Open Access
ARTICLE
On the Use of Recycled Asphalt and Trinidad Lake Asphalt (TLA) for the Preparation of High Modulus Asphalt Concrete
1 Foshan Transportation Science and Technology Co., Ltd., Foshan, 528315, China
2 Key Laboratory of Road Structure and Material of Ministry of Transport (Changsha), Changsha University of Science & Technology, Changsha, 410114, China
* Corresponding Author: Chao Li. Email:
(This article belongs to the Special Issue: Advances in Solid Waste Processing and Recycling Technologies for Civil Engineering Materials)
Fluid Dynamics & Materials Processing 2023, 19(7), 1939-1950. https://doi.org/10.32604/fdmp.2023.026103
Received 16 August 2022; Accepted 27 October 2022; Issue published 08 March 2023
Abstract
Rutting of asphalt pavement occurs earlier and is more serious under the increasingly heavy traffic load conditions that can be found in subtropical monsoon climate regions. High modulus asphalt concrete (HMAC) with excellent anti-rutting and anti-fatigue properties is generally used to mitigate this issue. Given the relatively high cost of the additives used in this type of asphalt, in this study the feasibility of using recycled asphalt mixture (RAP) and Trinidad lake asphalt (TLA) for the preparation of HMAC is considered. The mineral composition of the RAP is first analyzed, then the TLA modified asphalt with different levels of RAP content is used to assess the effect of RAP on the performance of the mixture. Finally, the high-temperature rheological properties of extracted bitumen and the adhesion characteristic between mineral and bitumen are analyzed. The results show that after RAP combustion, the yellowish and greyish minerals display different alkalinity values, while the greyish mineral exhibits an improved ability to combine with bitumen. Thus, RAP can significantly enhance the high-temperature rutting resistance of TLA modified asphalt mixtures. The aged bitumen in RAP makes the mixed binder harder and improves its rheological properties at high temperatures.Keywords
In areas with subtropical monsoon climate, the annual average temperature of asphalt pavement can reach more than 20°C, and the maximum temperature inside the asphalt pavement in summer can reach above 70°C [1]. Under such high-temperature conditions, the bitumen in it would produce a viscous flow due to the action of the shear stress generated by vehicles driving over the paving, leading to permanent deformation of the road surface and cause rutting [2]. Rutting not only endangers driving safety and reduces driving stability [3], but also leads to early damage to the roadbed because of the accumulation of water in the potholes created by rutting [4].
In response to this situation, French road workers took the lead in inventing high modulus asphalt concrete (HMAC) in 1960 [3]. HMAC was a pavement material with a complex modulus ≥14000 MPa under the test conditions of 15°C and 10 Hz. It had excellent anti-rutting and anti-fatigue properties [5]. HMAC in China was defined as asphalt mixture with a dynamic stability greater than 4000 pass/mm [6]. At present, to meet the requirements of HMAC, researchers mainly adopt two methods: The first is to add hard asphalt (hard paving grade bitumen) with penetration of less than 30 dmm, then the overall structure of the mixture can be improved by the stabilizing effect of the binder. The second is to add high modulus additives to the mixture to reduce the rutting of the asphalt pavement [7]. However, under current conditions, there are still some difficulties in promoting the HMAC. Firstly, unlike the commonly used PG 76–22 bitumen, there is almost no hard asphalt on the market, and manufacturing solely for high-modulus asphalt mixtures is quite costly. Secondly, the price of high modulus additives on the market is generally higher, and there are still problems such as uneven dispersion, a large difference in material properties, and unstable properties when mixed with pure bitumen. Therefore, it is necessary to develop a high modulus asphalt mixture that is suitable for the current situation of road construction and usage.
Recycled asphalt mixture (RAP) is produced by the removal and milling of the surface layers of an existing asphalt pavement [8]. However, because the bitumen in the RAP has been severely aged, the penetration has dropped to below 30 dmm [9]. Although the lower penetration helps to enhance the high-temperature anti-rutting performance and meet the requirements of composite high-modulus asphalt concrete, the RAP-added mixture still lacks fatigue durability [10], and other materials need to be added to improve it.
