Open Access
ARTICLE
Study on Optimal Water Control Methods for Horizontal Wells in Bottom Water Clastic Rock Reservoirs
1 Sinopec Northwest Oilfield Branch, Northwest Oilfield Branch of China Petroleum and Chemical Corporation, Urumqi, 830011, China
2 School of Petroleum and Natural Gas Engineering, Southwest Petroleum University, Chengdu, 610500, China
* Corresponding Author: Dongling Qiu. Email:
(This article belongs to the Special Issue: Fluid and Thermal Dynamics in the Development of Unconventional Resources II)
Fluid Dynamics & Materials Processing 2024, 20(10), 2377-2392. https://doi.org/10.32604/fdmp.2024.051418
Received 05 March 2024; Accepted 20 June 2024; Issue published 23 September 2024
Abstract
The segmented water control technology for bottom water reservoirs can effectively delay the entry of bottom water and adjust the production profile. To clarify the impact of different methods on horizontal well production with different reservoir conditions and to provide theoretical support for the scientific selection of methods for bottom water reservoirs, a numerical simulation method is presented in this study, which is able to deal with wellbore reservoir coupling under screen tube, perforation, and ICD (Inflow Control Device) completion. Assuming the geological characteristics of the bottom-water conglomerate reservoir in the Triassic Formation of the Tahe Block 9 as a test case, the three aforementioned completion methods are tested to predict the transient production characteristics. The impact of completion parameters, reservoir permeability, bottom-water energy, and individual well control on the time to encounter water in horizontal wells (during a water-free production period) is discussed. A boundary chart for the selection of completion methods is introduced accordingly. The results show that the optimized ICD completion development effect for heterogeneous reservoirs is the best, followed by optimized perforation completion. Permeability is the main factor affecting the performances of completion methods, while bottom water energy and single well controlled reserves have a scarce impact. The average permeability of the reservoir is less than 500 mD, and ICD has the best water control effect. If the permeability is greater than 500 mD, the water control effect of perforation completion becomes a better option.Keywords
Nomenclature
Symbol | Description Units |
hperf | Spacing between perforations m |
lperf | Perforation depth m |
Kh | Formation permeability mD |
Kdp | Permeability of perforation compacted zone mD |
Kd | Permeability of contaminated zone mD |
rdp | Radius of perforation tunnel mm |
rp | Perforation penetration radius mm |
Kgrav | Permeability of double-layer screen filling mD |
Kg | Effective permeability of gas reservoir mD |
rs1 | Outer diameter of outer screen of double-layer screen tube mm |
rs2 | Inner diameter of outer screen of double-layer screen tube mm |
The Tahe Oilfield comprises multiple layers, including Paleogene, Cretaceous, Triassic, and Carboniferous formations. These layers are vertically distributed with clastic rock reservoirs containing bottom water. The thickness of the oil layer varies significantly between different formations and ranges from 1.5 to 20 m, with substantial differences in porosity and permeability. The porosity ranges from 6% to 30%, whereas the permeability ranges from 0.1 to 2600 mD. Horizontal wells have the advantages of reducing differences in production pressure and delaying the rise of bottom water, and they are widely used in the development of reservoirs with bottom water [1,2]. However, certain issues have become more prominent as horizontal well technology has been applied and promoted in different types of reservoirs. These are primarily manifested as nonuniform production of liquid along the horizontal section that leads to premature breakthrough of bottom water [3,4]. Consequently, there is a sharp decline in oil production, which severely affects the development effect and the benefits of reservoirs with bottom water. Some investigators have used physical experiments, analysis, numerical simulations, and other methods to study the formation morphology and water breakthrough behavior of horizontal wells in reservoirs with bottom water [5–8]. Their research focused on the effects of reservoir thickness, crude oil viscosity, reservoir heterogeneity, and differences in production pressure on the rules governing water breakthrough in horizontal wells [9,10]. Okon et al. [11] conducted a correlation evaluation and prediction of water coning to identify the primary influencing factors of coning in horizontal wells. Their objective was to optimize the critical oil production rate and breakthrough time in reservoirs with bottom water. On the basis of physical simulation experiments, Li et al. [12] proposed a new method for calculating the characteristic shape of water ridges in heavy oil reservoirs in the presence of bottom water, taking into consideration the effects of horizontal production wells and natural waterflooding. Kuo et al. [13] conducted a numerical simulation to analyze the sensitivity of water coning. They also made predictions of the critical production rate, breakthrough time, and water content and provided judgments for actual production operations.
