Open Access
ARTICLE
Performance Characterization of CR/PU Asphalt for Potential Application in Assembled Fast-Repairing Engineering
1 Hubei Regional Management Headquarters, CCCC Asset Management Co., Ltd., Beijing, 101399, China
2 School of Civil Engineering and Architecture, Wuhan Institute of Technology, Wuhan, 430073, China
3 Hubei Provincial Engineering Research Center for Green Civil Engineering Materials and Structures, Wuhan Institute of Technology, Wuhan, 430073, China
* Corresponding Author: Xiong Xu. Email:
Fluid Dynamics & Materials Processing 2024, 20(5), 1063-1074. https://doi.org/10.32604/fdmp.2023.044000
Received 18 July 2023; Accepted 13 October 2023; Issue published 07 June 2024
Abstract
Conventional repairing methods for asphalt pavement have some inconveniences, such as insufficient strength, and are typically time-consuming. To address these issues, this study proposes a new technological method to design and prepare a high-performance assembled asphalt concrete block for fast repair of the potholes. A series of composite modified asphalt binders with 10% crumb rubber (CR) and different dosages (0%, 1%, 3%, 5%) of polyurethane (PU) are examined to determine the optimized binder. Subsequently, the corresponding asphalt mixtures are prepared for further comparison and assessment of engineering properties, such as moisture-induced damage, high-temperature deformation, and low-temperature cracking characteristics. The test results show that PU can significantly improve the high-temperature performance and hardness of (crumb rubber modified asphalt) CRMA binder; 3% PU contributes allowing the resistance of CRMA mixture to moisture-induced damage at higher levels, particularly under water whole immersion; as 3% PU is added, the high-temperature rutting deformation resistance of the CRMA mixture increases significantly, and the low-temperature anti-cracking properties are also improved slightly. Therefore, the innovatively designed high-quality assembled fast-repairing asphalt concrete block is recommended as an appropriate option for highway maintenance.Keywords
Asphalt pavement is the most commonly applied paving technique around the world. Along with the rapid development of the Chinese economy, the traffic infrastructures, including asphalt pavement, are also continuously developing [1–3]. However, it is inevitable that a variety of road distresses arise, especially potholes, under the repeated loads and environmental aging, during the in-service period [4,5]. Therefore, there is a need to actively consider how to quickly repair potholes and ease traffic for highway maintenance.
With regard to practical engineering, traditional hot/cold mix asphalt repairing technologies are usually used for potholes [6–8]. For hot mix asphalt repair technology, some accessory working equipment is needed to assist the mixing, paving, and rolling processes during implementation [9]. In addition, higher temperature-heating conditioning and a longer closed traffic time are required, which will cause bitumen aging and traffic inconvenience [10]. However, for the cold mix asphalt repairing technology, it is relatively easy to complete similar implementing works, but significant engineering disadvantages such as poor engineering properties and weak bonding with existing asphalt concrete in the original pavement still exist [11–13]. Thus, it is necessary to fully consider how to fast repair pavement potholes in a sustainable manner.
To achieve the high-quality fast repair of asphalt pavements, the prefabricated assembled repairing technology is a possible way for highway maintenance engineering [14]. This technology involves cutting the damaged pavement sections to match the prefabricated assembled repairing block with the corresponding shape and size, and then gluing the gaps between the block and old pavement. Significantly, it brings about some advantages such as a shortened repair time and a controllable performance to the repaired asphalt pavement. To this end, the engineering properties of the prefabricated assembled repairing block should be guaranteed to a satisfactory level.
