anionic surfactants. Journal of Chemical Physics, 118(24), 11265–11277. [Google Scholar]
28. Bray, D. J., Anderson, R. L., Warren, P. B., Lewtas, K. (2020). Wax formation in linear and branched alkanes with dissipative particle dynamics. Journal of Chemical Theory and Computation, 16(11), 7109–7122. [Google Scholar] [PubMed]
29. Zhao, T. Y., Wang, X. G. (2013). Solvent effect on phase transition of lyotropic rigid-chain liquid crystal polymer studied by dissipative particle dynamics. Journal of Chemical Physics, 138(2), 024910. [Google Scholar] [PubMed]
30. Li, B. F., Guo, Z. Y., Li, N., Wang, D. X., Li, G. H. et al. (2023). Molecular dynamics simulation of wax deposition in crude oil systems. Colloids and Surfaces A: Physicochemical and Engineering Aspects, 674, 131965. [Google Scholar]
Cite This Article
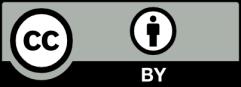
This work is licensed under a Creative Commons Attribution 4.0 International License , which permits unrestricted use, distribution, and reproduction in any medium, provided the original work is properly cited.