However, the influence of different completion methods has not been considered. At present, the main approach for solving the problem of premature water breakthrough and achieving a balanced production profile in horizontal wells involves completion processes that control the inflow profile of the wellbore. These processes employ stepped screen tubes, central tubes, variable-density perforations, and flow-regulating screen tubes [14,15]. Some researchers have also studied the development of reservoirs with bottom water and conducted flow modeling based on different spatial parameters, such as the reservoir, gravel pack, inflow control device (ICD) completion interval, and horizontal well. Using integrated ICD completion in horizontal wells, the oil production, water yield, and gravel pack water cut under different conditions can be predicted [16]. Jin et al. [17] developed a new device, known as the downhole water loop, for effectively controlling water coning. This device has been utilized in combination with production operations, and its feasibility has been confirmed, which demonstrated good applicability. Yang et al. [18] established a three-dimensional dynamic simulation model of two-phase flow in horizontal wells completed using a phase selection controller (PSC) by coupling the reservoir heterogeneity, anisotropy, drilling damage, and other factors. They also proposed a design method for optimizing the completion of horizontal wells with a PSC. Elverhøy et al. [19] optimized and improved the Autonomous Inflow Control Valve (AICV) to prevent premature water breakthrough in horizontal wells and implemented AICV technology to enhance oil production and the recovery factor. Zhang et al. [20] addressed reservoir heterogeneity and differences in lithology in horizontal wells by employing adjustable-flow production control valves to regulate segmented production in horizontal wells. This represented an effective method for delaying water breakthrough and achieving satisfactory results. Luo et al. [21] used simulation methods to conduct calculations in instances of ICD completion and analyses of parameter sensitivity. They also proposed design methods for optimizing ICD completion under different arrangements. The results indicate that this design approach not only leads to a more uniform liquid production profile but also increases the oil recovery index in horizontal wells.
However, appropriate completion methods for water control and process parameters for different reservoirs with bottom water have not been systematically demonstrated and comprehensively evaluated. In the Tahe area, in the sandstone reservoir with bottom water, three methods are mainly adopted: screen tube, perforation, and ICD completion. Field production operations show significant differences in the water control ability of these completion methods, which affects the final development of oil wells. Therefore, it is necessary to study the effects of different completion methods in horizontal wells in sandstone reservoirs with bottom water under various conditions to develop recommended completion practices that are suitable for clastic reservoirs. On the basis of the geological characteristics of typical clastic rock reservoirs with bottom water in the Tahe area, in this study a numerical simulation mechanistic model of wellbore-reservoir coupling with perforation, screen tube, and flow-regulating screen tube completion was established. This model simulates the effects of different geological parameters on the development of horizontal wells in reservoirs with bottom water, compares and analyzes the adaptability of completion methods for water control, and provides technical support for optimizing and efficiently developing completion methods for reservoirs with bottom water.
