Open Access
ARTICLE
Energy Design and Optimization of Greenhouse by Natural Convection
Advanced Systems Engineering Laboratory (ASELab), National School of Applied Sciences, Ibn Tofail University, Kenitra, Morocco
* Corresponding Author: Aouatif Saad. Email:
(This article belongs to the Special Issue: Materials and Energy an Updated Image for 2023)
Fluid Dynamics & Materials Processing 2024, 20(8), 1903-1913. https://doi.org/10.32604/fdmp.2024.050557
Received 10 February 2024; Accepted 10 April 2024; Issue published 06 August 2024
Abstract
This study addresses the pressing need for energy-efficient greenhouse management by focusing on the innovative application of natural ventilation. The primary objective of this study is to evaluate various ventilation strategies to enhance energy efficiency and optimize crop production in agricultural greenhouses. Employing advanced numerical simulation tools, the study conducts a comprehensive assessment of natural ventilation’s effectiveness under real-world conditions. The results underscore the crucial role of the stack effect and strategic window positioning in greenhouse cooling, providing valuable insights for greenhouse designers. Our findings shed light on the significant benefits of optimized ventilation and also offer practical implications for improving greenhouse design, ensuring sustainable and efficient agricultural practices. The study demonstrated energy savings in cooling from November to April, with a maximum saving of 680 kWh in March, indicating the effectiveness of strategically positioning windows to leverage the stack effect. This approach enhances plant growth and reduces the need for costly cooling systems, thereby improving overall energy efficiency and lowering operational expenses.Graphic Abstract
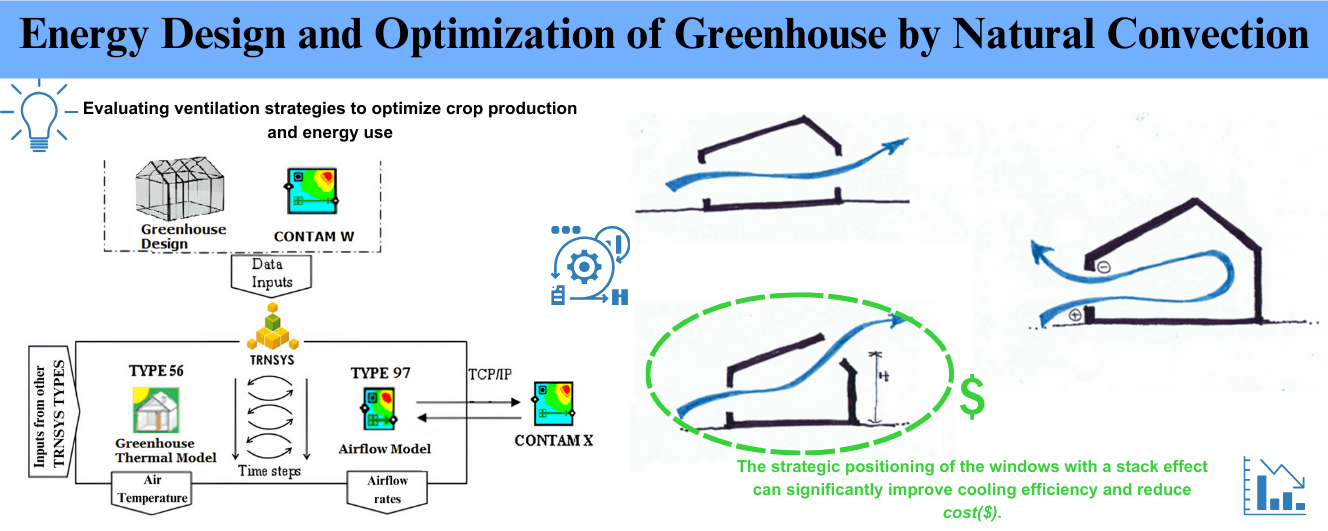
Keywords
Nomenclature
TRNSYS | Thermal Simulation System |
TRNBUILD | Thermal Balance Building Model in TRNSYS (TYPE 56) |
TYPE 56 | Building model type in TRNSYS |
TYPE 97 | Module in TRNSYS for communication with CONTAM |
CONTAM | A program for building airflow and contaminant transport simulations |
CONTAMW | User Interface for Visualization of Air Circulation Network Components in CONTAM |
CONTAMX | Numerical solver used in CONTAM |
TCP/IP | Transmission Control Protocol/Internet Protocol |
BUI | Building User Interface |
TMF | TRNSYS Model File |
TPF | TRNSYS Project File |
PRJ | Project File |
.AIR | Airflow Information File |
.B18 | Building File |
.OUT | Output File |
TCP/IP | Transmission Control Protocol/Internet Protocol |
Agriculture is pivotal to the global economy, and the role of greenhouse crop production is increasingly vital in meeting the surging demand for fresh produce. Effectively managing the internal climate of greenhouses, especially temperature and humidity, is crucial as these elements significantly influence plant growth. Our study focuses on natural ventilation as an effective strategy for cooling in agricultural greenhouses.
