Open Access
ARTICLE
Effects of Different Concentrations of Sulfate Ions on Carbonate Crude Oil Desorption: Experimental Analysis and Molecular Simulation
School of Petroleum and Natural Gas Engineering, Changzhou University, Changzhou, 213164, China
* Corresponding Author: Nannan Liu. Email:
Fluid Dynamics & Materials Processing 2024, 20(8), 1731-1741. https://doi.org/10.32604/fdmp.2024.048354
Received 05 December 2023; Accepted 31 January 2024; Issue published 06 August 2024
Abstract
Low salinity water containing sulfate ions can significantly alter the surface wettability of carbonate rocks. Nevertheless, the impact of sulfate concentration on the desorption of oil film on the surface of carbonate rock is still unknown. This study examines the variations in the wettability of the surface of carbonate rocks in solutions containing varying amounts of sodium sulfate and pure water. The problem is addressed in the framework of molecular dynamics simulation (Material Studio software) and experiments. The experiment’s findings demonstrate that sodium sulfate can increase the rate at which oil moisture is turned into water moisture. The final contact angle is smaller than that of pure water. The results of the simulations show that many water molecules travel down the water channel under the influence of several powerful forces, including the electrostatic force, the van der Waals force and hydrogen bond, crowding out the oil molecules on the calcite’s surface and causing the oil film to separate. The relative concentration curve of water and oil molecules indicates that the separation rate of the oil film on the surface of calcite increases with the number of sulfate ions.Graphic Abstract
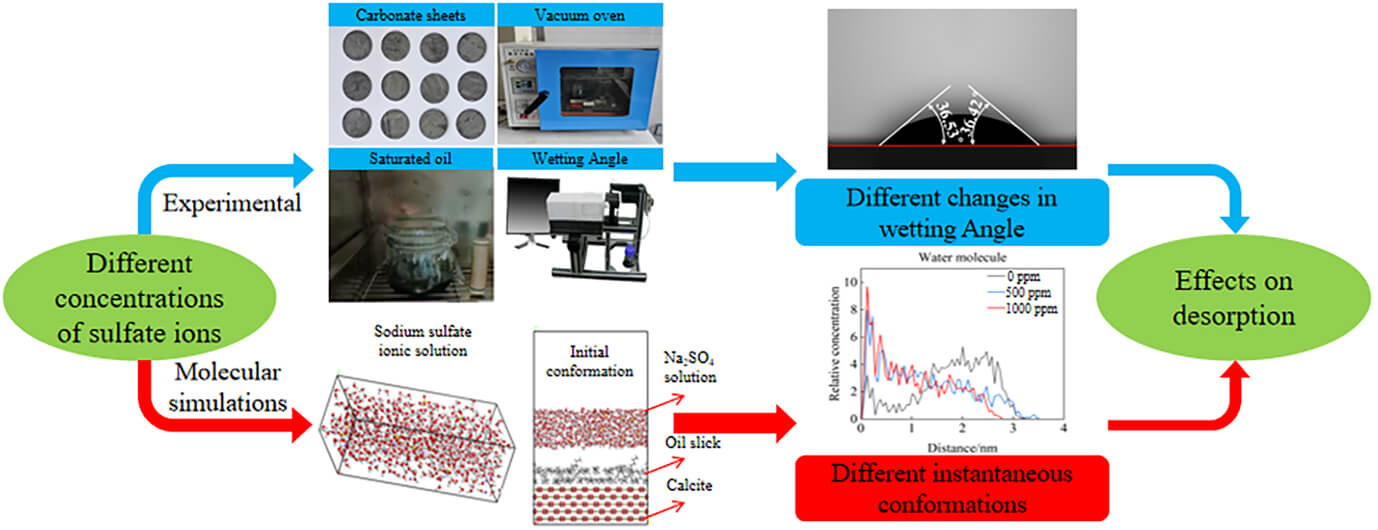
Keywords
In nature, supersaturated calcium bicarbonate solutions precipitate from water to form carbonate rocks. About 60% of the world’s remaining oil reserves are found in carbonate reservoirs [1], which are mostly composed of limestone and dolomite carbonate rock types [2,3], of which calcite mineral composition makes up a sizable share [4]. The increase in oil production from ordinary water flooding is typically minimal because carbonate reservoirs have high heterogeneity and strong oil wetness on the surface [5]. Therefore, to increase oil production, we consider modifying the composition of water flooding and using a low-salinity solution to displace the remaining oil in the reservoir.