2 Overview of Tahe Clastic Rock Reservoir
The Triassic lower oil reservoir in Block 9 of the Tahe Oilfield in the Tarim Basin is characterized by a low-amplitude faulted anticline trap. The southern flank of the reservoir is intersected by a northeast-trending normal fault, which divides the structure into two asymmetrical blocks. This reservoir is considered to be a significant reservoir with bottom water, with a bottom water thickness of approximately 120 m and extensive edge water, which both contain abundant natural energy. The Triassic lower oil-bearing group in Block 9 represents typical braided river deposition and consists of light-gray conglomeratic coarse sandstone, conglomeratic medium-coarse sandstone, medium sandstone, and fine-medium sandstone lithologies. In terms of physical properties, the reservoir mainly exhibits medium porosity (ranging between 17.7% and 23.5%, with a median value of 21.3%) and medium permeability (ranging from 16.4 × 10−3 μm2 to 1893.4 × 10−3 μm2, with a median value of 375 × 10−3 μm2). The reservoir also exhibits significant directional permeability, with a ratio of vertical permeability to horizontal permeability of 1:1.34. The density of the formation crude oil is 0.7576 g/cm3, with a viscosity of 2.78 mPa·s and a saturation pressure of 33.17 MPa. The original gas/oil ratio is 125 m3/m3, and the volume coefficient of crude oil is 1.3404 m3/m3. The difference between the reservoir pressure and the bubble point pressure is 16.59 MPa, which indicates that the reservoir is in an unsaturated state. The original formation pressure is 49.76 MPa, and the reservoir temperature is 110.53°C. Overall, these parameters suggest that the reservoir is currently in an unsaturated state, according to the difference between the reservoir pressure and the bubble point pressure.
3 Establishment of a Numerical Simulation Model for Horizontal Wells in Sandstone Reservoirs with Bottom Water Considering Completion Methods
3.1 Establishment of a Numerical Simulation Mechanistic Model for Horizontal Wells in Sandstone Reservoirs with Bottom Water
A conceptual mechanistic model of a sandstone reservoir with strong bottom water in Tahe Block 9 was established on the basis of geological, logging, and fluid parameters (Table 1). An analysis of the water breakthrough characteristics and production dynamics of horizontal wells was conducted using Petrel RE commercial numerical simulation software. The grid size in the 3D conceptual model was 50 m × 20 m in the I direction and 30 m × 20 m in the J direction. As for the K direction, the oil layer grid size was 20 m × 1 m, whereas the water layer grid size was 1 m × 5 m. Below the water layer, a Fetkovich aquifer was established with a water body multiple of 80 times the reservoir volume and a water encroachment factor of 500.
From the results of logging interpretation (Fig. 1), it is evident that the porosity and permeability exhibit different changes with variations in formation depth. On the basis of the results of logging interpretation for well TK907H in the target area, we established a saturation model of the reservoir with bottom water and a conceptual geological model of the bottom water heterogeneity in five segments with different permeability (Fig. 2). Furthermore, these models were simplified for clarity and ease of understanding. The models were then used to simulate and analyze the effect of encountering heterogeneity while drilling horizontal wells on completion methods using the curve of the relative permeability of the target block (Fig. 3). In order to enhance the representativeness and reliability of the mechanistic model, production history matching for well TK907H was initially conducted (Fig. 4). It can be observed from Fig. 4 that data points for actual production are located on both sides of the fitted curve of the water content, which indicates accurate fitting.
Figure 1: Logging interpretation results
Figure 2: Conceptual model of Tahe Block 9
Figure 3: Curve of relative permeability (Kro = relative permeability of oil; Krw = relative permeability of water)
Figure 4: Fitted historical data of water cut for well TK907H
3.2 Numerical Simulation of Coupled Well Completion Methods
Using the Petrel RE software platform, a three-dimensional conceptual model of a heterogeneous reservoir was established. Firstly, on the basis of actual drilling and completion engineering parameters, parameters such as the horizontal section trajectory and wellbore diameter are set. Different completion methods are employed according to the reservoir heterogeneity, which include setting the completion time, segmentation, and sizes. This enables the prediction of transient production for a coupled reservoir and horizontal wellbore with segmented completion. Essentially, the flow restriction in horizontal well completion methods controls the liquid production profile by introducing an additional pressure drop as fluid flows through the channels into the wellbore. Screen and perforation completion methods mainly limit the flow rate in each segment according to different skin factors. ICD completion methods are analyzed directly on the basis of specific ICD completion parameters. The formulas for calculating the skin factors for perforation and screen completion methods [22,23] are shown below:
According to the actual field conditions, in the Tahe conglomerate reservoir 102 and 89 mm perforating guns are primarily utilized for perforation. The main perforation pattern is a six-sector spiral with phase angles ranging from 30° to 330°. The perforation density ranges from 8 holes/m to 16 holes/m. In accordance with the characteristics and parameter ranges of the perforation process, the skin factor for perforation completion is calculated using Eq. (1) and falls within the range of −0.48 to 4.26. For the target zone in screen completion in horizontal wells, the slot width is 0.4 to 0.45 mm, the length of each slot is 60 mm, and the spacing between slot units is 40 mm. By applying Eq. (2), the skin factor for screen completion (Ss) can be calculated and ranges from 1.1 to 1.28.
