Open Access
ARTICLE
Influence of Polyaluminum Chloride Residue on the Strength and Microstructure of Cement-Based Materials
1 School of Civil Engineering, Henan Polytechnic University, International Joint Research Laboratory of Henan Province for Underground Space Development and Disaster Prevention, Jiaozuo, 454003, China
2 China Construction Seventh Engineering Division Co., Ltd., Zhengzhou, 450004, China
3 China Construction Seventh Engineering Division Co., Ltd., General Contracting Company, Zhengzhou, 450004, China
* Corresponding Author: Ping Xu. Email:
Fluid Dynamics & Materials Processing 2024, 20(6), 1299-1312. https://doi.org/10.32604/fdmp.2023.046183
Received 21 September 2023; Accepted 15 December 2023; Issue published 27 June 2024
Abstract
In this paper, cement and dechlorinated Polyaluminum Chloride Residue (PACR) have been used to prepare a net slurry and mortar specimens. Two hydration activity indicators have been used to quantitatively analyze the dechlorinated PACR hydration activity. In particular, the effect of dechlorinated PACR content on the compressive strength of mortar has been assessed by means of compressive strength tests. Moreover, X-ray diffraction (XRD) and scanning electron microscopy (SEM) have been employed to observe the microstructure of the considered hydration products. The following results have been obtained. The 28th day activity index of the dechlorinated PACR is 75%, and therefore it meets the criterion for the use of active admixture. The increase in the content of the dechlorinated PACR tends to reduce the compressive strength of mortar specimens, however, it is beneficial to its later strength growth. When the content is not greater than 10%, the strength remains unchanged, otherwise, it decreases. The PACR does not form a new crystalline phase in the cement slurry, and the dechlorinated PACR remains active until the age of the 28th day. The inclusion of the PACR mainly deteriorates the early strength of the cement slurry, but it promotes the production of hydration products in the cement slurry after the 7th day.Keywords
The incorporation of mineral admixtures and industrial by-product minerals into concretes offers several advantages, including the efficient utilization of industrial solid waste and a reduction in cement consumption. This leads to a decrease in the heat generated during cement hydration and an overall improvement in concrete quality [1]. Mineral admixtures such as fly ash, silica fume, slag, and limestone powder have been widely used in concrete products, effectively enhancing concrete performance and yielding positive social and economic outcomes [2–4]. However, the distribution and properties of mineral admixtures in concrete vary due to geographical and industrial factors, making the development and utilization of new industrial solid waste concrete admixtures and the performance of existing admixtures crucial focal points within the industry.
Polyaluminum chloride (PAC) is a widely recognized high-quality water purifying agent both domestically and internationally [5,6]. The manufacturing process involves the reaction of calcined bauxite, calcium aluminate powder, and hydrochloric acid to produce liquid polyaluminum chloride, with the resulting precipitated solid forming a waste residue primarily composed of SiO2, Al2O3, CaO, and so on. Due to the increasing demand for water purifiers in urban wastewater treatment, the stock of PACR has been increasing, leading to environmental pollution when the residue is landfilled, particularly due to its acidic nature. However, through water washing and low-temperature calcination for dechlorination, the dechlorinated PACR transforms into metakaolin [7,8], a material similar to high iron and high titanium, holding potential as active admixture in cement-based materials. Modified waste residue can also enhance sludge dewatering performance [9] and be utilized in the production of baking-free bricks [10]. Although the existing research has tested and analyzed the chemical composition of PACR [11] and demonstrated its potential activity, in-depth research on the hydration activity of polyaluminum chloride and its influence on cement-based products remains limited. The hydration activity of PACR is a critical factor that determines the strength of cement-based products [12–14], necessitating a systematic investigation into PACR’s hydration activity and strength, as well as its impact on the microstructure of cement-based materials.