People’s interest has grown in low salinity water flooding as a novel experimental technique to improve oil recovery. It is possible to convert an oil-wet reservoir into a water-wet one and increase the displacement efficiency of the residual oil by adjusting the ion type, concentration, and charge quantity of injected water in rock reservoirs [6–8]. Salt deposition will occur during water injection, which reduces the permeability of the rock [9]. The key elements influencing the displacement of crude oil are Cl−, SO42− ions in low salinity water and Ca2+, and Mg2+ ions in carbonate rocks [10]. When sulfate-containing solutions are used instead of traditional water flooding, carbonate rocks’ wettability can be altered, becoming wetter in the process [11]. The wettability of carbonate reservoirs can be greatly altered and oil recovery can be much enhanced by increasing the concentration of SO42− [12,13]. It is evident that SO42− affects the wettability of carbonate reservoirs; nevertheless, it is yet unknown how various sulfate ion concentrations will behave microscopic when it comes to oil film desorption and the alteration of wettability on the surface of oil-wet carbonate rocks.
The molecular dynamics simulation (MDS) application system is currently developing at an increasingly advanced rate [14,15]. It is capable of characterizing the forces that interact between various complex systems at the molecular level [16,17], such as the electrostatic, Coulomb, and van der Waals forces, among others, and providing a detailed description of the dynamics, energy, relative concentration, and other features of complex systems [18] The process of oil film adsorption and desorption on the surface of carbonate rocks can be simulated using molecular dynamics. From a molecular perspective, the impact of sodium sulfate concentrations on the surface wettability of carbonate rock was examined.
In this paper, carbonate rocks were used as the research object, and the treated thin slices were immersed in formation crude oil to form oil film. The wettability angle of the carbonate sheet in pure water and different concentrations of Na2SO4 solution at different times was measured. The Amorphous Cell (AC) module in the Material Studio software was used to establish boxes of pure water and sodium sulfate solutions of different concentrations, and the Material Dynamic (MD) module was used for molecular dynamics simulation. The effects of desorption and wettability of oil film on the surface of carbonate rock with different concentrations of sulfate were explained. The idea and method are provided for sulfate solution production of the carbonate reservoir, and it is convenient to optimize sulfate injection parameters. The effect of sulfate concentration on oil production is discussed from the microscopic point of view of molecular simulation. In the future, simulation can be combined with experiments and field production to improve the recovery of carbonate reservoirs.
The carbonate core slices used in the experiment were from Shengli Oilfield (Shandong, China). The detailed rock properties were obtained by an X-ray diffractometer. The light crude oil with a density of 0.83 g/cm3 and a viscosity of 60 cp at ambient temperature was acquired from a heavy oil reservoir (15 MPa, 80°C) in Shengli Oilfield, Shandong, China. Anhydrous sodium sulfate (AR) and n-heptane (AR) are provided by Sinopharm Chemical Reagent Co., Ltd. (Shanghai, China). X-ray diffractometer (XRD) was purchased from Dandong Tongda Technology Company (Dandong, China). The vacuum drying oven is produced by Huyue Instrument Equipment Factory (Shanghai, China). The contact Angle measuring instrument is provided by Shanghai Zhongchen Digital Technology Equipment Co., Ltd. (Shanghai, China) and deionized water.
2.2.1 Oil Wet Surface Formation
(1) Prepare a total of 12 carbonate flakes. Six of them are for experiments and the rest for backup. Polishing test core sheets with fine sandpaper, washing and drying with deionized water to ensure that the surface is free of any contaminants;
(2) Dip test sheets in light crude oil and place them in a drying oven at 60°C;
(3) The slices were taken out every other day, washed quickly with n-heptane (C7H16), and then dried to determine the surface wetting Angle of the slices using a contact angle measuring instrument.