4 Prediction of Production and Effects in Horizontal Wells with Different Completion Methods
On the basis of the heterogeneous mechanistic model of well TK907H, the effect of reservoir permeability heterogeneity on horizontal well completion methods, including screen, perforation, and ICD completion, was simulated and analyzed. A schematic diagram of these three completion methods is shown in Fig. 5. Five different simulation schemes based on these three well completion methods were set up to account for differences in reservoir properties, and the results are presented in Table 2. The range of variations in the additional skin factor for completion using the screen tube was small, and an additional skin factor of 1 was set. The parameters of perforation–ICD heterogeneous completion were adjusted and optimized according to the range of the additional skin factor and the size of the ICD aperture to make the horizontal well production profile as balanced as possible.
Figure 5: Schematic illustration of different well completion settings in numerical simulation
Production forecasts for the three different completion methods (five scenarios) are depicted in Figs. 6 and 7. There were significant differences in production characteristics among the various scenarios; however, overall, the water breakthrough pattern and predicted water cut at the end of the forecast period in the sandstone reservoir with strong bottom water and horizontal wells were relatively similar. It can be concluded from the schemes illustrated in Fig. 7 that under the same bottom water conditions, optimized ICD completion can effectively regulate the ridging of bottom water, delay water breakthrough, and prevent premature water breakthrough in high-permeability zones and cases of oil well flooding. In the other four schemes, the heterogeneity of the reservoir mainly shows that formation water invaded rapidly along the high-permeability zone and exhibited an uneven advance.
Figure 6: Results of production forecasts for different well completion methods
Figure 7: Water saturation profile for different completion methods
A comparison of the water breakthrough time and cumulative production during the water-free period for the different completion methods in horizontal wells is illustrated in Figs. 8 and 9. Following optimization, it is evident that ICD completion yielded the most favorable effect on development, followed by perforation completion, whereas screen tube completion exhibited the weakest water control effects. Specifically, segmented ICD completion exhibits superior water control effects and regulatory capabilities in sandstone reservoirs where significant bottom water is present; however, targeted optimization of parameters based on heterogeneity of reservoir properties is essential.
Figure 8: Comparison of water breakthrough time for different well completion methods
Figure 9: Comparison of oil recovery during water-free period for different well completion methods
5 Boundary Chart for Selecting Completion Methods According to Different Geological Parameters
Research and field production indicate that the excellent properties and abundant bottom water energy of sandstone reservoirs with strong bottom water are the main factors influencing water breakthrough in horizontal wells. According to the heterogeneous model, a diagram was created to illustrate the boundaries between the geological parameters for which different completion methods are suitable.
On the basis of the actual heterogeneous geological model of the target area, seven sets of heterogeneous models with average permeabilities of 10, 50, 100, 300, 500, 700, and 900 mD, respectively, were constructed using a proportional scaling approach. The completion parameters should be adjusted according to the permeability heterogeneity to help the advance of water ridges to be as uniform as possible. Furthermore, it is important to predict the effects of various completion methods on horizontal well production from sandstone reservoirs with bottom water under conditions of different permeabilities.