In order to utilize industrial waste effectively and cleanly, this paper explores the possibility of PACR as an auxiliary cementitious material. Firstly, PACR undergoes pretreatment, and its chemical composition is analyzed. Subsequently, composite cementitious materials composed of ordinary silicate cement and dechlorinated PACR are used to prepare net slurry specimens and mortar specimens. The effects of dechlorinated PACR on the hydration characteristics of composite cementitious materials are analyzed through compressive strength tests, scanning electron microscopy (SEM), and X-ray diffraction (XRD). Additionally, an experimental study on the activity index of PACR is carried out by comparing mortar specimens with an equal amount of fly ash replacing cement as the control group. This helps elucidate the mechanism of dechlorinated PACR’s action in the composite cementitious system, cement-dechlorinated PACR, and provides evidence for the feasibility of PACR as a cementitious admixture. The results suggest that PACR can be applied to cement-containing building materials such as mortar, bricks, and concrete. Finally, by determining the optimal amount of PACR as an admixture, this research lays the foundation for future large-scale utilization of PACR.
2 Test Materials and Test Design
2.1 Test Materials and Components
The specific materials used in this test encompass P.O 42.5 ordinary silicate cement, ISO standard sand, and river sand. The ISO standard sand serves as a reference for the strength comparison activity index test. The river sand, on the other hand, is utilized for the mortar strength test, after being sieved with only particles below 1.18 mm retained. The density of the river sand is 2.58 g/cm3, with a fineness modulus of 2.4. Furthermore, the test incorporates dechlorinated PACR, which is derived from industrial processes involving water washing, dichlorination treatment, and low-temperature calcination of waste materials. The resulting polymeric aluminum chloride water purification agent is subsequently crushed and ground to form a brownish-yellow powder. It possesses a neutral pH and a density of 2.23 g/cm3.
2.1.2 Dechlorinated Polyaluminum Chloride Residue
The PACR test involves a series of steps. First, PACR is mixed with quicklime at a mass ratio of 20:1 and stirred to ensure even distribution. Next, water is added to create a slurry, which is stirred for 30 min. The slurry is then left to settle for 15 min, during which time natural precipitation occurs, resulting in supernatant and waste residue. Once the precipitation is complete, the supernatant is carefully removed, and the waste residue undergoes low-temperature calcination and drying at 150°C–200°C. This process aims to eliminate chloride ions and acids, as the supernatant contains high levels of CaCl2 and Ca(OH)2. Finally, after undergoing procedures such as crushing, screening, grinding, and other procedures by ball mills, the lumpy waste residue is reduced to particles ranging from 0–0.075 mm, resulting in dechlorinated PACR. This dechlorinated PACR is illustrated in Fig. 1.
Figure 1: 0–0.075 mm dechlorinated PACR
Table 1 presents the main chemical components of dechlorinated PACR and cement. It is evident that the dechlorinated PACR has a significant composition of SiO2 and Al2O3, and after the dichlorination process, the chloride ion content is minimal, making it suitable for use as raw materials in cement-based building materials. Fig. 2 illustrates the analysis of crystal composition, revealing the presence of crystalline phases such as SiO2, Al2O3, CaCO3, and CaTiO3 in the dechlorinated PACR. The diffraction peak of SiO2 is the most obvious. The reactivity of silicon depends on its phase form, whether crystalline or non-crystalline. Generally, higher silicon content leads to increased reactivity of volcanic ash after calcination [15–17]. The dechlorinated PACR prepared in this study underwent a chemical composition test, and the results are displayed in Fig. 2. The treatment process involving low-temperature calcination and subsequent procedures suggest the potential reactivity of dechlorinated PACR. Furthermore, the alkaline environment generated during cement hydration may trigger a reaction with the PACR due to its volcanic ash properties.
Figure 2: XRD diffraction pattern of the dechlorinated PACR
2.2.1 Polyaluminum Chloride Residue Total Activity Test
The test was conducted following the standard industrial waste activity test method, maintaining a fixed water-binder ratio of 0.5. Initially, the dechlorinated PACR in the 0–0.075 mm size range was mixed with cement at a mass ratio of 3:7. This combination was then used as the cementing material to produce mortar specimens (H1). To compare the activity level of PACR with that of an active admixture fly ash, first-grade fly ash and cement were mixed at a mass ratio of 3:7 to create cementitious material for mortar specimens (FA). The mortar ratios for each group can be found in Table 2, and the prepared mortar underwent standard maintenance. Compressive strength measurements were taken at different ages, and the resulting values were utilized in the admixture activity index equation to calculate the PACR’s activity level.
