Open Access
ARTICLE
Effect of Nanomaterials Addition to Phase Change Materials on Heat Transfer in Solar Panels under Iraqi Atmospheric Conditions
1
Department of Power Mechanics, Haweja Technical Institute, Northern Technical University, Kirkuk, 36001, Iraq
2
Department of Reconstruction and Projects, Ministry of Higher Education and Scientific Research, Baghdad, 10065, Iraq
3
Department of Physics, College of Education, Al-Iraqia University, Baghdad, 10053, Iraq
4
Engineering Technical College of Mosul, Northern Technical University, Mosul, 41002, Iraq
* Corresponding Author: Omar Rafae Alomar. Email:
Frontiers in Heat and Mass Transfer 2023, 21, 215-226. https://doi.org/10.32604/fhmt.2023.041668
Received 01 May 2023; Accepted 30 May 2023; Issue published 30 November 2023
Abstract
It is common knowledge that phase-change materials are used for the purpose of thermal storage because of the characteristics that are exclusive to these materials and not found in others. These characteristics include a large capacity for absorbing heat and a large capacity for releasing heat when the phase changes; however, these materials have a low thermal conductivity. This paper presents the results of an experimental study that investigated the impact that nanoparticles of copper oxide had on reducing the temperature of solar panels. The phase change substance that was used was determined to be beeswax. The impact of adding nanoscale copper oxide at a concentration of 0.05% of the total mass of wax was investigated and compared to a reference solar panel that did not contain any nanoscale additions. The findings demonstrated that the incorporation of nanoscale copper oxide brought about a reduction of three °C in the plate’s average temperature as well as a one percent improvement in its electrical efficiency. In cases where it seems that the use of nanoparticles might potentially enhance the performance of integrated solar energy systems that contain phase change.Keywords
Nomenclature
PCM | Phase change material |
MW | Microcrystalline wax |
PW | Paraffin wax |
NPCMs | Nano-particles phase change materials |
TiO2 | Titanium oxide nanoparticle |
Al2O3 | Aluminum oxide |
CuO | Copper oxide |
Phase change material’s (PCM) is perfectly suited for energy storage as it can absorbs or releases a considerable amount of heat during the phase transition process. The metamorphic material’s flexibility is a characteristic of the pure phase alone, but it is constrained by poor thermal conductivity and rapid cooling. Elements have been added to certain qualities to enhance them and broaden their application. Nanomaterials are used to support its development and can enhance the phase change material’s thermal conductivity, heat capacity, and other features [1]. Experimental research has been done to determine how PCM combined with nanoparticles will perform. Different volume percentages of aluminum oxide (AL2O3) ranging from 0.11% to 0.77% are combined with PCM. Additionally, the cylindrical fins are employed to replicate photovoltaic solar cells by acting as a heat sink and thermal conductivity enhancer for the aluminum thermal plate. The frontal surface temperature is improved by 20%–46.3% when cylindrical fins with PCM are used, and increases to 52.3% when nanoparticles are used [2]. When the performance of microcrystalline wax (MW) as a phase change material was compared to paraffin wax (PW) supported with nano-alumina at different levels (0.5, 1 and 2 by weight%), the results showed a significant improvement (PW) supported with nano-alumina in terms of thermal conductivity and heat capacity at these endurance levels [3]. Using copper nanoparticles and molten trichloride that contained sodium chloride (15%), potassium chloride (45%), and lithium chloride (40%) by weight, a new energy storage PCM was created through an experimental investigation. According to the results of the thermal analysis, the combination of copper nanoparticles and trichloride salts greatly improves thermal conductivity and specific heat, while having no impact on latent heat of fusion, melting point, or freezing point. Particularly, it has been demonstrated that CuO nanoparticles with a mass fraction of 3% are more effective as enhancing heat transfer agents. High heat transfer efficiency, high storage density, and extraordinarily high-temperature cycle stability are all characteristics of phase change materials. The outcomes also supported