The prefabricated assembled repairing maintenance technology is a newly emerging approach to achieving the fast repair of pothole distress occurring in asphalt pavements [15]. The conventional repair methods have some inadequacies such as insufficient strength, ready-to-use mixing, and paving, and are time-consuming, leading to their short service life. To adequately address this issue, the prefabrication and application of high-performance assembled fast-repairing asphalt concrete blocks are of great significance. Some publications have proved that crumb rubber (CR), as a cheap asphalt modifier, can effectively improve the overall performance of asphalt mixtures and shows high economic benefits and engineering prospects for asphalt pavement engineering [16–18]. Meanwhile, polyurethane (PU), as a new polymer material, can impart some better performance properties such as high-temperature resistance, aging resistance, and wear resistance, to the asphalt pavement, because it can react with some oxygen-containing molecules, such as hydroxyl-based substances, to form a stable molecular structure [19–20]. Therefore, the appropriate hybrid use of CR and PU can help improve the engineering quality of prefabricated assembled repairing blocks.
This study was aimed at designing a fast-repairing asphalt concrete to quickly repair the pothole distress occurring in highway pavement, instead of simple repair methods. Afterward, the CR/PU composite modified asphalt binders were prepared and characterized by tests including softening point, penetration, and viscosity. Finally, the engineering performance characteristics of the designed asphalt concrete incorporating CR and PU, such as resistance to moisture-induced damage, high-temperature deformation, and low-temperature cracking were evaluated.
The asphalt binder used in this study was a virgin bitumen with a penetration grade of 70, and procured from a local factory. Its main physical properties are presented in Table 1.
To better enhance the prefabricated assembled asphalt concrete, the CR particles, obtained through a process of crushing waste tire rubber at ambient temperature, were selected. The particles were provided by a local supplier. Some physical and chemical properties of the particles are summarized in Table 2.
PU was provided by BASF (China) Co., Ltd., and was used for performance enhancement of the asphalt mixture. It is an isocyanate-based prepolymer and has a high reaction with some oxygen-containing groups, especially -OH. Some basic physical properties are presented in Table 3.
The graded aggregates used in this study were all of the limestone type. Their main physical properties are presented in Table 4.
2.2 Prefabrication of Assembled Fast-Repairing Asphalt Concrete Block
First, the base asphalt was heated to 150°C. Then, the modified asphalt was prepared by adding CR with 10% relative asphalt mass fraction into the molten binder and stirring at 4000 rpm for 30 min. Following this, PU was mixed into the melted CR modified asphalt at contents of 0%, 1%, 3%, and 5% of the relative asphalt mass fraction. The mixture was evenly mixed at a low speed of 3000 rpm for 30 min, and the stirring temperature was maintained at 150°C ± 5°C. Finally, the CR/PU composite modified asphalt binders were prepared. According to the different PU contents, the CR/PU composite modified asphalts were named CR/PU-0, CR/PU-1, CR/PU-3 and CR/PU-5.
To prefabricate the assembled fast-repairing asphalt concrete block, the procedure was as follows: (a) the asphalt mixtures were fully mixed through a mixer at an elevated temperature of 170°C; (b) these mixtures were transferred to a predesigned square mold for shaping; (c) after cooling down, the assembled asphalt concrete block was demolded for further fast repair use.
2.3 Mix Design of Asphalt Concrete
AC-13 type aggregate gradation was selected in this study for determining optimum binder content in asphalt concrete. The selected aggregate gradation curve is shown in Fig. 1. Using the Marshall design method, a binder content of 4.8% was first determined for a series of mixture specimen fabrication, i.e., for Marshall stability, high-temperature rutting deformation, and low-temperature bending deformation. For further performance comparisons, the asphalt mixture with 0% and 3% PU were selected and denoted as CR/PU-0RM and CR/PU-3RM.
Figure 1: AC-13 gradation curve for the target asphalt mixtures
To complete the implementation of the assembled fast-repairing asphalt concrete block, some main processes are summarized and shown in Fig. 2. These processes included the clearing and cutting of the pothole, brushing the adhesive, and finally placing the block to repair the hole. In greater detail, the implementation processes were as follows: first, the pothole was cleared and cut into preset square size and thickness; then, the prepared adhesive was brushed on the surfaces of the cut hole; afterwards, the prefabricated assembled fast-repairing asphalt concrete block was put into the hole; and finally, the gap between the old pavement and the concrete block was fixed with asphalt binder or other adhesives.