In this study, the hydration activity of dechlorinated PACR was assessed using two indices: the compressive strength activity index
The compressive strength activity index
To evaluate the effect of the dechlorinated PACR on the compressive strength of the specimens, the mineral admixture activity index evaluation method proposed by Pu [22] was employed. The hydration activity contribution of the admixture
Here,
2.2.2 Effect of Polyaluminum Chloride Residue Content on Compressive Strength of Cement Mortar
The cement mortar specimens were prepared using the 0–0.075 mm dechlorinated PACR and a fixed water-cement ratio of 1.0, as outlined in Table 3. Five groups of specimens were configured, each with a different residue content. The dechlorinated PACR replaced varying percentages of the cement content in the mortar specimens, namely 0%, 5%, 10%, 15%, and 20% by mass. Each group consisted of three specimens, labelled as PM1, PM2, PM3, PM4, and PM5. The specimen size was 70.7 mm × 70.7 mm × 70.7 mm. After pouring, the specimens were placed in a standard curing box with a temperature of 20°C ± 2°C and a relative humidity exceeding 90%. Following 24-h curing period, the specimens were demolded and numbered accordingly. Subsequently, the specimens were further cured under the standard environmental conditions, and the compressive strength test corresponding to each age was conducted.
To investigate the correlation between the activity, hydration products, micromorphological characteristics, and strength of the dechlorinated PACR cement-based composite slurry, various experimental procedures were conducted using pure slurry specimens with different PACR content. The specific ratios used in these specimens are provided in Table 4. Firstly, X-ray diffraction (XRD) analysis was used to observe the hydration products of the pure slurry specimens, both from the reference group and those containing different PACR content, on the 7th and 28th days. This allowed for the examination of the relationship between activity, hydration products, and PACR content. Secondly, scanning electron microscopy (SEM) was employed to study the microstructure of the cement mortar specimens. This involved comparing the reference group specimens with those containing the 0–0.075 mm dechlorinated PACR. Furthermore, SEM was employed to investigate the effect of dechlorinated PACR content on hydration products and the microstructure of cement-based materials. In particular, the pure slurry specimens with 5% and 20% PACR were examined using SEM. However, before conducting the microscopic tests, it was necessary to wait until the specimens were cured to the age of 7th day and 28th day. Specimens with diameters of 5–10 mm were taken from the center of the mortar specimens, stored in glass jars with anhydrous ethanol, and sealed to prevent the continuation of the hydration process. The mortar specimens were then taken out and air-dried before the SEM test to facilitate the examination.
3.1 Hydration Activity of the Dechlorinated Polyaluminum Chloride Residue
According to the results of the compressive strength test, substituting into Eq. (1), the compressive strength activity index A of different specimens can be calculated, and the findings are tabulated in Table 5.
The compressive strength activity index of the dechlorinated PACR at the same age is lower than that of fly ash, indicating that the dechlorinated PACR is a weak active admixture. However, the 28th day activity index of the dechlorinated PACR is 75% according to Table 5, exceeding the critical value of 65% specified in national standards [23] for a volcanic ash activity index. This suggests that the dechlorinated PACR can still be utilized as an active admixture in cement. The activity index of the dechlorinated PACR and fly ash increases with the growth of hydration age.
The contribution of the hydration activity of the dechlorinated PACR with respect to its age was determined by calculating the values of Eqs. (2)–(5) using the compressive strength test results obtained from each specimen at various ages. These calculations were then utilized to create graphs showcasing the relationship between the hydration activity contribution rate and the age of the dechlorinated PACR, as depicted in Fig. 3.