An extensive review of greenhouse design and cooling technologies indicates that a synergy of natural ventilation, evaporative cooling, and shading can substantially lower the energy requirements of greenhouses and optimize indoor conditions, thereby maximizing crop yields in hot climates [1]. Our goal is to enrich the understanding of various greenhouse ventilation strategies to assist in their design. This endeavor is driven by the need to fill gaps in existing literature concerning natural ventilation in greenhouse environments [2,3]. One study enhances this understanding by analyzing the impact of roof openings on heat transfer in tunnel greenhouses, emphasizing the importance of natural ventilation in managing internal climates [4].
While previous research has underscored the importance of ventilation openings and internal airflows in temperature control [5,6], it often falls short of addressing the entire spectrum of thermal dynamics and energy systems in greenhouses comprehensively. Another significant contribution in this area explores the thermal behavior and energy needs in specific climatic conditions, examining how design parameters like cladding material and orientation influence greenhouse efficiency [7].
Recent studies have furthered our understanding in this field, highlighting the complexities of greenhouse microclimates. For instance, research on the thermal behavior and energy efficiency of greenhouses presents novel insights into optimizing these environments for better crop production This study underscores the importance of energy-efficient control and modeling in greenhouses, emphasizing the need for comprehensive analysis of thermal behaviors [8].
In addition to this, advancements in the field expand our understanding of greenhouse microclimates and ventilation. Critical evaluations and studies [9,10] provide insights into the mechanics and models of natural ventilation, highlighting factors such as wind, buoyancy, and greenhouse geometry. They focus on the potential for natural ventilation under changing climate conditions in North America, the design of solar-powered forced ventilation systems, and the analysis of natural ventilation behavior in warm climates through 3D simulation. Additionally, further research [11] contributes to our understanding of ventilation behavior in Colombian greenhouses under warm climate conditions. The developments in microclimate monitoring devices [12], web-based greenhouse microclimate control automation systems [13], and comprehensive reviews of control methods and strategies [14], further augment the literature in this field.
Studies on the heterogeneous microclimate in typical greenhouses in Central China [15], the development of microclimate models for predicting temperatures in naturally ventilated greenhouses [16], and the formulation and validation of mathematical models of greenhouse microclimates [17] are crucial for our approach. The exploration of greenhouse environment modeling and simulation for microclimate control by [18,19], as well as the works on microclimate conditions in naturally ventilated greenhouses [20], and the optimization of greenhouse microclimate parameters [21] considering the impact of CO2 and light, make significant contributions.
In this research, we conduct an extensive evaluation of natural ventilation strategies using state-of-the-art simulation tools such as TRNSYS and CONTAM. We integrate advanced models to examine the complex interplay between various environmental factors and their impact on greenhouse climate control, exploring different scenarios within our simulations. By considering variables such as window positioning and the stack effect, we aim to derive optimized strategies for ventilation. This approach allows us to fill the existing research gap by considering the thermal and dynamic effects of the entire energy system, thus providing new insights into natural ventilation strategies for sustainable and economically viable greenhouse designs.