the best practice of using nanoparticles with a mass fraction of 3% [4]. Four sets of phase-changing composite materials (PCMs) were used for this, each of which contained a single kind of nanoparticle. Where 0.5% by weight of nanoparticles (NPs) were added to myristic acid, which served as the base material Each group received a 0.5% weight of one of the following materials: aluminium oxide, silicon dioxide, copper oxide, or silver. The melting point of myristic acid is between 55.54°C and 55.94°C, and the solidification point is between 48.42°C and 48.71°C. By using a scattering of nanomaterials and a laser flicker analyzer, it was confirmed that myristic acid increased thermal conductivity. The study’s findings show that composite PCMs melt and solidify more quickly than pure PCMs [5]. Due to their exceptional qualities, carbon materials can be employed to enhance PCM properties. High thermal conductivity and superior adsorption capabilities can be found in carbon-based materials. The mechanical, electrical, and flame-retardant capabilities of PCMs can all be enhanced by certain carbon materials. The performance and applicability of two-dimensional carbon (graphite, graphene, and graphene oxide) are illustrated also [6]. To reduce energy consumption and industrial waste, an original eutectic mixture of paraffin, recycled SiNPs, and lauric and palmitic acids has been made for keeping thermal energy in buildings, fatty acids and paraffin are attractive options. However, it leaks, conducts heat poorly, and has weak mechanical strength. The addition of high-density polyethylene and sintered processing increased mechanical strength. According to microstructure studies, PCM can fill voids in frameworks with a maximum weight of 75% without leaking. Thermal testing revealed a material with a high capacity for the heat of 53.9–104.4 J/g and a low phase transition point of 33.1°C that is appropriate for building applications. The thermal conductivity of the recovered SiNPs increased by 300% as a result of PbO inclusion [7]. The impact of employing paraffin wax mixed with copper nanoparticles as a phase change material (PCM) was examined in relation to instillation potency. According to the experimental findings, using copper nanoparticles in coatings, reflectors, nanocoatings, reflector assemblies, and PCM with CuO nanoparticles running on the reference ones boosted the total freshwater yield of the billet distiller by 57%, 14%, 70.7%, and 108%. Each day, a total of 2,400 and 5,000 mL/m2 of fresh water were collected from traditional trailers and trays, respectively [8]. By integrating the nano-particles into PCM, the charge and discharge time of a PCM can be shortened the distillation with the aid of nano-particle enhanced PCM. The yields of various 0.1%, 0.2%, and 0.3% nano-particles were determined to be 440, 455, and 510 ml/0.25 m2 per day in an experimental investigation using paraffin wax coated with CuO nano-particles [9]. A new stable-phase transition material is created by combining silicon dioxide nanoparticles that improve thermal conductivity and stability, and the eutectic chlorides (MgCl, NaCl, and KCl). The compound’s heat capacity grew to 1.36 times in the solid phase and 1.63 times in the liquid form when compared carefully to pure trichloride, whilst the conductivity grew by 23.2 and 9.2 times, respectively, in both states. By using electron microscopy to analyze the pores, it was possible to identify a highly dense nanostructure that was uniformly distributed throughout them. This structure appears to be the cause of the material’s increased specific heat and thermal conductivity [10] synthesis of a thermal energy storage shape-stable nanocomposite (PCM) based on multi-walled carbon nanotubes and beeswax. Due of its high latent heat, beeswax was used as the PCM, and multi-walled carbon nano-tubes (MWCNTs) served as the thermally effective support substance. The three types of MWCNTs used in this experiment were untreated, ball-milled, as well as acid-treated. Beeswax and CNT samples were created in mixtures of 5 and 20 weight percent. Thermal performance tests, such as those for latent and sensitive heat, melting and hardening point, thermal conductivity, and up to 300 thermal cycles, were performed on the composite samples. Utilizing carbon nanotubes boosted the heat conductivity [11], the hydrothermal properties and adsorption rate were investigated using ethylene glycol