Trinidad lake asphalt (TLA) is the world’s most famous, widely used natural bitumen [11]. Physical and rheological properties of TLA-modified bitumen showed that TLA could help to improve the stiffness [12], high-temperature stability [13], and low-temperature-induced cracks [14] of bitumen. Yang et al. investigated the high-temperature properties of TLA modified asphalt, and the results indicated that the modified asphalt had higher resistance to rutting deformation [15]. If TLA modified asphalt can be used in combination with RAP, the advantages of both can be further utilized to improve the performance of the asphalt mixture.
Therefore, this paper investigated the effect of RAP content on the performance of TLA modified asphalt. Firstly, by polarizing microscope and XRF, the compositions of the mineral in the RAP were analyzed. Then, the TLA modified asphalt mixtures with different contents of RAP were prepared to study the effect of RAP content on the performance of the mixture. Finally, the bitumen in various mixtures was extracted, the high-temperature rheological properties of extracted bitumen, and the adhesion characteristic between mineral and bitumen were analyzed.
2 Materials and Experimental Methods
The TLA modified bitumen used in this study was provided by Foshan Guangnan Asphalt Co., Ltd. Foshan, China. The technical properties of the TLA modified asphalt are presented in Table 1.
The coarse aggregates used in the project were produced by Jiujiang Huatai Mining Co., Ltd., China. The specifications of the coarse aggregate were 3–5, 5–10, 10–15, 10–20, 10–25 mm. The fine aggregates of 0–3 mm were produced by Dongguan Longsheng Asphalt Co., Ltd., China. The RAP was milled from the asphalt surfaces removed during highway maintenance with a particle size of 0–8, 8–13, 13–19 and 19–25 mm. The properties of RAP and extracted binder from RAP are shown in Table 2.
2.2 Characterization of Aged Minerals in RAP
Firstly, the aged minerals in RAP were obtained by combustion. Then, through cutting, grinding, polishing, etching, etc., flakes of different minerals were obtained for polarizing microscope testing. Polarizing microscope uses the basic characteristics of crystal birefringence to convert ordinary light into polarized light, and through a polarizer to identify whether a material exhibits single refraction (isotropy) or birefringence (anisotropy), to judge the lithofacies composition of the minerals. The test instrument was an Eclipse E200 polarizing microscope manufactured by Nikon, Japan, set to a magnification of 200X.
Finally, the minerals were ground into powdered samples with a particle size of less than 0.075 mm and subjected to X-ray fluorescence (XRF) analysis to obtain the elemental composition. XRF uses primary X-ray photons or other microscopic ions to excite atoms in the substance to be tested, causing the substance to generate fluorescence (secondary X-rays), to conduct substance composition analysis and chemical state research. The test instrument was an XRF-1800 manufactured by Shimadzu, Japan.
2.3 Preparation of the Asphalt Mixture
To analyse the influence of RAP content on the performance of TLA modified asphalt mixture, three types of AC-20 asphalt mixture were designed. They were named TMAM, TMAM-20% RAP, and TMAM-40% RAP, respectively, where the mass content of RAP was set to 0%, 20%, and 40%, respectively. The graduation curves are shown in Fig. 1.
Figure 1: Gradation curves of used asphalt mixtures
The asphalt contents of all asphalt mixtures were 4.4%, and the contents of aged bitumen and TLA modified bitumen are shown in Table 3. The preparation process of TMAM is shown below: the TLA modified bitumen and RAP were preheated at 160°C and 130°C for 2 h, aggregates were preheated at 200°C for 4 h firstly. Then, RAP and aggregates were mixed at 175°C for 60 s, then TLA modified bitumen was added and mixed for 120 s. After former procedures, different types of TMAM were prepared and could be used to form samples for different tests.