Our study not only advances theoretical understanding but also offers practical guidance for greenhouse design, emphasizing sustainable and energy-efficient practices in agricultural settings. By systematically analyzing different ventilation configurations and their effects on greenhouse climates, we aim to provide actionable insights that can significantly improve the efficiency and productivity of greenhouse operations. In summary, our research underscores the significance of considering a holistic view of greenhouse environments to develop effective natural ventilation strategies that support sustainable agriculture.
In evaluating various natural ventilation strategies for agricultural greenhouses, our study employs a robust numerical simulation model, integrating both thermal and airflow dynamics. The thermal model, managed using TRNSYS software [22], meticulously simulates heat transfer within the greenhouse, taking into account heat flux through the greenhouse’s structural components, as well as real-time solar radiation heat flux [23]. Complementarily, the airflow model facilitated by CONTAM [24] diverges from conventional complex Navier-Stokes equations, opting for a more balanced airflow approach [25].
In our integrated TRNSYS-CONTAM framework, TRNSYS handles the thermal simulations, calculating internal temperatures and heat fluxes across greenhouse surfaces. These thermal results are then seamlessly fed into CONTAM’s airflow balance model. The thermal data from TRNSYS significantly influences the airflow simulation in CONTAM, impacting natural convection and pressure differentials. The amalgamation of these models creates a dynamic feedback loop, refining the overall thermal model in each iteration [26].
CONTAM’s airflow model is crucial in simulating the impact of wind speed, direction, and pressure on natural ventilation, accounting for external factors like local weather conditions. This, in tandem with TRNSYS’s thermal model, allows for an effective evaluation of natural ventilation efficacy in greenhouses, integrating both thermal and aerodynamic aspects for a comprehensive understanding of natural ventilation efficiency [27].
2.1 Numerical Modeling Coupling TRNSYS-CONTAM
We used TRNSYS software to develop a building model (TYPE 56), enhanced with brand-new features, as shown in Fig. 1. This model employs thermal balance calculations to evaluate temperatures and amounts of heat at each time step, enabling accurate simulations of complex building systems. To facilitate communication and data exchange between the building model and the CONTAM program, a specific module of TRNSYS (TYPE 97) was developed. This module acts as a server for CONTAMX, the numerical solver of the program, allowing execution control and data transfer via TCP/IP sockets.
Figure 1: Simulation tools—related programs that interact with TRNSYS
The integration of the building model with ancillary programs, such as CONTAM, enabled us to perform detailed simulations of thermal performance in buildings and to evaluate the effectiveness of various ventilation strategies.
The mathematical coupling between TRNSYS and CONTAM is described by a set of differential equations representing the energy balance for TRNSYS and the airflow and contaminant transport for CONTAM. For TRNSYS (Type 56), the heat balance equation is utilized.
And for CONTAM (Type 97), the airflow network model equation might be represented as:
where
Finally, to create and visualize the representation of the air circulation network components, the user interface known as CONTAMW is utilized [29]. To edit the BUI file and input the necessary information for simulating the greenhouse, we used the TRNBuild input description manager. This input includes the description of the envelope, detailing materials, layer thicknesses, and thermophysical parameters.
Table 1 summarizes the input data and the main resulting files that comprise the project file and presents the linkage process used [28].
2.2 Simulation of the Greenhouse
Our study centers on a greenhouse in Kenitra, Morocco, noted for its Mediterranean climate that significantly influences the dynamics of greenhouse ventilation and temperature regulation. Kenitra’s geographic coordinates, 34.2610°N latitude and 6.5802°W longitude, along with its elevation, are critical in shaping local climate patterns. These specific geographic characteristics are essential for comprehending environmental interactions within the greenhouse.
The greenhouse we are examining is designed as an “even span” structure, a common design known for efficient space utilization and effective light distribution—advantages that are particularly beneficial in Kenitra’s climatic conditions, where sunlight exposure is crucial for crop growth and energy efficiency.
An in-depth analysis of Kenitra’s local climate conditions, which significantly impact the greenhouse’s internal environment, is a key aspect of our study. To accurately simulate these effects, we have gathered a comprehensive set of climatic data for Kenitra, focusing on temperature, wind, and sunlight patterns.