as PCM. Solar energy conversion relies heavily on nanoscale phase transition materials, which are also used to improve thermal conduction behavior in thermal energy storage devices. Nanomaterials have a larger surface area and better thermal properties than larger particles. Both pond material yield and evaporation rate are improved by the addition of nano-particles phase change materials (NPCM). It has been shown that NPCM is more promising than PCM for Salt water desalination procedures. According to this study, paraffin composites are stable up to 160°C and enhance efficiency due to the improved thermal properties of NPCM [12]. The experimental investigation carried out to reveal the impact of silver nanoparticles on phase transition materials’ capacity on store thermal energy. The Paraffin wax as a phase change material and silver nanoparticles prepared at a ratio between 0.05% and 0.1% of the total mass. Paraffin wax’s melting and solidifying characteristics were investigated both with and without nanoparticles. According to the experiment findings, adding silver nanoparticles to paraffin wax decreased the phase change materials’ melting and solidification temperatures. The ability of a material to store thermal energy is improved when the concentration of silver nanoparticles in phase change materials increases [13]. Titanium oxide nanoparticle (TiO2) was added to the wax to evaluate the thermophysical changes that occur. Equally crucial is picking the appropriate materials to use in (PV/T) applications. Five samples were produced using nano-titanium oxide by mass% (0, 0.5, 1, 2, and 3). The results showed that introducing Nano-TiO2 at every concentration leads to a rise in density and viscosity. After the addition of 3% Nano-TiO2, the viscosity and density of paraffin wax increased by 9.5% and 5.5%, respectively. The thermal conductivity rose to 28.94%, 58.42%, 77.9%, and 91.57%, respectively, for mass fractions of 0.5%, 1%, 2%, and 3%. Additionally, Nano–TiO2 improves thermal stability by lessening the thermal breakdown of paraffin wax [14]. A polyaniline-cobalt nanocomposite encapsulated in a matrix was created to enhance the thermophysical characteristics of paraffin. Polyaniline-based nanocomposites with cobalt contents of 1.0 and 2.0 wt% (PC1 and PC2). The paraffin was sonicated at various weight ratios of 0.1%, 0.5%, 1%, and 5%. When PC1 and PC2 were added in increasing weight ratios, the thermal conductivity of paraffin reinforced with nanocomposites improved by up to 1% and dropped by 5% when paraffin was added. The thermogravimetric data showed that the early decomposition temperatures were considerably lower than those of the basic PCM paraffin with paraffin wax. In comparison to paraffin wax, 0.1% and 0.5% displayed latent heats of melting of 182.04 J/g & 170.86 J/g, respectively [15]. A photovoltaic system combining a conventional PV module and a PCM-cooled. The temperature of the cell is controlled by PCM and nanoscale PCM in photovoltaic systems (nono-PCM). Polyethylene glycol (PEG) 1000, which melts between 33°C and 39°C, was used to prepare the PCM. The PCM’s input materials were Al2O3 and silica nanoparticles. The results showed that the addition of PV-Alumina Nano PCM and Variable PV-Silica Nano Material Cooling System (SNPCM) improves the system’s power efficiency by 4.82%, 8.1%, and 7.17%, correspondingly, and boosts the energy output to 5.12%, 8.4%, and 7.29%. The temperature of the solar panels drops by 17.15% when compared to a solar system without cooling [16]. To increase the PCM’s conductivity and, thus, its rate of evolution, copper and aluminium oxide nanoparticle powder is added. Then, the temperature profiles and storage capabilities of the hybrid PCM and the pure PCM are compared. When 0.165% Cu nanoparticles are present, the thermal charge lifetime is reduced by 25.3%, and when 0.165%–0.816% Cu-Al2O3 hybrid nanoparticles are present, it is reduced by 10.8% [17]. When highly conductive nanoparticles migrate into phase change materials, the thermophysical properties of the resulting nanocomposites are improved (PCMs). The base matrix is mostly composed of palmitic acid and eutectic paraffin wax. A detailed uncertainty analysis was performed on each thermophysical parameter assessed. The composite nanocomposite has a maximum thermal conductivity of 0.59 W/m.K with 0.5% nanofillers’, which is 2.3 times more than the base value of 0.25 W/m.K. Test