Figure 3: Hydration activity contribution rate of the dechlorinated PACR
Fig. 3 demonstrates the relationship between the contribution rate of FA hydration activity and age. It shows a gradual increase in the contribution rate with age. The contribution of dechlorinated PACR to hydration activity, however, initially had a negative impact until the 7th day. This suggests that under these conditions, the presence of dechlorinated PACR had some adverse effect on slurry properties. With the exception of the 7th day, the contribution of dechlorinated PAC sludge to hydration activity gradually increased with age, although the rate of increase slowed down as the age progressed. This behavior can be attributed to the decrease in cement and calcium ion content in the slurry due to the replacement of cement with dechlorinated PACR. Additionally, before the 7th day, the low hydration degree of cement led to low alkalinity in the slurry, which did not stimulate the activity of dechlorinated PACR. Furthermore, as an inert admixture, dechlorinated PACR does not undergo a volcanic ash reaction. As the age increased, the hydration degree deepened, resulting in higher alkalinity and Ca(OH)2 content in the slurry. This stimulated the activity of dechlorinated PACR, resulting in an increased contribution to hydration activity.
3.2 Influence of Polyaluminum Chloride Residue Content on Compressive Strength
The test results are illustrated in Fig. 4 and Table 6, which involved the design of five groups of cement mortars. The 0–0.0.75 mm dechlorinated PACR was used to replace cement in equal amounts at mass percentages of 0%, 5%, 10%, 15%, and 20%.
Figure 4: Compressive strength of 0–0.0.75 mm dechlorinated PACR mortar specimens with different contents
Fig. 4 illustrates that the 7th day compressive strength of dechlorinated PACR cement mortar gradually decreases as the PACR content increases. However, for contents below 10% (PM2 and PM3 groups), the 28th day compressive strength of dechlorinated PACR cement mortar remains comparable to that of the PM1 group without dechlorinated PACR. Moreover, as indicated in Table 6, when the PACR content increases from 15% to 20% (PM4 and PM5 groups), the average 7th day compressive strength of dechlorinated PACR cement mortar experiences the most significant decrease, measuring 1.7 MPa. Conversely, the average 28th day compressive strength of these two groups of specimens shows a lesser decline, with a decrease of 0.9 MPa. Notably, there is even a slight increase in strength after substituting 5% dechlorinated PACR for cement. This suggests that PACR primarily influences the early strength of mortar and reacts with cement hydration products during later stages, resulting in the formation of new hydration products and improved strength on the 28th day. Consequently, when the content of dechlorinated PACR remains below 10%, the strength of cement mortar remains relatively stable on the 28th day.
3.3 Hydration Activity of Dechlorinated Polyaluminum Chloride Residue
The results of XRD analysis for cement slurry specimens are presented in Fig. 5. The specimens include pure cement net slurry as well as slurry mixed with 5%, 10%, 15%, and 20% dechlorinated PACR. The analysis was conducted at 7 days and 28 days of age.
Figure 5: XRD spectrum of cement pure slurry specimen
Fig. 5 shows that the hydration products of pure slurry specimens remain consistent regardless of the addition of dechlorinated PACR. These products mainly consist of Ca(OH)2, calcium carbonate (CaCO3), tricalcium silicate (Ca3SiO5), and a small amount of ettringite (AFt). The chromatogram of specimens mixed with dechlorinated PACR at 28 days reveals a diffraction peak of SiO2, indicating the presence of this crystal phase introduced directly by the active substance of dechlorinated PACR after precipitation. It is evident that dechlorinated PACR does not generate new crystal phases in the slurry. Comparing the hydration products of net slurry specimens with and without PAC slag doping, there is no significant difference observed in the main peak of Ca(OH)2 and the characteristic peak of Ca3SiO5 in the hydration product at 7 days. However, at 28 days, these peaks weaken. This phenomenon is attributed to the low substitution rate of slag replacing cement and the reduction of cement content in the system, resulting in minimal changes in the crystalline phase. Additionally, the insufficient release of PACR activity at 7 days and the volcanic ash reaction consuming a portion of Ca(OH)2 with increasing age contribute to these changes. When comparing net slurry specimens with different dosages of PACR, the waste slag dosage varies (0%, 10%, 20%). By observing the characteristic peaks of CaCO3 and Ca3SiO5 in the hydration products of 7 days and 28 days, it can be seen that the enhancement of the hydration products of 28 days is obvious, and at the same time, the characteristic peaks of SiO2 are clearly enhanced. However, in the 28 days plots, it can be seen that the hydration products of the three groups of net slurry specimens with different dosages of waste slag are essentially the same. This finding indicates that the addition of PACR admixture does not substantially alter the hydration reactants of cement, but only has a marginal effect on the rate of hydration.