Simulating air circulation within the greenhouse requires detailed knowledge of its geometric characteristics, including size, shape, orientation, and the number and positioning of openings such as windows. Our greenhouse, measuring 5 m × 4 m × 3.5 m (L × W × H) with a glazing rate of 77% in double glazed argon and an aluminum frame, is modeled using TRNBuild software see Fig. 2.
Figure 2: “Even span” greenhouse
The configuration depicted in Fig. 3 showcases the precise interplay among the components that model the environmental conditions of the greenhouse. At the heart of this configuration is the simulation of energy transfer and airflow, driven by real-time meteorological data such as temperature, wind, atmospheric pressure, and solar radiation. The arrangement of the ‘airflow model’, ‘Weather’ module, and ‘GREENHOUSE’ block is meticulously tailored to emulate the actual conditions in Kenitra, providing a comprehensive view of thermal dynamics and ventilation efficiency. This computational modeling is critical for assessing the efficacy of natural ventilation strategies, enabling us to refine the placement of windows to leverage the stack effect for enhanced climate control within the greenhouse.
Figure 3: TRNSYS-CONTAM coupled simulation interface for greenhouse environmental modeling
We have developed a series of simulation scenarios to assess the impact of different parameters on air circulation and indoor temperature. Each scenario introduces various configurations of windows, each with a surface area of 0.8 m2, strategically placed on the side walls and roof of the greenhouse see Table 2. The orientation of the greenhouse and the window positions are precisely determined to enable a thorough analysis of air circulation patterns [30].
The outcomes of these simulations are vital for optimizing greenhouse design parameters, with the goal of enhancing energy performance and improving crop production efficiency. This holistic approach, considering the geographical setting, greenhouse design, and local climate conditions in Kenitra, provides a nuanced understanding of the environmental factors affecting greenhouse operations.
Our study investigates the impact of window positioning on internal climate control in greenhouses, emphasizing natural ventilation as a strategy to reduce reliance on artificial cooling during cooler months. The insights from our research are detailed through a series of figures which illustrate the thermal dynamics within the greenhouse, ensuring a clear visual understanding of the findings as the reader progresses through the document.
Our analysis considers external meteorological data as critical inputs, which include external temperature, wind speed, and solar radiation, as graphically represented in Fig. 4. The data, illustrated by the daily fluctuations on the graph, inform our understanding of the environmental factors that affect greenhouse conditions. This empirical evidence underpins the development of our natural ventilation strategies, acknowledging that each meteorological variable contributes to the overall climate control efficiency within the greenhouse setting. These findings are integral to the study, providing a basis for recommending design and operational adjustments aimed at optimizing natural ventilation.
Figure 4: Meteorological data from KENITRA for 10.11.12 January (Temp, wind speed, direction and solar radiation)
Temperature profiles within the greenhouse, crucial for climate management, are detailed in the study’s temperature distribution analysis. These profiles, critical in assessing the effectiveness of natural ventilation, are portrayed in the center of Fig. 5, The figure demonstrates how the strategic arrangement of windows, capitalizing on the stack effect, substantially cools the interior. In contrast to scenarios with closed windows “GH close” or those merely exposed to ambient temperatures, this configuration leads to significantly lower temperature peaks.
Figure 5: Indoor air temperature of the greenhouse as a function of window scenarios
Our analysis reveals that the stack effect exerts a more pronounced influence on temperature moderation than merely increasing window surface area. A design with just two windows, each contributing a stack effect of 0.8 m2, resulted in a greater temperature decrease than a larger aggregate window surface without utilizing the stack effect. This suggests that strategic window positioning is key to optimizing the greenhouse’s thermal condition.
Fig. 6, ‘Cooling Energy Savings Comparison,’ provides an annual comparative analysis of energy savings achieved by implementing the stack effect vs. maintaining a sealed greenhouse. From November to April, the graph highlights substantial energy savings with the stack effect, especially in March, where savings reached up to 680 kWh.