Using the cumulative production during the initial water-free period with barefoot completion as a baseline, simulations were conducted to compare the incremental oil production ratio for cumulative production during the water-free period between the three completion methods (screen tube, perforation, and ICD) and barefoot completion at different permeabilities. The curve of the relationship with the average permeability is shown in Fig. 10. The average permeability of the reservoir was less than 500 mD. Both ICD completion and perforation completion had certain water control effects, but ICD completion exhibited superior water control effects. However, when the permeability exceeded 500 mD, the water control effects of both ICD completion and perforation completion deteriorated. In this case, the water control effect of ICD completion decreased more as the permeability increased. Therefore, perforation completion is recommended because of its better water control effect. Overall, owing to its weak ability in terms of flow regulation and water control, the water breakthrough time and cumulative production during the water-free period in the case of screen tube completion were similar to those for barefoot completion. In addition, it has been observed that screen tube completion has a minimal effect on increasing oil production and controlling water in comparison with other methods.
Figure 10: Relationship between permeability and incremental oil production ratio during water-free period for different well completion methods
Mechanistic models with water body multiples of 10, 50, 100, 300, 500, and 1000 were constructed to predict the effects of completion methods on production from sandstone reservoirs with bottom water and water bodies of varying sizes (Fig. 11). The geological conditions of the oil reservoir were fixed, and the size of the water body had a significant effect on the water breakthrough time and water production pattern but a relatively small effect on the optimal completion method. From Fig. 11, it can be seen that, regardless of the water body multiple, the ICD well completion method was hardly affected. When the water body multiple was less than 100, the incremental oil production ratio during the water-free period for perforation completion and screen tube completion increased; when the water body multiple reached 100, this ratio stabilized.
Figure 11: Relationship between water body multiple and incremental oil production ratio during water-free period for different well completion methods
On the basis of the conceptual model of reservoir heterogeneity, ten sets of mechanistic models with reserve multiples of 0.6–1.5 were constructed to predict the effect of the reserve size on production from sandstone reservoirs with bottom water. As depicted in Fig. 12, larger reserves led to a delay in water breakthrough and an increase in cumulative oil production during the water-free period from wells employing the three completion methods. Overall, the reserve size did not significantly affect the choice of completion method.
Figure 12: Relationship between controlled reserves in reservoir and incremental oil production ratio during water-free period for different well completion methods
Sensitivity analysis of reservoir permeability and oil viscosity yielded results as shown in Figs. 13 and 14, respectively. For the analysis of reservoir permeability, maintaining the ratio of vertical permeability to horizontal permeability at 0.1, horizontal permeabilities of 100, 200, 300, and 400 mD were considered while keeping other parameters constant to investigate the effect of the simulated permeability on oil well production (Fig. 13). Higher permeability enhanced the deliverability of the reservoir, which led to longer stable production periods for horizontal wells (ICD completion) and correspondingly later breakthrough times. Regarding oil viscosity, with other parameters held constant and setting the viscosity of reservoir crude oil at 1, 3, 5, and 7 mPa·s, the analysis examined the influence of oil viscosity on horizontal well production (Fig. 14). An increase in oil viscosity resulted in a reduction in fluidity, which led to earlier breakthrough times and shorter stable production periods in oil wells.
Figure 13: Effect of permeability on oil well production (ICD completion) (Kh = horizontal permeability)
Figure 14: Effect of oil viscosity on oil well production (ICD completion)
(1) A computational simulation method has been developed to integrate screen tube, perforation, and ICD completion methods in order to achieve predictions of transient production dynamics for horizontal wells across various completion methods.
(2) For heterogeneous reservoirs, ICD completion has been found to achieve the most effective development after optimization, followed by perforation completion. Conversely, screen tube completion exhibited the poorest water control performance. Specifically, in highly heterogeneous sandstone reservoirs with strong bottom water, segmented ICD completion in horizontal wells has been shown to possess the strongest capabilities in terms of water control and adjustment. However, targeted optimization of parameters based on heterogeneity of reservoir properties is necessary.