Electron microscopy was employed to analyze the internal microstructure of mortar specimens from two groups: the H0 group (specimens without dechlorinated PACR) and the H1 group (specimens with 0–0.075 mm dechlorinated PACR). Scans were conducted on the 7th and 28th days, and the results are displayed in Fig. 6.
Figure 6: Comparison of microstructure between cement mortar specimen and dechlorinated PACR cement mortar specimen
The existing studies have indicated that the microscopic morphology of cement hydration products, specifically C-S-H gels, typically exhibit spiny-like, needle-like, column-like, and network-like gel particles. Ettringite (AFt) is predominantly needle-like [24,25]. From Figs. 6a and 6b, it is apparent that on the 7th day, a large number of “network-like” C-S-H gels and some “needle-like” AFt were observed in the baseline group H0. The hydration products were interlaced, and the overall structure was dense. In contrast, in group H1, which was mixed with the dechlorinated PACR, a small number of C-S-H gels were observed, showing cluster radial growth. The microstructure of the H1 group contained more pores, generated fewer hydration products compared to the H0 group during the same period, and appeared looser due to the accumulation of unreacted dechlorinated PACR particles. Analysis of Figs. 6c and 6d a reveals that on the 28th day, the H0 group exhibited a dense skeleton attributed to the encapsulation of AFt by the settled C-S-H gel. Microporosity was reduced. On the other hand, the microstructure of the H1 group contained more pores, and the presence of these pores offered less sufficient support from AFt generation. In comparison to the H0 group during the same period, the growth of hydration products in the H1 group was more pronounced, accompanied by the formation of a C-S-H gel matrix and inter-pore AFt. This indicates that as the hydration reaction progresses, the dechlorinated PACR activity is gradually released, and a volcanic ash reaction occurs, promoting the generation of hydration products in the cement-dechlorinated PACR composite cementitious system.
To investigate the impact of chlorinated PACR content on hydration products and microstructure in cement-based systems, SEM was utilized to observe the micro-morphology of pure slurry specimens with 5% (P2) and 20% (P5) dechlorinated PACR. The results are presented in Fig. 7.
Figure 7: Comparison of microstructure between cement mortar specimen and dechlorinated PACR cement mortar specimen
Upon comparing (a) and (b) in Fig. 7, it is evident that on the 7th day, the P2 specimen exhibited a significantly higher amount of hydration products compared to the P5 specimen. The P2 specimen contained numerous needle rod-like AFt structures within the pores. This finding aligns with a study conducted by Liu et al. [26], which suggests that when weak active admixture is used instead of cement, it fails to stimulate activity due to insufficient alkalinity. In the P5 specimen, PACR particles accumulated, and although some hydration products were present on the surface of the waste residue particles, their integrity was poor. Furthermore, several pores and cracks were observed between the structures, indicating insufficient alkalinity in the slurry. Consequently, the hydration reaction was reduced, leading to fewer hydration products to fill the pores of slurry on the 7th day.
Examining Figs. 7c and 7d reveals that on the 28th day, the P2 specimen contained a considerable amount of columnar C-S-H gel, partially laminated sheet Ca(OH)2 crystals, and acicular AFt. These findings indicate a compact structure within the slurry. In contrast, the unreacted PACR particles disappeared in the P5 specimen, which exhibited abundant hydration products with tightly bonded structures. This outcome can be attributed to the increased hydration degree and alkalinity of the slurry as it aged. When comparing cement specimens with 5% and 20% PACR at the same age, it is evident that the latter still contained a significant number of pores within the microstructure and exhibited poor microstructural integrity. This explains why the compressive strength of PACR cement mortar with 20% PACR content at the same age is lower than that of PACR mortar with 5% PACR content.