2.2.2 Wetting Angle Experiment
(1) Sodium sulfate (Na2SO4) solution with 500 and 1000 ppm salinity and pure water at room temperature, the reagent used is analytically pure [19–21];
(2) The 6 slices of oil-wet carbonate rock were divided into 3 parts, each with 2 pieces, and soaked in different concentrations of sodium sulfate solution and pure water for 0, 6, 12, 24, 36, 48, 60 and 72 h. The slices were taken out and the wettability angles of oil-wet carbonate slices in different solutions and at different times were measured by a contact angle measuring instrument;
(3) The wettability of rock can be reflected by the contact angle. Usually, the contact angle formed by the liquid-solid interface is measured and determined geometrically. The conventional wetting angle is classified as water wet: 0°~75°, neutral wetting: 75°~115°, oil wet: 115°~180° [22,23].
3.1 Software Simulation and Modules
The Forcite module of the simulation software Material Studio 2017 was used to calculate the molecular interaction force and energy and analyze the adsorption and desorption process of oil film on the surface of calcite. The COMPASS II force field of the module was used to set the charge of different ions, and the motion of several single molecules and their condensed state were accurately predicted by van der Waals force, electrostatic force, and hydrogen bond interaction [24–26]. The Amorphous Cell (AC) module of the simulation software of Material Studio 2017 adopts the Monte Carlo method to build an amorphous model, such as a polymer blending model and solution model of various components and different proportions [27].
(1) Based on the sodium sulfate salt solution used in the experiment (500 ppm), Amorphous Cell (AC) module is used to construct the box containing 700 water molecules, 20 Na+ ions, and 10 SO42− ions salt solution [28], as shown in Fig. 1a;
(2) Place the salt solution box above the oil film, and add a 3 nm vacuum layer to eliminate the effect of periodic boundary, as shown in Fig. 1b. To demonstrate the effectiveness of the SO42− ion, a pure water solution box composed of 700 water molecules was compared;
(3) The number of sulfate ions in sodium sulfate solution was changed. Construct the box containing 700 water molecules, 32 Na+ ions, and 16 SO42− ions salt solution, and the simulation results were compared and analyzed;
(4) It used the steepest descent method to perform geometric optimization, obtain the optimal structural model, and simulate molecular dynamics relaxation (T = 298.15 K, t = 2 ns) under the canonical ensemble (NVT) [29,30]. The structure diagram of pure water and Sodium sulfate solution at the same time was recorded. The overall process of molecular simulation is shown in Fig. 2.
Figure 1: Initial model of oil film desorption (a) Initial confirmation of sodium sulfate ionicsolution box. (b) Initial conformation
Figure 2: Molecular simulation flow chart
The detailed rock properties obtained by an X-ray diffractometer as shown in Fig. 3. The composition of carbonate slices is mostly CaCO3. As shown in Fig. 4, the wetting Angle of the wafer gradually increased, and the growth rate was fast. After the seventh day, the wetting Angle increment was small and gradually stabilized. The wetting angle changed from the initial 22.36° to 117.28°, indicating that the surface of the carbonatite became oil-wet.
Figure 3: XRD mineral composition analysis diagram
Figure 4: Wetting angle over time
Fig. 5 shows the variation of the wetting angle of the carbonate sheet with time in pure water and sodium sulfate (500 and 1000 ppm) solution. Both pure water and sodium sulfate solution reduced the wetting Angle of the carbonate sheet surface, and the sheet changed from oil-wet to water-wet.
Figure 5: Change of surface wetting Angle of carbonate sheet with time in solution of different concentration
However, in the same period, the wetting angle of the wafer soaked in sodium sulfate solution changed greatly as shown in Figs. 5a and 5b. Finally, the surface wetting angle of the wafer soaked in sodium sulfate solution was smaller than that of the wafer soaked in pure water (θNa2SO4 = 49.35°, θH2O = 77.45°). It can be seen that the ions in sodium sulfate solution play a major role in the surface wettability change of the wafer. By changing the concentration of sodium sulfate solution, the salinity of sodium sulfate in the solution increased from 500 to 1000 ppm, we found that the wetting angle changes faster and the final wetting angle is smaller (θNa2SO4 (1000 ppm) = 36.46°). The higher the concentration of sodium sulfate solution, the more obvious effect on the wetting angle, as shown in Fig. 5c.