2.4 Properties of Asphalt Mixture
In this paper, the moisture resistance and high-temperature rutting resistance of TMAMs were studied. Based on the T0710-2011 method, a wheel-track device was performed on the TMAMs, and the dynamic stability (DS) values of TMAMs were obtained from tests to evaluate the high-temperature rutting resistance. The temperature values were set as 60°C and 70°C. Based on the T0719-2011 and T0729-2011 methods, the ratio (MS0) of the Marshall stability of 48 h immersion (MS1) to the Marshall stability of 0.5 h of immersion (MS), and the ratio (TSR) of split tensile strength ratio after freeze-thaw cycle (RT2) to split tensile strength ratio before freeze-thaw cycle (RT1) were obtained. The MS0 and TSR values were used to evaluate the moisture resistance. Based on the T0715-2011 method, the low-temperature bending beam tests were conducted on the TMAMs, the maximum bending tensile strain (εB) was used to evaluate the low-temperature performance of TMAMs. The test temperature was −10°C, and the loading rate was 50 mm/min.
2.5 High-Temperature Rheological Properties of Bitumen
High-temperature rheological properties of extracted bitumen from different asphalt mixtures were studied by the Dynamic Shear Rheometer (DSR) based on ASTM D7175-08. The high-temperature sweep analysis was performed between 40°C and 100°C. To ensure that the entire measurement process was carried out within the linear viscoelastic range of bitumen, based on previous work [18], a temperature sweep analysis test was performed at a fixed frequency of 10 rad/s and a fixed strain of 0.5%.
As shown in Fig. 2, after burning RAP, it is found that the mineral materials can be divided into two colors, one is grayish, and the other is yellowish. To find the difference between them, the lithofacies analysis was carried out.
Figure 2: Images of grayish RAP (a) and yellowish RAP (b)
The results show that the two types of minerals are both olivine basalt. As shown in Fig. 3, the main mineral of the first type consists of plagioclase, pyroxene, and a small amount of olivine. The accessory mineral is mainly magnetite, and the altered mineral is hematite. Plagioclase is self-shaped lath-like crystallites, which are arranged in an interlaced arrangement, with the gaps filled with pyroxene and a small amount of olivine. Pyroxene is columnar, and olivine is either granular- or elliptical-shaped. Reddish-brown secondary hematite is filled in mineral cleavage cracks or grain boundaries.
Figure 3: Lithofacies composition of grayish mineral
Fig. 4 shows the second type with a porphyritic structure. The phenocrysts are mainly olivine and pyroxene; the matrix is mainly composed of plagioclase and a small amount vitreous. Olivine is heterogeneously granular or oval, and almost all hematite is mineralized, with only a few remnants. Plagioclase is self-shaped lath-like crystallites, interwoven and arranged, and the gaps are filled with fine-grained pyroxene and a small amount of glass. The accessory minerals mainly include magnetite, altered mineral hematite, and post-magmatic alteration product quartz.
Figure 4: Lithofacies composition of yellowish mineral
The reason for the difference in aggregate color is that although the two types of minerals are a kind of stone, due to the changes in the initial mixing, compaction, and use process, the thickness of asphalt film on the mineral’s surface is different, and the adhesion between the materials is also different. After burning, the surface of the mineral material with a larger film thickness and strong adhesion has more combustion products, while the surface of the mineral material with a smaller film thickness and general adhesion shows the original color of aggregates.
It can be seen from Table 4 that the elemental compositions of the two different colored minerals are quite similar, and both mainly contain silicon, aluminum, iron, calcium, magnesium, sodium, and other elements. The results are also consistent with the lithological analysis. From the previous research [19], the alkalinity M can be calculated based on the following equation:
where, M represents the alkalinity, wCaO, wSiO2 and wP2O5 represent the mass fractions of calcium oxide, silicon dioxide and phosphorus pentoxide, respectively, which can be obtained from the XRF analysis. The results indicated that the alkalinity values of grayish mineral and yellowish mineral are 2.03 and 1.81. In general, aggregates with higher alkalinity have better adhesion to bitumen [20,21]. Therefore, the greyish mineral has the potential to combine better with bitumen.
3.2 High-temperature Rutting Resistance of Asphalt Mixture
As presented in Table 5, the dynamic stability values of the TMAM at 60°C are all greater than the 4000 pass/mm and far higher than what is required by the HMAC specification [5], indicating that the addition of TLA modified bitumen improves the high-temperature stability of the asphalt mixture. Regardless of whether it is at 60°C or 70°C, with an increase of RAP content, the dynamic stability values of the TMAM gradually increase, demonstrating that the incorporation of RAP can improve the high-temperature stability of the TLA modified asphalt mixture.