Figure 2: Implementation of assembled fast-repairing asphalt concrete block (a) pothole; (b) clear & cut; (c) brush adhesive; and (d) block repairing
According to ASTM D36 and ASTM D113, the high temperature properties and temperature sensitivity of CR/PU composite modified asphalt with different PU contents were evaluated for softening point and penetration. According to ASTM D4402, the viscosities of the CR/PU composite modified asphalt at different temperatures (125°C, 135°C, 145°C, 155°C, and 165°C) were measured by Brookfield rotary viscometer, and the corresponding viscosity-temperature curves were drawn to determine the construction performance of CR/PU composite modified asphalt mixture at different PU contents.
The wheel tracking test (WTT) is a commonly used method to evaluate the high-temperature rutting resistance of asphalt mixture through examining the dynamic stability (DS) of the asphalt mixture, ASTM D8292. For the WTT test, the following standard steps were conducted: a. the prefabricated 300 mm × 300 mm × 50 mm specimen was put into the test chamber and cured at a constant temperature of 60°C for more than 5 h; b. the specimen was rolled for 60 min at a back-and-forth rolling speed of 42 times/min and a wheel load of 0.7 MPa at 60°C. The DS value of the asphalt mixture was calculated according to Eq. (1):
where DS is the dynamic stability of the asphalt mixture, pass/mm; d1 and d2 are respectively the rut depths of the asphalt mixture at 45 and 60 min, mm; and N is the back-and-forth rolling speed of the test wheel, usually 42 times/min.
2.7 Moisture-Induced Damage Test
The moisture-induced damage resistance of the asphalt mixtures was evaluated by immersed Marshall stability and freeze-thaw splitting tests. Two technical parameters, namely, residual Marshall stability (RMS) and freeze-thaw splitting ratio (TSR), were used in this study to verify the influence of different PU dosages on the moisture-induced damage resistance of the CR/PU composite modified asphalt concrete under different conditions.
In accordance with ASTM D6927, the Marshall test specimen was soaked in a water bath at 60°C for 30 min and 48 h, and then tested with a Marshall stability tester at a loading rate of 50 mm/min to obtain the Marshall stability of the test specimen. On this basis, the immersed Marshall residual stability (RMS) of the specimen was obtained, as given by Eq. (2):
where, RMS (%) is the residual Marshall stability after immersion, which indicated that the mixture had a stronger resistance to moisture-induced damage as its value remained higher; MS0 (kN) is the Marshall stability of the specimen after soaking in a water bath at 60°C for 30 min; and MS1 (kN) is the Marshall stability of the specimen after soaking in a water bath at 60°C for 48 h.
According to AASHTO T283, the specimen is required to undergo the freeze-thaw (F-T) process through the following steps: a) put the specimen into a vacuum container, inject distilled water into the container to fully cover the specimen to a depth of no less than 25 mm, reach the absolute pressure of 13–67 kPa (3.8–19.8 inHg) in a short time (5–10 min), and then remove the vacuum in a short time (5–10 min), and take out the specimen; b) cover the specimen with a plastic bag, inject 10 mL of water into it, and seal it; c) place the plastic-sealed specimen in the freezer at −18°C ± 3°C for not less than 16 h and remove from freezer and soak in 60°C ± 1°C water bath for 24 h; and d) soak in a 25°C ± 0.5°C water bath for 2 h. At the same time, the specimens cured under dry conditions were also immersed in a 25°C ± 0.5°C water bath for 2 h. After the described process, the splitting test was carried out. Furthermore, the F-T splitting strength ratio (also called tensile strength ratio, TSR) was calculated following Eq. (3):
where, TSR (%) is the residual tensile strength percentage of the asphalt mixture after the F-T process; ITS0 is the indirect tensile strength of the asphalt mixture without the freeze-thaw cycle; and ITS1 is the indirect tensile strength of the asphalt mixture after one freeze-thaw cycle.