Figs. 6–8 show the simulation of oil film desorption using molecular simulation software. It can be seen from the figure that the oil film gradually separated from the calcite surface. Comparing Figs. 6 and 7, we found that SO42− ions in solution can accelerate the oil film desorption on the surface of calcite. As shown by the relative concentration curve in Fig. 7, the relative concentration of water molecules near the calcite surface increases over time and gradually presents a hierarchical pattern, while the relative concentration of oil molecules gradually decreases. When the equilibrium state is reached, the relative concentration of water molecules near the surface reaches the maximum value, while the relative concentration of oil molecules is 0, indicating that the oil film has completely separated from the calcite surface and is replaced by water film molecules, which further proves that the wettability of calcite surface changes from oil-wet to water wet. Under the strong hydrogen bond between water molecules and the surface of calcite, the density of water molecules on the surface of calcite gradually increases and spreads along the surface of calcite, and water molecules form water film on the surface of calcite. The water film thickens with the increase in the concentration of water molecules. Under the repulsive force of the water film, the oil molecules finally break away from the calcite surface, and the balanced case shows that the calcite surface will change from oil-wet to water-wet.
Figure 6: Molecular simulation of pure water solution
Figure 7: Molecular simulation of 500 ppm sulfate solution
Figure 8: Molecular simulation of 1000 ppm sulfate solution
Compared with Figs. 7 and 8, as the number of SO42− ions increases, a large number of water molecules move faster toward the surface of the calcite at the same time, and the time for the water layer to break through the oil film is shorter. The oil film is released from the surface of carbonate rock faster. It can be seen from the relative concentration curve that the concentration of water molecules from the rock surface changes faster and more intensively. The 1000 ppm sodium sulfate solution makes the oil film desorption faster and achieves ensemble equilibrium earlier.
Fig. 9 shows the simulation results of simultaneous water molecular motion and oil film desorption when the salinity of SO42− ions is 0, 500, and 1000 ppm. It can be seen that water molecules move downward faster with the movement of SO42− ions, and gather on the surface of calcite after tearing the oil film, and the desorption effect of the oil film is faster. It can be seen from the relative concentration curve that the concentration of water molecules near the rock surface is larger in the solution of high-concentration sodium sulfate at the same time, so the surface oil molecules decrease more and the oil film desorption is achieved.
Figure 9: Comparison of instantaneous system conformation and relative concentration
Based on experiments and molecular simulation, wettability change on the oil-wet calcite surface and the effects of different concentrations of sodium sulfate solution on oil film desorption were studied. The important role of sulfate ions in oil film desorption was more directly reflected from the molecular scale.
(1) When the carbonate rocks were immersed in light crude oil, the oil film on its surface gradually formed with a wettability angle of 117°. With the increase of immersing time in Na2SO4 solution, the surface of carbonate slices gradually changed from oil-wet to water-wet (49°). The rate and degree of wetting Angle change also increase with the increase of solution concentration and the final wetting angle is 36°. This is because the reduction of sulfate concentration is conducive to the formation of a thicker water film so that the hydrophilicity of carbonate rock is enhanced, the crude oil is more easily removed from the rock surface, and the oil washing efficiency is improved.
(2) A large number of water molecules broke through the oil film and formed water channels and then adsorbed on the surface of calcite. With the increase in the number of sulfate ions, the speed of breaking through the oil film and the formation of water channels will be accelerated. Under some force including electrostatic force, van der Waals force, and hydrogen bond, a large number of water molecules accumulated and spread on the surface of calcite along the channel formed, crowding out the oil molecules on the surface of calcite and forming oil film desorption. Sulfate ions played an important role in the surface wettability alternation of carbonate rocks. Under the electrostatic action, hydrated sulfate ions and water molecules pass through the oil layer to form a water channel between the water phase and the calcite surface. Under the action of hydrogen bonding, water molecules adsorb on the surface of calcite to form a water film, the contact line between the oil film and calcite shrinks gradually, and the oil molecules gather gradually. Under the repulsive force of the water film, the oil molecules finally break away from the calcite surface, and the calcite surface changes from oil-wet to water-wet.
Acknowledgement: The authors thank the anonymous reviewers for their constructive and valuable opinions gratefully.
Funding Statement: The research was supported by CNPC-CZU Innovation Alliance, and the Research Start-Up Fund of Changzhou University.