At 60°C, the dynamic stability of the TMAM-20% RAP is increased by 31.41% more than that of the TLA modified asphalt mixture without RAP, while the dynamic stability of the TMAM-40% RAP is increased by 14.71% compared with that of the TMAM-20% RAP. These results show that RAP can significantly enhance the high-temperature stability of TMAM. This is because, firstly, the physical and chemical properties of TLA and RAP are the same as ordinary asphalt, with good compatibility and no segregation. Secondly, TLA contains about 54% bitumen, 36% minerals and 10% other useful substances. The minerals are very small, 90% of the minerals have a particle size of less than 0.075 mm [22], and the aged bitumen in RAP is also relatively hard, both of which can improve the softening point and wear resistance of the asphalt. Finally, both RAP and TLA can increase the thickness of the asphalt mold and increase the friction coefficient of the road surface.
3.3 Low-Temperature Performance of Asphalt Mixture
As shown in Table 6, the εB values of TMAM and TMAM-20% RAP can meet the requirements, while the εB value of TMAM-40% RAP is lower than the requirements. With the increase of RAP content, the εB values of asphalt mixture decrease by 10.73% and 25.99%, respectively. Results indicate that the addition of RAP is not conducive to the low-temperature performance of the asphalt mixture, and TMAM with RAP is more suitable for use in areas where low temperature performance requirements are not strict.
3.4 Moisture Resistance of Asphalt Mixture
As presented in Table 7, the water stability of the TMAMs meet the technical requirements. After adding RAP, the water immersion residual stability and the freeze-thaw splitting test strength ratio of the asphalt mixture increase, indicating that the moisture resistance of TMAM-20% RAP and TMAM40% RAP are better than that of TMAM. With the increase of RAP content, the water immersion residual stability of asphalt mixture increased by 2.36% and 0.67%, respectively, and the freeze-thaw split test strength was increased by 3.11% and 1.73%, respectively. Although the addition of RAP helps to improve the moisture resistance of the mixture, the property does not show a trend of increasing with the augment of RAP content. This may be due to the presence of two different types of minerals in RAP, which have different effects on the moisture resistance of the mixture.
3.5.1 High-Temperature Rheological Properties of Bitumen
To characterize the effect of RAP content on the high-temperature rheological properties of TLA-modified bitumen, DSR was used to conduct temperature scanning tests on the extracted bitumen from different asphalt mixtures. DSR measures the complex modulus (G*) and phase angle (δ) to evaluate the viscoelastic performance of the bitumen under repeated shear loads. G* can be calculated from the ratio of the shear stress limit value to the shear strain limit value, and it can be decomposed into two parts: the storage modulus of the elastic part (G’ = G*⋅cosδ) and the loss modulus of the viscous part (G’’ = G*⋅sinδ). A larger G’ represents a better ability of bitumen to recover from deformation, while a larger G’’ means a better ability of the bitumen to resist deformation. Therefore, a larger G* means that the asphalt material has greater stiffness and better resistance to flow and deformation in high-temperature environments, which allows the asphalt mixture to have better resistance to deformation and rutting. The other main parameter, the phase angle δ, is the arctangent value of G’’ and G’, which can also be used to characterize the viscoelastic performance of the asphalt. A smaller δ means a better elastic performance of the asphalt, and a larger δ means that the viscosity performance of the asphalt is better. In a high-temperature environment, the modified asphalt with larger δ has poor resistance to deformation and is more prone to permanent deformation. As shown in Fig. 5, at 40°C, the G* and δ of the extracted bitumen from TMAM are 234000 Pa and 74.63°. After the addition of RAP, the G* increases rapidly with the increase of the dosage, while the δ follows is progressively smaller.