2.8 Low Temperature Trabecular Bending Test
In accordance with JTG E20 T0715-2011, trabecular bending tests were carried out on the two kinds of asphalt mixtures at low temperature. The low temperature properties of CR/PU composite modified asphalt mixtures were evaluated for their maximum flexural strain (εB) and bending stiffness modulus (SB). The test specimens were first prefabricated with dimensions of 250 mm × 35 mm × 30 mm and then placed onto the bearing supports with a 200 mm-span in the universal testing machine (UTM) while maintaining a temperature of −10°C for not less than 4 h. After this, the test was started at a loading speed of 50 mm/min. Finally, the εB and SB values of the specimen were calculated according to Eqs. (4) and (5):
where, εB is the maximum flexural strain of the specimen during failure, με; h is the height across the interrupted interview piece, mm; d is the mid-span deflection when the specimen is damaged, mm; L is the span of the specimen, mm; and RB is the flexural tensile strength of the specimen during failure, MPa.
3.1 Physical Properties of Composite Modified Asphalt Binder
Fig. 3 shows the basic physical properties of the CR/PU composite modified asphalt binder. As the PU content decreased, the penetration of the modified asphalt binder gradually decreased, while the softening point correspondingly increased. When the PU content was added at 1%, 3%, and 5%, the penetration value decreased from 40 to 38 dmm, 37 dmm, and 34 dmm, respectively. Meanwhile, the softening point increased from 51.8°C to 56.5°C, 57.0°C, and 58.7°C. The results obtained indicated that the incorporation of PU was helpful in improving the high temperature performance and enhancing the hardness of the CRMA binder.
Figure 3: Physical properties of CR/PU composite modified asphalt binders: (a) softening point and penetration; and (b) viscosity
As shown in Fig. 3b, the viscosity of the CRMA binder increased to some extent with the increasing PU content at 125°C–165°C, while the viscosities were significantly lower than 3 Pa·s (135°C) required by the specification. Moreover, it was also understood that the viscosity-temperature changing trends of all binders were similar, indicating that the viscosity-temperature sensitivities of the CRMA binder with different PU contents were close to each other. The results indicated that the addition of PU somewhat increased the viscosity of the CRMA binder, but did not obviously influence the ultimate workability and temperature sensitivity as its content changed. This is because some of the PU molecules will react with the water molecules in the air environment and become self-solidified in the asphalt binder, leading to a restriction of the movement of asphalt molecules, and at higher temperatures, most solidified molecules will regain movement.
Overall, the results showed that the PU performed adequately enough to improve the physical properties of the CRMA binder, especially contributing to improving the high-temperature characteristic of the binder. For further characterization of the mixture, the CR/PU-0RM and CR/PU-3RM mixtures were optimally selected.
3.2 Engineering Performance of Composite Modified Asphalt Mixture
3.2.1 Moisture-Induced Damage Resistance
Figs. 4 and 5 display the test results of the specimens after F-T and immersion Marshall tests, respectively. As shown in Fig. 3, the TSR value for CR/PU-0RM reached 92.7% and slightly changed to 89.4% for CR/PU-3RM, both of which were still far higher than the standard requirement of ≥80%. As shown in Fig. 4, the RMS value for CR/PU-0RM reached 92.2% and improved to 95.7% for CR/PU-3RM, which were higher than the specification requirements of ≥85%. These results indicated that the moisture-induced damage resistance of the CRMA mixture stayed at a higher level with the incorporation of 3% PU, particularly under full immersion in water. This is because PU can fully react with the water molecules, to impart the binder with a strong bonding ability for aggregates under the 60°C water-bath immersion and slightly decrease the flexural property at low temperature due to the resultant hardening effect. Overall, it is appropriate to use this design to fabricate the assembled fast-repairing asphalt concrete with excellent moisture-induced damage resistance.