Through various test methods such as the PACR composition test, PACR activity index test, the effect of PACR content on compressive strength of cement mortar, and microscopic examination, the collected data will be organized and analyzed. Based on the obtained results, the following key findings can be made:
1. The dechlorinated PACR exhibits a low strength activity index in the early stages. However, with increasing age, the activity index gradually improves, reaching 75% on the 28th day. When compared to fly ash, dechlorinated PACR behaves as an admixture with weak activity, as its strength activity index is lower.
2. As the content of dechlorinated PACR increases, the compressive strength of the dechlorinated PACR-cement mortar decreases. The addition of PACR mainly deteriorates the early strength of the mortar. When the PACR content is small (not more than 10%), the 28th day compressive strength of the mortar remains relatively unchanged.
3. The dechlorinated PACR does not introduce new crystalline phases in the cement paste. On the 7th day, the reaction degree of the dechlorinated PACR is low, while the hydration cementation products increase significantly by the 28th day of age. This indicates that PACR contributes to the later strength growth of the cement paste.
4. With an increase in dechlorinated PACR dosage, the early hydration products of cement slurry reduce, leading to an increase in structural porosity and an impact on mortar strength. However, as the hydration age progresses, the internal structure of the slurry undergoes significant improvement. Notably, the dosage of PACR in cement mortar should not exceed 10%.
Acknowledgement: Financial supports from Henan Science and Technology Key Project, Research on Key Technology and Performance of Polyaluminum Chloride Residue (PACR) Concrete Preparation (202102310253) and National Natural Science Foundation Project “Carbonization Strengthening of Recycled Coarse Aggregate and Its Influence on Mechanical Properties of Recycled Concrete Materials and Structures” (U1904188) are gratefully appreciated.
Funding Statement: Henan Science and Technology Key Project, Research on Key Technology and Performance of Polyaluminum Chloride Residue (PACR) Concrete Preparation (202102310253) and National Natural Science Foundation Project “Carbonization Strengthening of Recycled Coarse Aggregate and Its Influence on Mechanical Properties of Recycled Concrete Materials and Structures” (U1904188).
Author Contributions: The authors confirm contribution to the paper as follows: study conception and design: Ping Xu, Zhiwei Zhang; data collection: Zhenguo Hou; analysis and interpretation of results: Ping Xu, Zhiwei Zhang, Mankui Zheng; draft manuscript preparation: Jin Tong, Zhiwei Zhang. All authors reviewed the results and approved the final version of the manuscript.
Availability of Data and Materials: The data and materials used in the current study are available from the corresponding author on reasonable request.
Conflicts of Interest: The authors declare that they have no conflicts of interest to report regarding the present study.