(3) The permeability of conglomerate reservoirs with bottom water is the primary factor influencing the selection of optimal completion methods, whereas the energy of the bottom water and reserves controlled by individual wells have minimal effects on the choice of completion methods.
(4) For reservoirs with a permeability of less than 500 mD, ICD completion is most effective in controlling water production. However, when the permeability exceeds 500 mD, both ICD completion and perforation completion are less effective, with perforation completion exhibiting superior performance. Screen tube completion has the weakest capabilities in terms of flow regulation and water control among the three methods.
(5) On the basis of discussions of completion methods according to various geological parameters, a decision boundary chart has been established for selecting completion methods in the Tahe fractured sandstone reservoir with bottom water. This considers different permeabilities, bottom water energies, and controlled reserves.
Acknowledgement: The authors would like to thank the reviewers and editors for their useful suggestions for improving the quality of our manuscript.
Funding Statement: The authors received no specific funding for this study.
Author Contributions: The authors confirm contribution to the paper as follows: study conception and design: Xianghua Liu and Hai Song; data collection: Lu Zhao and Neng Yang; analysis and interpretation of results: Neng Yang, Dongling Qiu, and Yan Zheng; draft manuscript preparation: Dongling Qiu and Xianghua Liu. All authors reviewed the results and approved the final version of the manuscript.
Availability of Data and Materials: Data will be made available on request.
Conflicts of Interest: The authors declare that they have no conflicts of interest to report regarding the present study.
References
1. Huang S, Zeng B, Zhao F, Cheng L, Du B. Water breakthrough shape description of horizontal wells in bottom-water reservoir. Math Probl Eng. 2014;2014:1–9. [Google Scholar]
2. Yuan H, Li W, Yuan Y, Luo J, Yan W. Productivity evaluation of horizontal well in heterogeneous reservoir with composite water aquifer. J Petrol Explor Prod. 2021;11:1363–73. doi:10.1007/s13202-021-01104-6. [Google Scholar] [CrossRef]
3. Li YT, Yao YD, Peng T, Sang D, Wang XC. Systematic evaluation on horizontal well performance in bottom water reservoir. Appl Mech Mater. 2013;411–14:3129–33. [Google Scholar]
4. Zhang JM, Wu XD, Zhang Z, Zhang HH, Zhang J, Wang YN. Study on fluid solid coupling of horizontal wells in bottom water sandstone reservoir. Adv Mater Res. 2014;827:239–43. [Google Scholar]
5. Recham R, Osisanya SO, Touami M. Effects of water coning on the performance of vertical and horizontal wells-a reservoir simulation study of Hassi R’mel field, Algeria. In: Proceedings of the SPE/CIM International Conference on Horizontal Well Technology; 2000; Calgary, Alberta, Canada. SPE-65506-MS. [Google Scholar]
6. Giger FM. Analytic 2-D models of water cresting before breakthrough for horizontal wells. In: Proceedings of the SPE Annual Technical Conference and Exhibition; 1986; New Orleans, LA, USA. CONF-861080. [Google Scholar]
7. Dou H, Guan C, Lian S. The experimental studies of physical simulation of bottom water reservoirs with barrier and permeable interbed on horizontal well. In: Proceedings of the SPE Western Regional Meeting; 1999; Anchorage, AK, USA. SPE-55995-MS. [Google Scholar]
8. Luo W, Li HT, Wang YQ, Wang JC. A new semi-analytical model for predicting the performance of horizontal wells completed by inflow control devices in bottom-water reservoirs. J Nat Gas Sci Eng. 2015;27(3):1328–39. [Google Scholar]