Figure 4: Effect of 3% PU on the TSR value of CRMA mixture
Figure 5: Effect of 3% PU on the RMS value of CRMA mixture
3.2.2 High-Temperature Deformation Resistance
The high-temperature rut depth and DS of the asphalt mixtures are shown in Figs. 5 and 6. As shown in Fig. 6, it was clear that the rut depth of CR/PU-3RM was lower than that of CR/PU-0RM during the test period, and at the end stage between 45 and 60 min, the rut depth of CR/PU-3RM was markedly lower. Therefore, the results showed that PU can help reduce the deepening of ruts in the CRMA mixture at high temperature, contributing to improving the resistance to high-temperature deformation. As shown in Fig. 7, the DS value for CR/PU-3RM reached 4809 pass/mm and was 3620 pass/mm for CR/PU-0RM, showing that the addition of PU caused a 32.8% increment in the DS value of the CRMA mixture. The results demonstrated that 3% PU is capable of greatly improving the resistance to high-temperature deformation of the CRMA mixture. This is dependent upon the PU being able to gradually enhance the binder strength and anti-deformation ability at high temperature over time due to the curing reaction caused by water in the air. In summary, the designed mixture can be used to provide better resistance to high-temperature loads when employed in assembled fast-repairing maintenance engineering.
Figure 6: Rut depth development of CRMA mixture containing 0% and 3% PU
Figure 7: DS results of CRMA mixture containing 0% and 3% PU
3.2.3 Low-Temperature Property
Fig. 8 shows the results of low-temperature trabecular bending test of the asphalt mixtures. It is significant that as 3% PU was added, the εB value of CR/PU-0RM increased from 2655.05 to 2963.29 με, while the SB value correspondingly decreased from 3843.24 to 3443.47 MPa. The results indicated that 3% PU can give a slight flexural improvement to the asphalt mixture at low temperature and only limitedly decreased the bending deformation resistance. Thus, it is obvious that CR/PU-3RM can provide a better low-temperature property to meet the application requirement when used in assembled fast-repairing maintenance engineering.
Figure 8: Low-temperature bending test results of CRMA mixture containing 0% and 3% PU
This study proposed to design a prefabricated assembled asphalt concrete block to fast repair the pothole distress occurring in highway pavement, as an alternative to simpler repair methods. To meet this objective, the engineering performance of the designed asphalt concrete incorporating crumb rubber and polyurethane, on properties such as moisture-induced damage, high-temperature deformation, and low-temperature cracking characteristic, were evaluated and verified. Some main conclusions were obtained as follows:
1) Physical properties results indicated that as the polyurethane content increased, the softening point and the penetration of the CRMA binder increased and then decreased. The viscosity increased a little bit within the whole temperature range, implying that PU is capable of improving the high-temperature property with less negative influence on the workability of the CRMA binder.
2) According to the moisture-induced damage results, incorporation of 3% PU allowed the RMS and TSR values of the CRMA mixture to remain at higher levels of 95.7% and 89.4%, respectively, due to the chemical interaction between PU and water.
3) Wheel tracking test results showed that incorporation of 3% PU increased the DS value of the CRMA mixture from 3620 to 4809 pass/mm indicating a better resistance to high-temperature deformation when applied in practice.
4) Low-temperature properties results indicated that incorporation of 3% PU helped improve the flexural behavior of the CRMA mixture and provides a slightly decreased stiffness, which can enhance the low-temperature anti-cracking performance within the allowable loading range.
Overall, this study provides a new fast-repair method and the use of prefabricated assembled asphalt concrete blocks to fill potholes for highway pavement maintenance. However, there are still some issues that need to be addressed in the future, such as aging characteristics of the block, bonding strength improvement between old pavement and the block, and simpler implementation methods. After further investigations, the prefabricated assembled asphalt concrete block may be recommended and promoted in highway pavement construction, especially for expressway construction and service examinations.
Acknowledgement: The authors thank to the financial supports from the company for this work.
Funding Statement: This work was supported by the Scientific Technology R&D Project of CCCC Asset Management Co., Ltd. (RP2022015294 & RP2022015296).