References
1. Aprianti, E. (2017). A huge number of artificial waste material can be supplementary cementitious material (SCM) for concrete production-A review part II. Journal of Cleaner Production, 142, 4178–4194. https://doi.org/10.1016/j.jclepro.2015.12.115 [Google Scholar] [CrossRef]
2. Akid, A. S. M., Hossain, S., Munshi, M. I. U., Elahi, M. M. A., Sobuz, M. H. R. et al. (2021). Assessing the influence of fly ash and polypropylene fiber on fresh, mechanical and durability properties of concrete. Journal of King Saud University-Engineering Sciences, 35(7), 474–484. https://doi.org/10.1016/j.jksues.2021.06.005 [Google Scholar] [CrossRef]
3. Wetzel, A., Middendorf, B. (2019). Influence of silica fume on properties of fresh and hardened ultra-high performance concrete based on alkali-activated slag. Cement and Concrete Composites, 100, 53–59. https://doi.org/10.1016/j.cemconcomp.2019.03.023 [Google Scholar] [CrossRef]
4. Ramezanianpour, A. A., Ghiasvand, E., Nickseresht, I., Mahdikhani, M., Moodi, F. (2009). Influence of various amounts of limestone powder on performance of Portland limestone cement concretes. Cement and Concrete Composites, 31(10), 715–720. https://doi.org/10.1016/j.cemconcomp.2009.08.003 [Google Scholar] [CrossRef]
5. Matsui, Y., Shirasaki, N., Yamaguchi, T., Kondo, K., Machida, K. et al. (2017). Characteristics and components of poly-aluminum chloride coagulants that enhance arsenate removal by coagulation: Detailed analysis of aluminum species. Water Research, 118, 177–186. https://doi.org/10.1016/j.watres.2017.04.037. [Google Scholar] [PubMed] [CrossRef]
6. Mohamed, A. Y. A., Siggins, A., Healy, M. G., Ó hUallacháin, D., Fenton, O. et al. (2020). Appraisal and ranking of poly-aluminium chloride, ferric chloride and alum for the treatment of dairy soiled water. Journal of Environmental Management, 267, 110567. https://doi.org/10.1016/j.jenvman.2020.110567. [Google Scholar] [PubMed] [CrossRef]
7. Raheem, A. A., Abdulwahab, R., Kareem, M. A. (2021). Incorporation of metakaolin and nanosilica in blended cement mortar and concrete-A review. Journal of Cleaner Production, 290, 125852. https://doi.org/10.1016/j.jclepro.2021.125852 [Google Scholar] [CrossRef]
8. Homayoonmehr, R., Ramezanianpour, A. A., Mirdarsoltany, M. (2021). Influence of metakaolin on fresh properties, mechanical properties and corrosion resistance of concrete and its sustainability issues: A review. Journal of Building Engineering, 44, 103011. https://doi.org/10.1016/j.jobe.2021.103011 [Google Scholar] [CrossRef]
9. Peeters, B., Dewil, R., Vernimmen, L., van den Bogaert, B., Smets, I. Y. (2013). Addition of polyaluminiumchloride (PACl) to waste activated sludge to mitigate the negative effects of its sticky phase in dewatering-drying operations. Water Research, 47(11), 3600–3609. https://doi.org/10.1016/j.watres.2013.04.012. [Google Scholar] [PubMed] [CrossRef]
10. da Silva, E. M., Morita, D. M., Lima, A. C. M., Teixeira, L. G. (2015). Manufacturing ceramic bricks with polyaluminum chloride (PAC) sludge from a water treatment plant. Water Science and Technology, 71(11), 1638–1645. https://doi.org/10.2166/wst.2015.132. [Google Scholar] [PubMed] [CrossRef]
11. Li, Q., Zhang, J., Gao, J., Huang, Z., Zhou, H. et al. (2022). Preparation of a novel non-burning polyaluminum chloride residue (PACR) compound filler and its phosphate removal mechanisms. Environmental Science and Pollution Research, 29(1), 1532–1545. https://doi.org/10.1007/s11356-021-15724-2. [Google Scholar] [PubMed] [CrossRef]
12. Kim, T., Kang, C., Hong, S., Seo, K. Y. (2019). Investigating the effects of polyaluminum chloride on the properties of ordinary portland cement. Materials, 12(20), 3290. https://doi.org/10.3390/ma12203290. [Google Scholar] [PubMed] [CrossRef]
13. Kim, T., Kim, I. T., Seo, K. Y., Park, H. J. (2019). Strength and pore characteristics of OPC-slag cement paste mixed with polyaluminum chloride. Construction and Building Materials, 223, 616–628. https://doi.org/10.1016/j.conbuildmat.2019.07.009 [Google Scholar] [CrossRef]