results showed that the composites had good solar transmittance (82%) due to the addition of nanomaterials with high refractive indices. The latent heat of NeUPCMs was increased by 17%, although the melting point of the nanocomposites only slightly decreased [18]. By functioning as a light absorber and combining modest amounts of carbon nanotubes, extending graphite is a restricted but effective technique to boost the capacity of EG-paraffin wax composite PCM to absorb solar energy (CNTs). Strong latent heat preservation (159 kJ/kg), excellent conductivity (2.05 W/m.K), and high solar absorption capacity are all characteristics of the composite PCMs produced with 5 and 2 wt% EG. Furthermore, these superior thermal and optical properties may be maintained even after 1,000 freeze-thaw cycles [19]. A new integrated thermal storage system (PEG-6000) was built using pure polyethylene glycol-6000 and (SA) gels with photothermal materials (CuS-CNTs). A three-dimensional plant nanostructure with exceptional photothermal conversion and optical absorption was the end result. CuS’s strong photo-thermal characteristics, a significant storage heat of about 150.8 J/g, and dependable thermal stability were all transferred to the hydrogel. Solar energy storage had a 91.4% efficiency [20]. In the current study, experimental investigation is conducted to reveal the impact of adding nanoparticles of copper oxide on reducing the temperature of solar panels. The phase change substance that was used was determined to be beeswax. The impact of adding nanoparticles copper oxide at a concentration of 0.05% of the total mass of wax on solar panels performance was investigated and compared to a reference solar panel without nanoparticles additions.
The experimental work has been conducted under real weather conditions of the city of Hawija in Northern Iraq (35°, 20.12N, 43°, 47.03E) in the summer of 2022 using two solar panels with a capacity of 50 watts. All the technical specifications of the solar panel used in the experiment are in Table 1. PCM was integrated into one of the two solar panels, while the other was PCM enhanced with nano-copper oxide placed side by side on an iron stand that can change the angle of fixation from the horizon. The inclination angle was set at an angle of 45 degrees from the horizon throughout the experiment period, This angle is used in photovoltaic solar collectors that are stationary and do not use solar trackers throughout the year in the study area. and current and voltage readings were taken for two consecutive days to determine the deviation in the readings for both current and voltage, and the deviation was less than one percent before conducting the experiments. Where natural beeswax (a phase-changing material) was employed since it is readily available, inexpensive, and a byproduct of the natural material (honey) product. The temperature at which beeswax transitions from a solid to a liquid makes it ideal for heat control applications in Iraq’s hot environment. where the natural wax was melted using a water bath and filtered from foreign materials by filtering it, so it becomes yellowish-white, as oxide was added nano CuO2 utilized in the study was created by Sky spring nanomaterial, Inc. (Houston, USA), and obtained from regional marketplaces. Due to its great thermal conductivity and inexpensive cost, the material was selected. with an average particle size of 40 nm and a purity of 99% with a weight of 0.05%. CuO nanoparticles were mixed with PCM in the liquid state, as shown in Fig. 1 for copper oxide nanoparticles. The volume of the container was calculated and thus the approximate weight of the wax was known, as the wax is weighed with a sensitive scale to measure the weight to determine the quantity. In addition, 0.05% of Nano-crystalline copper oxide. Then kept in the ultrasound unit for three and a half hours at 65°C until the samples were well combined and the colour of the beeswax had totally changed. To verify the distribution of nanoparticles in the melted beeswax, nanoparticles were introduced to the beeswax at weight ratios of 0.05%. Homogenously, preventing any sedimentation or agglomeration of nanoparticles until homogeneity is achieved. It is worth noting that the preparation process was carried out while the beeswax was in the liquid state. The back was covered with an aluminum sheet and tighten with a sealing material to prevent leakage when the wax melts.