Author Contributions: The authors confirm contribution to the paper as follows: Hong Pang: Conceptualization, Methodology, Resources, Formal analysis, Writing-review & editing; Ao Lu & Ming Xiong: Data curation, Formal analysis, Investigation, Validation, Writing-original draft; Chen Chen: Conceptualization, Methodology, Formal analysis, Writing-review & editing; Xian Cao & Xiong Xu: Test result analysis, Manuscript revision & editing; Jing Wang: Investigation, Testing and testing assistance, Funding acquisition. All authors reviewed the results and approved the final version of the manuscript.
Availability of Data and Materials: The data that has been used is confidential.
Conflicts of Interest: The authors declare that they have no conflicts of interest to report regarding the present study.
References
1. Yousefi, A. A., Haghshenas, H. F., Underwood, B. S., Harvey, J., Blankenship, P. (2023). Performance of warm asphalt mixtures containing reclaimed asphalt pavement, an anti-stripping agent, and recycling agents: A study using a balanced mix design approach. Construction and Building Materials, 363, 129633. https://doi.org/10.1016/j.conbuildmat.2022.129633 [Google Scholar] [CrossRef]
2. Xu, X., Sreeram, A., Leng, Z., Yu, J. Y., Li, R. et al. (2022). Challenges and opportunities in the high-quality rejuvenation of unmodified and SBS modified asphalt mixtures: State of the art. Journal of Cleaner Production, 378, 134634. https://doi.org/10.1016/j.jclepro.2022.134634 [Google Scholar] [CrossRef]
3. Anupam, B. R., Sahoo, U. C., Chandrappa, A. K. (2022). A methodological review on self-healing asphalt pavements. Construction and Building Materials, 321, 126395. https://doi.org/10.1016/j.conbuildmat.2022.126395 [Google Scholar] [CrossRef]
4. Koch, C., Jog, G. M., Brilakis, I. (2013). Automated pothole distress assessment using asphalt pavement video data. Journal of Computing in Civil Engineering, 27(4), 370–378. https://doi.org/10.1061/(ASCE)CP.1943-5487.0000232 [Google Scholar] [CrossRef]
5. Ouma, Y. O., Hahn, M. (2017). Pothole detection on asphalt pavements from 2D-colour pothole images using fuzzy c-means clustering and morphological reconstruction. Automation in Construction, 83, 196–211. https://doi.org/10.1016/j.autcon.2017.08.017 [Google Scholar] [CrossRef]
6. Wang, T., Dra, Y. A. S. S., Cai, X. P., Cheng, Z. Q., Zhang, D. et al. (2022). Advanced cold patching materials (CPMs) for asphalt pavement pothole rehabilitation: State of the art. Journal of Cleaner Production, 366, 133001. https://doi.org/10.1016/j.jclepro.2022.133001 [Google Scholar] [CrossRef]
7. Byzyka, J., Rahman, M., Chamberlain, D. A. (2020). Thermal analysis of hot mix asphalt pothole repair by finite-element method. Journal of Transportation Engineering, Part B: Pavements, 146(3), 04020029. [Google Scholar]
8. Byzyka, J., Rahman, M., Chamberlain, D. A. (2020). An improved interface temperature distribution in shallow hot mix asphalt patch repair using dynamic heating. International Journal of Pavement Engineering, 21(13), 1617–1625. https://doi.org/10.1080/10298436.2018.1559315 [Google Scholar] [CrossRef]
9. Hawesah, H. A., Sadique, M., Harris, C., Nageim, H. A., Stopp, K. et al. (2022). Polymer modified asphalt binder-an approach for enhancing temperature sensitivity for emergency pavement repair. International Journal of Pavement Engineering, 23(13), 4760–4774. https://doi.org/10.1080/10298436.2021.1975704 [Google Scholar] [CrossRef]