Figure 6: Stack effect vs. closed greenhouse: cooling energy savings
In summary, our research underscores the significance of the stack effect in natural ventilation strategies within greenhouses. By creating a detailed temperature profile and strategically placing windows to exploit this effect, we propose a sustainable model for greenhouse climate control, promising substantial reductions in energy consumption and operational expenses, paving the way for more sustainable agricultural practices.
Our investigation into natural ventilation within greenhouses has culminated in critical findings that elevate the role of such ventilation in bolstering energy efficiency and agricultural productivity. The study has brought to light the importance of thermal dynamics within greenhouse environments, including factors such as wind patterns, vent placement, and ambient temperatures—all pivotal to the optimization of natural ventilation strategies.
Our results, hinging on transient numerical simulation models, accentuate the significance of the stack effect in thermal regulation. This study demonstrates that the strategic placement of openings can significantly enhance the internal climate of greenhouses, leading to substantial energy savings—exemplified by a maximum saving of 680 kWh noted in March.
Comparatively, our innovative approach reveals a potential 56% energy consumption reduction during peak saving months, when contrasted with traditional designs relying on mechanical cooling systems. This advancement underscores not only the practicality and economic benefits of our strategy but also marks a novel contribution to the field of agricultural engineering.
However, this research is not without its limitations. The conclusions drawn are based upon a specific model under the unique climatic conditions of Kenitra, Morocco. Thus, the outcomes might differ when applied to different greenhouse structures and regional climates. The interplay between natural ventilation and other climate control systems also warrants further exploration to realize a holistic environmental management approach.
Looking forward, this study lays the groundwork for future explorations into greenhouse design, advocating for a multi-faceted approach that encompasses a wide variety of greenhouse configurations and climatic contexts. Subsequent studies should endeavor to integrate the dynamic interactions among all environmental control systems, aiming to develop comprehensive solutions that are universally applicable.
By forging a path toward more sustainable and cost-efficient agricultural practices, this research arms greenhouse designers and farmers with a deeper understanding and more robust tools for constructing advanced, environmentally friendly greenhouses. As we continue to refine these methods, the potential for transforming agricultural infrastructure becomes ever more tangible, guiding the industry toward greener and more economically viable solutions.
Acknowledgement: We would like to thank the Moroccan Ministry of Higher Education, Scientific Research and Innovation and the OCP Foundation who funded this work through the APRD Research Program.
Funding Statement: This project, named ‘SCAISAR’, was financed by the OCP Foundation. We express our sincere gratitude for their support and commitment to advancing research and development in this field.
Author Contributions: The authors confirm their contributions to the paper as follows: study conception and design were jointly developed by H. Benzzine and H. Labrim; data collection was led by H. Benzzine and D. Zejli; H. Benzzine and Y. Achour were responsible for the analysis and interpretation of results along with H. Labrim. The draft manuscript was prepared by D. Zejli and H. Benzzine, with revisions contributed by H. Labrim and R. El Bouayadi. A. Saad coordinated the study and contributed to the critical revisions of the manuscript. All authors reviewed the results and approved the final version of the manuscript.
Availability of Data and Materials: The data used generated and analyzed during this study are available from the first author upon reasonable request. Researchers interested in accessing the data can contact the first author directly to request the materials.
Conflicts of Interest: The authors declare that they have no conflicts of interest to report regarding the present study.