9. Bai Y, Pu W, Jin X, Zhang S. Research on numerical simulation of water breakthrough characteristics and influencing factors after oil reservoir plugging to determine the variation pattern of water cut in such reservoirs: a case study of Y oilfield. Petrol Sci Technol. 2023;42(5):1–22. doi:10.1080/10916466.2023.2254338. [Google Scholar] [CrossRef]
10. Pan Y, Wang K, Tang C, Li K, Liu H. Research on the water production tracking method of horizontal well in heavy oil reservoir with bottom water. Lithosphere. 2021;2021(Special 1):2593788. [Google Scholar]
11. Okon AN, Appah D. Water coning prediction: an evaluation of horizontal well correlations. Eng Appl Sci. 2018;3(1):21–8. [Google Scholar]
12. Li K, Zhang J, Zhu G, Zhou W, Wang K, Liang H. A new method for calculating the characteristic shape of the water ridge under horizontal production wells in marine sandstone heavy oil reservoirs with bottom water. Chem Technol Fuels Oils. 2021;57(4):690–7. doi:10.1007/s10553-021-01294-z. [Google Scholar] [CrossRef]
13. Kuo MCT, DesBrisay CL. A simplified method for water coning predictions. In: Proceedings of the SPE Annual Technical Conference and Exhibition; 1983; San Francisco, CA, USA. SPE-12067-MS. [Google Scholar]
14. Wang Q, Liu H, Yin F, Lai S. The water control technique of horizontal well completion strings with an inner-located nozzle in a nonhomogeneous bottom water reservoir. Petrol Sci Technol. 2013;31(18):1873–80. doi:10.1080/10916466.2011.553646. [Google Scholar] [CrossRef]
15. Zhao X. Research and application of gravel-packing flow-regulation water-control screen completion technique in horizontal wells. IOP Conf Ser: Earth Environ Sci. 2019;227(4):042034. [Google Scholar]
16. Qin S, Zhang N, Cao B, An Y, Huo R. Mathematical modeling of prediction of horizontal wells with gravel pack combined with ICD in bottom-water reservoirs. Processes. 2023;11(9):2777. doi:10.3390/pr11092777. [Google Scholar] [CrossRef]
17. Jin L, Wojtanowicz A, Hughes R. An analytical model for water coning control installation in reservoir with bottom water. J Can Petrol Technol. 2010;49(5):65–70. doi:10.2118/137787-PA. [Google Scholar] [CrossRef]
18. Yang M, Li H, Wang Y, Jiang R. Numerical modelling for the optimum design of horizontal well completions with PSCs in water drive reservoirs. Arab J Sci Eng. 2019;44:5215–32. doi:10.1007/s13369-019-03790-1. [Google Scholar] [CrossRef]
19. Elverhøy AB, Aakre H, Mathiesen V. Autonomous inflow control for reduced water cut and/or gas oil ratio. In: Proceedings of the Offshore Technology Conference; 2018; Houston, TX, USA. OTC-28860-MS. [Google Scholar]
20. Zhang F, Wang H, Wang M. Research on influencing factors of production control valve used for water control in horizontal wells. Int J Model Identif Control. 2020;36(4):363–72. doi:10.1504/IJMIC.2020.117496. [Google Scholar] [CrossRef]
21. Luo W, Lin Y, Li H, Jiang Z, Wu Q, Tao Z. ICD completion optimization design of horizontal wells in heterogeneous bottom-water reservoirs. Acta Petrol Sin. 2017;38(10):1200–9. [Google Scholar]
22. Furui K, Zhu D, Hill AD. A new skin-factor model for perforated horizontal wells. SPE Drill Complet. 2008;23(3):205–15. doi:10.2118/77363-PA. [Google Scholar] [CrossRef]
23. Wang J, Dai J, Xie B, Du J, Li J, Liu H, et al. Gas injection capacity of slotted liner and perforation completion in underground natural gas storage reservoirs. Processes. 2023;11(5):1471. doi:10.3390/pr11051471. [Google Scholar] [CrossRef]
Cite This Article
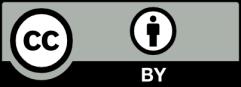
This work is licensed under a Creative Commons Attribution 4.0 International License , which permits unrestricted use, distribution, and reproduction in any medium, provided the original work is properly cited.