Figure 1: Experimental test rig (a) Nanoparticles, (b) Nanofluid sample, (c) Collected particles, (d) Blender, (e) Solar panel, (f) Weather station
The container is made with dimensions of (46.5 × 67 × 3) cm and filled with beeswax melted by a water bath. Type K Thermocouples for Temperature Measurement distributed on the plates and the enclosure as shown in Fig. 2. All thermocouples are connected to an APPLENT AT4516 type data logger. Temperatures are recorded hourly, as well as environmental temperatures and wind speed using the meteorological station as shown in Fig. 1. The intensity of solar radiation that reaches the surface of the panel was measured by a solar meter at the same angle of inclination, as well as an electrical output (current and electrical voltage) every hour, as the experiments continued for two consecutive months (July and August) and one experiment was conducted per week as the experiment data was recorded.
Figure 2: Longitudinal section showing the components and installation of the thermocouple
3.1 Solar Radiation and Environmental Temperature
The weather was distinctly summer as shown in Fig. 3 with an average temperature of 39.17°C and ranged from 33.1 at 8:00 AM to 42 at 2:00 PM. As for the solar radiation reaching the face of the board, it ranged from 498 watts per square meter at 8:00 AM to 1070 watts per square meter at noon.
Figure 3: Environmental temperature and solar radiation intensity with time for August 12, 2022
The temperatures of a solar panel using phase-change material, both with and without copper oxide nanoparticles, are shown in Fig. 4. The panel temperatures with PCM without additives and PCM with copper oxide nanoparticles added are shown in the above figure. The temperature of the PCM solar panel containing 0.05% CuO3 nanoparticles ranged from 36.35°C–63.5°C, while that of the PCM solar panel with pure PCM ranged from 38°C–65.9°C. The solar panel with pure PCM had an average temperature of 53.165°C, but the solar panel with secondary copper oxide added to the PCM had an average temperature of 50.7°C. The pure PCM integrated board’s surface temperature rises from 38°C at 8:00 to 65.9°C at 1:00, then progressively drops until it reaches 45.65°C at 5:00 PM. At 8:00 AM in the morning, the temperature of the integrated PCM solar panel, which is strengthened with copper oxide nanoparticles, found to be 36.35°C. At 1:00 PM, it peaked at 63.5°C. After that, it progressively dropped until reaches 44.05°C at 5:00 PM. From 8:00 AM to 11:00 AM, the temperature between the two solar panels rises until reaches 3.9°C. This variation could be explained by the solar panel’s improved PCM with nanoparticles’ thermal conductivity. This high thermal conductivity speeds up the top layer of the PCM’s heat transfer to the following layer, lowers the temperature of the top layer of the PCM, lengthens the time it takes for the top and bottom layers to undergo phase changes, and minimises the risk of an excessive rise in the temperature of the PCM fluid. Along with the rise in solar radiation and the proportionate drop in morning air temperature. The temperature of the panel rises along with that of its surroundings as solar radiation increases; this could result in a reduction in the temperature difference between the two panels.
Figure 4: The temperature of the PCM solar panel without additives and the temperature of the panel with additives
The graph displays the amount of electricity generated by solar panels compact of a phase-changing material with and without copper oxide nanoparticles. The power output of the board using PCM with and without copper oxide nanoparticles is depicted in Fig. 5. The graphic makes it evident that the productivity of PCM solar panels without additives decreases more during noontime than it does when copper oxide nanoparticles are added, along with a significant voltage drop. In pure beeswax and beeswax with nano additives, the average daytime voltage was 17 and 17.5 volts, respectively, as a result of the increase in current, as depicted in Figs. 6 and 7. After 10:00 AM, it is evident that the voltage decrease outweighs the current rise. This can be attributed to the high temperature of the solar panel, which results from the reduction in thermal conductivity, as well as the high temperature of the surroundings, which prevents the exchange of heat between the panel and the surroundings, and the rise in solar radiation, which also raises the temperature. After 1:00, the relative temperature starts to drop along with the solar radiation. Another factor that may be useful is that as the temperature drops, the PCM starts to freeze due to the release of latent heat, which either raises the temperature of the panel or delays the decrease in the temperature of the solar panel.