Figure 5: High-temperature sweep analysis of different bitumen
The ratio of G* to the sine of the phase angle is the rutting factor. The rutting factor can characterize the high-temperature stability of the asphalt mixture, and the higher the rutting factor, the better the high-temperature resistance. The rutting factor test curves of the different bitumen samples are shown in Fig. 6. The changing trend is consistent with the changing trend of the complex modulus. The test value decreases parabolically with increase in temperature. The initial rutting factor of extracted bitumen from TMAM at 40°C is 243000 Pa. The initial rutting factor values of bitumen extracted from TMAM-20%RAP, bitumen extracted from TMAM-40%RAP are 416664, and 590598 Pa, respectively. At a test temperature of 100°C at the end of the test, the final rutting factor values were 92.77, 162.17, and 231.56 Pa, respectively.
Figure 6: Rutting factor of different types of extracted bitumen
The rutting factor test results at six selected temperatures of 58°C, 64°C, 70°C, 76°C, 82°C, and 88°C are shown in Table 8. At all the six temperature points, the rutting factor of the extracted bitumen from TMAM remained the lowest, while the rutting factor of the extracted bitumen from TMAM-40% RAP was the highest.
These results show that aged bitumen makes the TLA-modified bitumen harder and improves its high-temperature rheological properties. When the amount of RAP is increased, the modified asphalt can have stronger high-temperature stability, which helps to reduce the occurrence of rutting under high-temperature conditions.
3.5.2 Adhesion Characteristic between Mineral and Bitumen
In order to evaluate the adhesion characteristic between the bitumen and minerals, the new mineral selected in this paper and the aged minerals of two colors were mixed with three kinds of extracted bitumen, and the adhesion tests were carried out according to T0616-1993 [16].
From the results presented in Table 9, it can be seen that the various combinations of the new mineral, the aged mineral and the extracted bitumen have adhesion properties above grade 4, showing good bonding performance. On the one hand, after the long-term service of the existing pavement, the surface of the minerals is affected by the vehicle load and asphalt erosion, the appearance tends to be rough, and the specific surface area increases, which is conducive to the combination of the bitumen and the mineral. On the other hand, after the bitumen is aged, the content of heavier components such as asphaltene increases, which increases the viscosity of the aged bitumen. Among them, the adhesion grade of the combination of the yellowish mineral and bitumen extracted from TMAM-20% RAP is lower than other combinations, and as shown in Fig. 7, about 10% of the surface of the mineral shows the phenomenon of asphalt spalling. The results are consistent with the XRF test, as the alkalinity of the yellowish mineral is lower than that of the grayish mineral, resulting in a lower adhesion of the yellowish mineral to the bitumen than other combinations. In addition, although the aged bitumen is helpful to improve the moisture resistance of TMAM, the difference in mineral composition of RAP in the TMAM also affects the moisture resistance such that it does not always increase with the amount of added RAP.
Figure 7: Pictures of results for different adhesion levels
This paper investigated the effects of RAP content on the performance of TLA-modified asphalt mixture, and the following conclusions can be drawn:
● Lithology analysis shows the presence of yellowish and greyish minerals after RAP combustion, with alkalinity values of 2.03 and 1.81, respectively. The greyish mineral with lower alkalinity has the potential to combine better with bitumen.
● The incorporation of RAP can improve the high-temperature rutting resistance of the TMAM. Although the addition of RAP helps to improve the moisture resistance of TMAM, the property does not show a trend of increasing with the increasing augmentmentation with RAP. RAP is not conducive to the low-temperature performance of the TMAMs.
● The aged bitumen in RAP makes the mixed binder harder and improves its high-temperature rheological properties, which helps to reduce the occurrence of rutting under high-temperature conditions. The difference in mineral composition of RAP in the TMAM causes effect that the moisture resistance does not always increase with the amount of added RAP.
Acknowledgement: The work was financially supported by Foshan Transportation Science and Technology Co., Ltd., Changsha University of Science & Technology.
Funding Statement: This work was funded by the Self-Financing Technology Plan Project of Foshan (2020001005386), Open Fund of Key Laboratory of Geological Survey and Evaluation of Ministry of Education (No. GLAB2019ZR01).