Author Contributions: Nannan Liu: Writing-original draft, Investigation, Conceptualization, Methodology, Supervision, Validation, Funding acquisition. Hengchen Qi: Writing-review & editing, Software, Investigation, Data curation. Hui Xu: Software, Investigation, Supervision, Visualization. Yanfeng He: Funding acquisition.
Availability of Data and Materials: The data are not publicly available due to restrictions (e.g., their containing information that could compromise the privacy of research participants).
Conflicts of Interest: The authors declare that they have no conflicts of interest to report regarding the present study.
References
1. Shams K, Sidqi AA, Shirish P, Mohamed M, Muhammad SK, Muhammad SK, et al. Adsorption reduction of a gemini Gemini surfactant on carbonate rocks using formic acid: static and dynamic conditions. Fuel. 2023;128166:345. [Google Scholar]
2. Julius T, Bai SH, Jan K, Mohammad P. Surfactant-induced wettability reversal on oil-wet calcite surfaces: experimentation and molecular dynamics simulations with scaled-charges. J Colloid Interface Sci. 2022;69:890–900. [Google Scholar]
3. Wang HJ. New approach to the thermal properties of carbonate rocks in geothermal reservoirs: molecular dynamics calculation and case studies. Renew Energ. 2022;198:861–87. [Google Scholar]
4. Rodrigo N, Paulo JR, Carlos RA. Multi-scale approach to assess total porosity and pore size in four different kinds of carbonate rocks. Micron. 2023;164:103385. [Google Scholar]
5. Pal S, Mushtaq M, Banat F, Sumaiti AMA. Review of surfactant-assisted chemical enhanced oil recovery for carbonate reservoirs: challenges and future perspectives. Pet Sci. 2018;15:77–102. [Google Scholar]
6. Joel TT, Patrick VB, Reza BG. Review of low salinity waterflooding in carbonate rocks: mechanisms, investigation techniques, and future directions. Adv Colloid Interf Sci. 2020;284:102253. [Google Scholar]
7. Mokhtari R, Anabaraonye BU, Afrough A, Mohammad-khani S, Feilberg KL. Experimental investigation of low salinity water-flooding in tight chalk oil reservoirs. J Petrol Sci Eng. 2022;208:109282. [Google Scholar]
8. Kalantariasl A, Tale F, Parsaei R, Keshavarz A, Jahanbakhsh A, Mercedes MM, et al. Optimum salinity/composition for low salinity water injection in carbonate rocks: a geochemical modeling approach. J Mol Liq. 2022;362:119754. [Google Scholar]
9. Khormali A, Ahmadi S. Prediction of barium sulfate precipitation in dynamic tube blocking tests and its inhibition for waterflooding application using response surface methodology. J Pet Explor Prod Technol. 2023;13(11):2267–81. [Google Scholar]
10. Negahdari Z, Khandoozi S, Ghaedi M, Malayeri MR. Optimization of injection water composition during low salinity water flooding in carbonate rocks: a numerical simulation study. J Pet Sci Eng. 2022;209:109847. [Google Scholar]
11. Moustafa EAAA, Shedid AS. Effects of magnesium and potassium sulfates on oil recovery by water flooding. Egypt. 2017;27:649–56. [Google Scholar]
12. Javad G, Siavash R. Effects of salinity and ionic composition of smart water on mineral scaling in carbonate reservoirs during water flooding. Pet Explor Dev. 2021;48:421–9. [Google Scholar]
13. Zhang P, Austad T. Wettability and oil recovery from carbonates: effects of temperature and potential determining ions. Colloid Surface A. 2006;279:179–87. [Google Scholar]
14. Yu T, Li Q, Tan YS, Xu L. Molecular dynamics simulation of CO2-N dissolution and stripping of oil films on pore walls based on intermolecular interaction energy. Chem Eng Sci. 2022;262:118044. [Google Scholar]
15. Liu N, Ju BS, Yang Y, Brantson TS, Wang J, Tian YP. Experimental study of different factors on dynamic characteristics of dispersed bubbles rising motion behavior in liquid-saturated porous media. J Petrol Sci Eng. 2019;180:396–405. [Google Scholar]