References
1. Ghoulem M, El Moueddeb K, Nehdi E, Boukhanouf R, Calautit JK. Greenhouse design and cooling technologies for sustainable food cultivation in hot climates: review of current practice and future status. Biosyst Eng. 2019 May 11;183:121–50. doi:10.1016/j.biosystemseng.2019.04.016. [Google Scholar] [CrossRef]
2. Kacira M, Sase S, Limi O. Effects of side vents and span numbers on wind-induced natural ventilation of a gothic multi-span greenhouse. Japan Agric Res Q. 2004 Oct 1;38:227–33. doi:10.6090/jarq.38.227. [Google Scholar] [CrossRef]
3. Liu S, Huang G. High-density block transformation to increase natural ventilation based on CFD simulation. Fluid Dyn Mater Proc. 2021;17(1):141–57. doi:10.32604/fdmp.2021.011990. [Google Scholar] [CrossRef]
4. Slatni Y, Djezzar M, Messai T. Numerical investigation of natural convection with heated tubes in tunnel greenhouse. J Therm Eng. 2021 May 1;7(4):731–45. doi:10.18186/thermal.915149. [Google Scholar] [CrossRef]
5. Evola G, Popov V. Computational analysis of wind driven natural ventilation in buildings. Energy Build. 2006 May;38(5):491–501. doi:10.1016/j.enbuild.2005.08.008. [Google Scholar] [CrossRef]
6. Jin M, Zuo W, Chen Q. Simulating natural ventilation in and around buildings by fast fluid dynamics. Numer Heat Transf Part Appl. 2013 Aug 15;64(4):273–89. doi:10.1080/10407782.2013.784131. [Google Scholar] [CrossRef]
7. Choab N, Allouhi A, El Maakoul A, Kousksou T, Saadeddine SAJ. Effect of greenhouse design parameters on the heating and cooling requirement of greenhouses in moroccan climatic conditions. IEEE Access. 2020 Dec 31;9:2986–3003. doi:10.1109/ACCESS.2020.3047851. [Google Scholar] [CrossRef]
8. Iddio E, Wang L, Thomas Y, McMorrow G, Denzer A. Energy efficient operation and modeling for greenhouses: a literature review. Renew Sustain Energy Rev. 2020 Jan 1;117:109480. doi:10.1016/j.rser.2019.109480. [Google Scholar] [CrossRef]
9. Gilani S, O’Brien W. Natural ventilation usability under climate change in Canada and the United States. Build Res Inf. 2021 May 19;49(4):367–86. doi:10.1080/09613218.2020.1760775. [Google Scholar] [CrossRef]
10. Revathi S, Sivakumaran N, Radhakrishnan TK. Design of solar-powered forced ventilation system and energy-efficient thermal comfort operation of greenhouse. Mater Today Proc. 2021 Jan 1;46:9893–900. doi:10.1016/j.matpr.2021.01.409. [Google Scholar] [CrossRef]
11. Villagran Munar E, Rodriguez Roa A. 3D Numerical analysis of the natural ventilation behavior in a Colombian greenhouse established in warm climate conditions. Sustainability. 2020 Oct 1;12:8101. doi:10.3390/su12198101. [Google Scholar] [CrossRef]
12. Negovanović S, Belingar A, Radojević M, Radičević B, Topisirović G. Improvement of the microclimate monitoring device in the greenhouse. Hung Agric Eng. 2019;35:26–33. doi:10.17676/HAE.2019.35.26. [Google Scholar] [CrossRef]
13. Hafiz M, Ardiansah I, Bafdal N. Website based greenhouse microclimate control automation system design. JOIN. 2020 Jun;5(1):105–14. doi:10.15575/join.v5i1.575. [Google Scholar] [CrossRef]
14. Xu ZF, Yin JJ, Ding J. Review on control methods and strategies of greenhouse microclimate. DEStech Trans Comput Sci Eng. 2019 May 20;133–41. doi:10.12783/dtcse/icaic2019/29415. [Google Scholar] [CrossRef]
15. Wang XW, Luo JY, Li XP. CFD based study of heterogeneous microclimate in a typical Chinese greenhouse in Central China. J Integr Agric. 2013 May 1;12(5):914–23. doi:10.1016/S2095-3119(13)60309-3. [Google Scholar] [CrossRef]