Figure 5: Generated electricity from the PCM solar panel without additives and the solar panel with additives over time
Figure 6: The current generated from the PCM solar panel without additives and the solar panel with additives over time
Figure 7: The voltage generated from the PCM solar panel without additions and the solar panel with additions over time
Fig. 8 shows the electrical efficiency of a solar panel with phase change material without and with copper oxide nanoparticles. The figure above shows the electrical efficiency of a solar panel with PCM without additives and PCM with copper oxide nanoparticles added. Where the figure shows an increase in the electrical efficiency of the solar panel with PCM with the addition of nanoparticles of copper oxide in the noon hours, unlike the panel without tautological additives, during which the efficiency decreased due to the high temperatures of the panel, as it seems that the addition of nanoscale copper oxide led to an improvement in thermal conductivity as well as heat capacity where it was average efficiencies without and with nanomaterials are 13.2% and 14.1%, respectively.
Figure 8: The electrical efficiency of the PCM solar panel without additives and the solar panel with additives over time
The effect of nanoparticles on the performance of beeswax as a PCM material in an experimental way by weight of 0.05% of copper oxide nanoparticles. In terms of temperatures, the solar panels incorporate natural beeswax without and with the addition of nanoparticles. It was found that the addition of a concentration of copper oxide nanoparticles by 0.05% in PCM reduces the temperature rates compared to pure PCM. It is clear that the average temperature decreased by 3% and the efficiency increased by 1%, meaning that it enhances thermal conductivity and retains thermal energy for a longer period. This experimental work shows that phase change materials with copper oxide nanoparticles can be an effective technology to improve the performance of solar energy systems.
Funding Statement: The authors received no specific funding for this study.
Author Contributions: The authors confirm contribution to the paper as follows: study conception and design: Majid Ahmed Mohammed, Abdullah Talab Derea; data collection: Mohammed Yaseen Lafta; analysis and interpretation of results: Obed Majeed Ali; draft manuscript preparation: Omar Rafae Alomar. All authors reviewed the results and approved the final version of the manuscript.
Availability of Data and Materials: All data is available on request.
Conflicts of Interest: The authors declare that they have no conflicts of interest to report regarding the present study.
References
1. Abdelrahman, H. E., Wahba, M. H., Refaey, H. A., Moawad, M., Berbish, N. S. (2019). Performance enhancement of photovoltaic cells by changing configuration and using PCM (RT35HC) with nanoparticles Al2O3. Solar Energy, 177(9), 665–671. [Google Scholar]
2. Abdullah, A. S., Essa, F. A., Bacha, H. B., Omara, Z. M. (2020). Improving the trays solar still performance using reflectors and phase change material with nanoparticles. Journal of Energy Storage, 31, 101744. [Google Scholar]
3. Kalidasan, B., Pandey, A. K., Shahabuddin, S., George, M., Sharma, K. et al. (2021). Synthesis and characterization of conducting Polyaniline@cobalt-Paraffin wax nanocomposite as nano-phase change material: Enhanced thermophysical properties. Renewable Energy, 173(1), 1057–1069. [Google Scholar]
4. Behura, A., Gupta, H. K. (2019). Use of nanoparticle-embedded phase change material in solar still for productivity enhancement. Materails Today Proceedings, 45, 3904–3907. [Google Scholar]
5. Han, L., Zhang, X., Ji, J., Ma, K. (2022). Research progress on the influence of nano-additives on phase change materials. Journal of Energy Storage, 55(17), 105807. [Google Scholar]