10. Li, J. S., Liu, J. F., Zhang, W. B., Liu, G. L., Dai, L. C. (2019). Investigation of thermal asphalt mastic and mixture to repair potholes. Construction and Building Materials, 201, 286–294. https://doi.org/10.1016/j.conbuildmat.2018.12.153 [Google Scholar] [CrossRef]
11. Hafezzadeh, R., Autelitano, F., Giuliani, F. (2021). Asphalt-based cold patches for repairing road potholes–an overview. Construction and Building Materials, 306, 124870. https://doi.org/10.1016/j.conbuildmat.2021.124870 [Google Scholar] [CrossRef]
12. Jain, S., Singh, B. (2021). Cold mix asphalt: An overview. Journal of Cleaner Production, 280, 124378. https://doi.org/10.1016/j.jclepro.2020.124378 [Google Scholar] [CrossRef]
13. Biswas, S., Hashemian, L., Hasanuzzaman, M., Bayat, A. (2016). A study on pothole repair in Canada through questionnaire survey and laboratory evaluation of patching materials. Canadian Journal of Civil Engineering, 43(5), 443–450. https://doi.org/10.1139/cjce-2015-0553 [Google Scholar] [CrossRef]
14. Fang, M. J., Zhou, R., Ke, W. H., Tian, B., Zhang, Y. B. et al. (2022). Precast system and assembly connection of cement concrete slabs for road pavement: A review. Journal of Traffic and Transportation Engineering (English Edition), 9(2), 208–222. https://doi.org/10.1016/j.jtte.2021.10.003 [Google Scholar] [CrossRef]
15. Ren, J. J., Wang, J., Li, X., Wei, K., Li, H. L. et al. (2020). Influence of cement asphalt mortar debonding on the damage distribution and mechanical responses of CRTS I prefabricated slab. Construction and Building Materials, 230, 116995. https://doi.org/10.1016/j.conbuildmat.2019.116995 [Google Scholar] [CrossRef]
16. Xu, X., Leng, Z., Lan, J. T., Wang, W., Yu, J. M. et al. (2021). Sustainable practice in pavement engineering through value-added collective recycling of waste plastic and waste tyre rubber. Engineering, 7(6), 857–867. https://doi.org/10.1016/j.eng.2020.08.020 [Google Scholar] [CrossRef]
17. Rodriguez-Fernandez, I., Baheri, F. T., Cavalli, M. C., Poulikakos, L. D., Bueno, M. (2020). Microstructure analysis and mechanical performance of crumb rubber modified asphalt concrete using the dry process. Construction and Building Materials, 259, 119662. https://doi.org/10.1016/j.conbuildmat.2020.119662 [Google Scholar] [CrossRef]
18. Ren, S. S., Liu, X. Y., Xu, J., Lin, P. (2021). Investigating the role of swelling-degradation degree of crumb rubber on CR/SBS modified porous asphalt binder and mixture. Construction and Building Materials, 300, 124048. https://doi.org/10.1016/j.conbuildmat.2021.124048 [Google Scholar] [CrossRef]
19. Ren, S. S., Liu, X. Y., Lin, P., Wang, H. P., Fan, W. Y. et al. (2021). The continuous swelling-degradation behaviors and chemo-rheological properties of waste crumb rubber modified bitumen considering the effect of rubber size. Construction and Building Materials, 307, 124966. https://doi.org/10.1016/j.conbuildmat.2021.124966 [Google Scholar] [CrossRef]
20. Shirzad, S., Hassan, M., Mohammad, L. N. (2020). Rheological and mechanical evaluation of polyurethane prepolymer-modified asphalt mixture with self-healing abilities. Journal of Materials in Civil Engineering, 32(8), 04020231. https://doi.org/10.1061/(ASCE)MT.1943-5533.0003307 [Google Scholar] [CrossRef]
Cite This Article
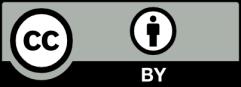
This work is licensed under a Creative Commons Attribution 4.0 International License , which permits unrestricted use, distribution, and reproduction in any medium, provided the original work is properly cited.