16. Singh MC, Singh JP, Singh KG. Development of a microclimate model for prediction of temperatures inside a naturally ventilated greenhouse under cucumber crop in soilless media. Comput Electron Agric. 2018 Nov 1;154:227–38. doi:10.1016/j.compag.2018.08.044. [Google Scholar] [CrossRef]
17. Singh G, Singh PP, Lubana PPS, Singh KG. Formulation and validation of a mathematical model of the microclimate of a greenhouse. Renew Energy. 2006 Aug 1;31(10):1541–60. doi:10.1016/j.renene.2005.07.011. [Google Scholar] [CrossRef]
18. Ma D, Carpenter N, Maki H, Rehman TU, Tuinstra MR, Jin J. Greenhouse environment modeling and simulation for microclimate control. Comput Electron Agric. 2019 Jul 1;162:134–42. doi:10.1016/j.compag.2019.04.013. [Google Scholar] [CrossRef]
19. Li A, Huang L, Zhang T. Field test and analysis of microclimate in naturally ventilated single-sloped greenhouses. Energy Build. 2017 Mar 1;138:479–89. doi:10.1016/j.enbuild.2016.12.047. [Google Scholar] [CrossRef]
20. Dewi VAK, Setiawan B, Waspodo R, Liyantono L. Microclimate condition in the natural ventilated greenhouse. J Tanah Dan Iklim. 2020 Jun 18;44:31. doi:10.21082/jti.v44n1.2020.31-36. [Google Scholar] [CrossRef]
21. Sokolov S. Optimization of greenhouse microclimate parametersconsidering the impact of CO2 and light. J Eng Sci. 2023;10(1):G14–21. doi:10.21272/jes.2023.10(1).g2. [Google Scholar] [CrossRef]
22. Klein SA, Beckman WA. TRNSYS 16: a transient system simulation program: mathematical reference. TRNSYS. 2007 Jan 1;5:389–96. [Google Scholar]
23. Sapounas A, Bartzanas T, Nikita-Martzopoulou C, Kittas C. Aspects of CFD modelling of a fan and pad evaporative cooling system in greenhouses. Int J Vent. 2008 Mar 1;6:379–88. [Google Scholar]
24. Dols WS, Polidoro B. CONTAM user guide and program documentation version 3.4. National Institute of Standards and Technology Technical Note 1887 Revision 1. doi:10.6028/NIST.TN.1887r1. [Google Scholar] [CrossRef]
25. Du J, Bansal P, Huang B. Simulation model of a greenhouse with a heat-pipe heating system. Appl Energy. 2012 May 1;93:268–76. doi:10.1016/j.apenergy.2011.12.069. [Google Scholar] [CrossRef]
26. Li Y, Sun F, Shi W, Liu X, Li T. Numerical simulation of ventilation performance in mushroom solar greenhouse design. Energies. 2022 Jan;15(16):5899. doi:10.3390/en15165899. [Google Scholar] [CrossRef]
27. Abdel-Ghany AM, Kozai T. Dynamic modeling of the environment in a naturally ventilated, fog-cooled greenhouse. Renew Energy. 2006 Aug;31(10):1521–39. doi:10.1016/j.renene.2005.07.013. [Google Scholar] [CrossRef]
28. Khalifa I, Gharbi Ernez L, Znouda E, Bouden C. Coupling TRNSYS 17 and CONTAM: simulation of a naturally ventilated double-skin façade. Adv Build Energy Res. 2015 Jul 3;9(2):293–304. doi:10.1080/17512549.2015.1050694. [Google Scholar] [CrossRef]
29. Dols WS, Wang L, Emmerich SJ, Polidoro BJ. Development and application of an updated whole-building coupled thermal, airflow and contaminant transport simulation program (TRNSYS/CONTAM). J Build Perform Simul. 2015 Sep 3;8(5):326–37. [Google Scholar]
30. Choab N, Allouhi A, Maakoul AE, Kousksou T, Saadeddine S, Jamil A. Effect of greenhouse design parameters on the heating and cooling requirement of greenhouses in moroccan climatic conditions. IEEE Access. 2021;9:2986–3003. doi:10.1109/ACCESS.2020.3047851. [Google Scholar] [CrossRef]
Cite This Article
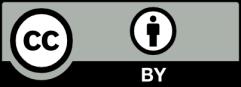
This work is licensed under a Creative Commons Attribution 4.0 International License , which permits unrestricted use, distribution, and reproduction in any medium, provided the original work is properly cited.