16. Liu N, Chen XL, Ju BS, Chen XL, He YF, Yang Y, et al. Microbubbles generation by an orifice spraying method in a water-gas dispersion flooding system for enhanced oil recovery. J Petrol Sci Eng. 2021;198:108196. [Google Scholar]
17. Azmi NSM, Bakar NFA, Mohd TAT, Azizi A. Molecular dynamics simulation on CO2 foam system with addition of SiO2 nanoparticles at various sodium dodecyl sulfate (SDS) concentrations and elevated temperatures for enhanced oil recovery (EOR) application. Comp Mater Sci. 2020;184:109937. [Google Scholar]
18. Parra JG, Domínguez H, Aray Y, Iza P, Zarate X, Schott E. Structural and interfacial properties of the CO2-in-water foams prepared with sodium dodecyl sulfate (SDSa molecular dynamics simulation study. Colloid Surf A. 2019;578:123615. [Google Scholar]
19. Zhang MS, Jin ZH. Molecular simulation on CO2 adsorption in partially water-saturated kaolinite nanopores in relation to carbon geological sequestration. Chem Eng J. 2022;450:138002. [Google Scholar]
20. Fang TM, Zhang YN, Ma R, Yan YG, Dai CL, Zhang J. Oil extraction mechanism in CO2 flooding from rough surface: molecular dynamics simulation. Appl Surf Sci. 2019;494:80–6. [Google Scholar]
21. Yu T, Li Q, Li XC, Hu HX, Tan YS, Xu L. Synergistic effects of CO2 density and salinity on the wetting behavior of formation water on sandstone surfaces: molecular dynamics simulation. J Nat Gas Sci Eng. 2022;105:104714. [Google Scholar]
22. Tetteh J, Kubelka J, Piri M. Effect of oil carboxylate hydrophobicity on calcite wettability and its reversal by cationic surfactants: an experimental and molecular dynamics simulation investigation. J Mol Liq. 2023;380:121663. [Google Scholar]
23. Liu ZL, Wang N, Li YX, Li X, Shi D, He WH, et al. Charge-modulated calcite surface for anionic surfactant adsorption from molecular dynamics simulations. Surf Interf. 2022;33:102234. [Google Scholar]
24. Spera MBM, Braga FN, Bartolomeu RAC, Economou IG, Franco LFM. Diffusion of fluids confined in carbonate minerals: a molecular dynamics simulation study for carbon dioxide and methane-ethane mixture within calcite. Fuel. 2022;325:124800. [Google Scholar]
25. Tang Y, Sadan K, Yin WZ. Surface chemistry of magnesite and calcite flotation and molecular dynamics simulation of their cetyl phosphate adsorption. Colloid Surf A. 2020;603:125246. [Google Scholar]
26. Yuan SD, Wang SY, Wang XY, Guo MM, Wang YD, Wang DS. Molecular dynamics simulation of oil detachment from calcite surface in aqueous surfactant solution. Comput Theor Chem. 2016;1092:82–9. [Google Scholar]
27. Wang SM, Zhou GG, Ma Y, Gao L, Song RR, Jiang GC, et al. Molecular dynamics investigation on the adsorption behaviors of H2O, CO2, CH4 and N2 gases on calcite (1 1¯0) surface. Appl Surf Sci. 2016;385:616–21. [Google Scholar]
28. Nada H, Nishimura T, Sakamoto T, Kato T. Heterogeneous growth of calcite at aragonite {001}- and vaterite {001}-melt interfaces: a molecular dynamics simulation study. J Cryst Growth. 2016;450:148–59. [Google Scholar]
29. Shi WY, Ding C, Yan JL, Han XY, Lv ZM, Lei W, et al. Molecular dynamics simulation for interaction of PESA and acrylic copolymers with calcite crystal surfaces. Desalinat. 2012;291:8–14. [Google Scholar]
30. Qin Z, Buehler MJ. Nonlinear viscous water at nanoporous two-dimensional interfaces resists high-speed flow through cooperativity. Nano Lett. 2015;15:3939–44. [Google Scholar] [PubMed]
Cite This Article
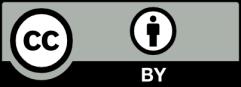
This work is licensed under a Creative Commons Attribution 4.0 International License , which permits unrestricted use, distribution, and reproduction in any medium, provided the original work is properly cited.