6. Harikrishnan, S., Dhass, A. D., Oztop, H. F., Abu-Hamdeh, N. (2022). Measurement of thermophysical properties with nanomaterials on the Melting/Freezing characteristics of phase change material. Measurement, 199(8), 111477. [Google Scholar]
7. Jacob, J., Pandey, A. K., Rahim, N. A., Selvaraj, J., Paul, J. et al. (2022). Quantifying thermophysical properties, characterization, and thermal cycle testing of nano-enhanced organic eutectic phase change materials for thermal energy storage applications. Solar Energy Materials and Solar Cells, 248, 112008. [Google Scholar]
8. Li, M., Mu, B. (2019). Effect of different dimensional carbon materials on the properties and application of phase change materials: A review. Applied Energy, 242(1), 695–715. [Google Scholar]
9. Lin, J., Ouyang, Y., Chen, L., Wen, K., Li, Y. et al. (2022). Enhancing the solar absorption capacity of expanded graphite-paraffin wax composite phase change materials by introducing carbon nanotubes additives. Surfaces and Interfaces, 30, 101871. [Google Scholar]
10. Mahdi, M. T., Kara, I. H. (2021). Thermophysical characteristic of Nano-TiO2 paraffin wax composite material. Journal of Mechanical Engineering Research and Developments, 44, 48–58. [Google Scholar]
11. Mohamed, N. H., Soliman, F. S., El Maghraby, H., Moustfa, Y. M. (2017). Thermal conductivity enhancement of treated petroleum waxes, as phase change material, by α nano alumina: Energy storage. Renewable and Sustainable Energy Reviews, 70(3), 1052–1058. [Google Scholar]
12. Nemati, S., Pircheraghi, G. (2020). Fabrication of a form-stable phase change material with green fatty acid and recycled silica nanoparticles from spent lead-acid battery separators with enhanced thermal conductivity. Thermochimica Acta, 693, 178781. [Google Scholar]
13. Nitsas, M., Koronaki, I. P. (2021). Performance analysis of nanoparticles-enhanced PCM: An experimental approach. Thermal Science and Engineering Progress, 25(2), 100963. [Google Scholar]
14. Pradeep, N., Paramasivam, K., Rajesh, T., Subash Purusothamanan, V., Iyahraja, S. (2021). Silver nanoparticles for enhanced thermal energy storage of phase change materials. Materails Today Proceedings, 45(3), 607–611. [Google Scholar]
15. Putra, N., Rawi, S., Amin, M., Kusrini, E., Kosasih et al. (2019). Preparation of beeswax/multi-walled carbon nanotubes as novel shape-stable nanocomposite phase-change material for thermal energy storage. Journal of Energy Storage, 21, 32–39. [Google Scholar]
16. Sasilatha, T., Elavarasi, R., Karthikeyan, V. (2021). Influence of nano phase change materials on the desalination performance of double slope solar still. European Journal of Formal Sciences and Engineering, 5(1). [Google Scholar]
17. Sheik, M. A., Aravindan, M. K., Beemkumar, N., Chaurasiya, P. K., Jilte, R. et al. (2022). Investigation on the thermal management of solar photo voltaic cells cooled by phase change material. Journal of Energy Storage, 52(7), 104914. [Google Scholar]
18. Tan, J., Luo, S., Ji, W., Li, Y., Li, L. et al. (2022). Phase-changing hydrogels incorporated with copper sulfide-carbon nanotubes for smart thermal management and solar energy storage. Journal of Energy Storage, 50, 104653. [Google Scholar]
19. Wang, Q., Wu, C., Sun, S., Wang, X., Wu, S. et al. (2023). Comprehensive performance of composite phase change materials based on ternary eutectic chloride with CuO nanoparticles for thermal energy storage systems. Solar Energy, 250(4), 324–334. [Google Scholar]
20. Yu, Q., Zhang, C., Lu, Y., Kong, Q., Wei, H. et al. (2021). Comprehensive performance of composite phase change materials based on eutectic chloride with SiO2 nanoparticles and expanded graphite for thermal energy storage system. Renewable Energy, 172(3), 1120–1132. [Google Scholar]
Cite This Article
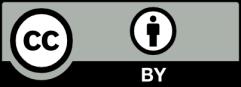
This work is licensed under a Creative Commons Attribution 4.0 International License , which permits unrestricted use, distribution, and reproduction in any medium, provided the original work is properly cited.