14. Kim, T. (2019). The effects of polyaluminum chloride on the mechanical and microstructural properties of alkali-activated slag cement paste. Cement and Concrete Composites, 96, 46–54. https://doi.org/10.1016/j.cemconcomp.2018.11.010 [Google Scholar] [CrossRef]
15. Firdous, R., Stephan, D. (2019). Effect of silica modulus on the geopolymerization activity of natural pozzolans. Construction and Building Materials, 219, 31–43. https://doi.org/10.1016/j.conbuildmat.2019.05.161 [Google Scholar] [CrossRef]
16. Yi, C., Ma, H., Zhu, H., Li, W., Xin, M. et al. (2018). Study on chloride binding capability of coal gangue based cementitious materials. Construction and Building Materials, 167, 649–656. https://doi.org/10.1016/j.conbuildmat.2018.02.071 [Google Scholar] [CrossRef]
17. Burris, L. E., Juenger, M. C. (2020). Effect of calcination on the reactivity of natural clinoptilolite zeolites used as supplementary cementitious materials. Construction and Building Materials, 258, 119988. https://doi.org/10.1016/j.conbuildmat.2020.119988 [Google Scholar] [CrossRef]
18. Pacheco-Torgal, F., Jalali, S. (2010). Reusing ceramic wastes in concrete. Construction and Building Materials, 24(5), 832–838. https://doi.org/10.1016/j.conbuildmat.2009.10.023 [Google Scholar] [CrossRef]
19. Idrees, M., Ekincioglu, O., Sonyal, M. S. (2021). Hydration behavior of calcium aluminate cement mortars with mineral admixtures at different curing temperatures. Construction and Building Materials, 285, 122839. https://doi.org/10.1016/j.conbuildmat.2021.122839 [Google Scholar] [CrossRef]
20. de Carvalho, J. M. F., Defaveri, K., Mendes, J. C., Schmidt, W., Kuehne, H. C. et al. (2021). Influence of particle size-designed recycled mineral admixtures on the properties of cement-based composites. Construction and Building Materials, 272, 121640. https://doi.org/10.1016/j.conbuildmat.2020.121640 [Google Scholar] [CrossRef]
21. Kapeluszna, E., Szudek, W., Wolka, P., Zieliński, A. (2021). Implementation of alternative mineral additives in low-emission sustainable cement composites. Materials, 14(21), 6423. https://doi.org/10.3390/ma14216423. [Google Scholar] [PubMed] [CrossRef]
22. Pu, X. C. (1997). Study on volcanic ash effect of active mineral admixture in cement and concrete by using specific strength index. Concrete and Cement Products, 1997(3), 6–14 (In Chinese). https://doi.org/10.19761/j.1000-4637.1997.03.001 [Google Scholar] [CrossRef]
23. China Building Materials Industry Association (2005). Pozzolanic materials used for cement production. GB/T 2847-2005. In: General administration of quality supervision, inspection and quarantine of the People’s Republic of China. China: Standardization Administration of China. [Google Scholar]
24. Yao, G., Cui, T., Zhang, J., Wang, J., Lyu, X. (2020). Effects of mechanical grinding on pozzolanic activity and hydration properties of quartz. Advanced Powder Technology, 31(11), 4500–4509. https://doi.org/10.1016/j.apt.2020.09.028 [Google Scholar] [CrossRef]
25. Pitarch, A. M., Reig, L., Tomás, A. E., Forcada, G., Soriano, L. et al. (2021). Pozzolanic activity of tiles, bricks and ceramic sanitary-ware in eco-friendly Portland blended cements. Journal of Cleaner Production, 279, 123713. https://doi.org/10.1016/j.jclepro.2020.123713 [Google Scholar] [CrossRef]
26. Liu, S., Wang, L., Li, Q., Song, J. (2020). Hydration properties of Portland cement-copper tailing powder composite binder. Construction and Building Materials, 251, 118882. https://doi.org/10.1016/j.conbuildmat.2020.118882 [Google Scholar] [CrossRef]
Cite This Article
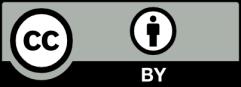
This work is licensed under a Creative Commons Attribution 4.0 International License , which permits unrestricted use, distribution, and reproduction in any medium, provided the original work is properly cited.