Conflicts of Interest: The authors declare that they have no conflicts of interest to report regarding the present study.
References
1. Ye, Z., Song, S., Zhong, R. (2022). Temporal and spatial evolution of climate comfort and population exposure in guangdong province in the last half century. Atmosphere, 13(3), 502. [Google Scholar]
2. Li, Y., Wu, S., Pang, L., Liu, Q., Wang, Z. (2018). Investigation of the effect of Mg-Al-LDH on pavement performance and aging resistance of styrene-butadiene-styrene modified asphalt. Construction and Building Materials, 172, 584–596. [Google Scholar]
3. Chen, Y., Wang, H., Xu, S., You, Z. (2019). High modulus asphalt concrete: A state-of-the-art review. Construction and Building Materials, 237, 117653. [Google Scholar]
4. Benta, A., Duarte, C., Almeida-Costa, A., Cordeiro, T., Pereira, R. (2015). Design and performance of a warm high-modulus asphalt concrete. Journal of Cleaner Production, 95(15), 55–65. [Google Scholar]
5. Abhijith, B., Narayan, S. (2021). Evolution of complex modulus and higher harmonics of stress response of asphalt concrete in strain-controlled four-point beam fatigue tests. International Journal of Pavement Engineering, 22(3), 18–23. [Google Scholar]
6. Wang, S., Li, I., Yue, J. (2015). Technology application research of high modulus asphalt concrete. Highways and Transportation in Inner Mongolia, 15(2), 12–16. [Google Scholar]
7. Li, P., Zheng, M. L., Wang, F., Che, F., Li, H. Y. et al. (2017). Laboratory performance evaluation of high modulus asphalt concrete modified with different additives. Advances in Materials Science and Engineering, 20(1), 1–14. [Google Scholar]
8. Zaumanis, M., Arraigada, M., Poulikakos, L. (2020). 100% recycled high-modulus asphalt concrete mixture design and validation using vehicle simulator. Construction and Building Materials, 260(10), 119891. [Google Scholar]
9. Prosperi, E., Bocci, E. (2021). A review on bitumen aging and rejuvenation chemistry: Processes. Materials and Analyses. Sustainability, 13(9), 20–25. [Google Scholar]
10. Wróbel, M., Woszuk, A., Ratajczak, M., Franus, W. (2021). Properties of reclaimed asphalt pavement mixture with organic rejuvenator. Construction and Building Materials, 271(4), 121514. [Google Scholar]
11. Rui, L., Wang, P., Xue, B., Pei, J. (2015). Experimental study on aging properties and modification mechanism of trinidad lake asphalt modified bitumen. Construction and Building Materials, 101(22), 878–883. [Google Scholar]
12. Fengler, R., Osmari, P., Leite, L. (2019). Impact of the addition of trinidad lake asphalt (TLA) on the rheological and mechanical behavior of two asphalt binders. Road Materials and Pavement Design, 20(2), 827–840. https://doi.org/10.1080/14680629.2019.1633789 [Google Scholar] [CrossRef]
13. He, R., Zheng, S., Chen, H., Kuang, D. (2019). Investigation of the physical and rheological properties of trinidad lake asphalt modified bitumen. Construction and Building Materials, 203(10), 734–739. https://doi.org/10.1016/j.conbuildmat.2019.01.120 [Google Scholar] [CrossRef]
14. Koodziej, K., Bichajo, L., Siwowski, T. (2021). Experimental study on physical and rheological properties of trinidad lake asphalt modified binder. Applied Sciences, 11(6), 2796. https://doi.org/10.3390/app11062796 [Google Scholar] [CrossRef]
15. Yang, E., Zhang, X. (2014). The study of high temperature performance of asphalt modified by trinidad lake asphalt. Advanced Materials Research, 831, 387–392. https://doi.org/10.4028/www.scientific.net/AMR.831.387 [Google Scholar] [CrossRef]
16. JTG E20-2011 Standard test methods of bitumen and bituminous mixtures for highway engineering (2011). [Google Scholar]
17. JTG F40-2004 Technical specification for construction of highway asphalt pavements JTG F40—2004 (2004). [Google Scholar]
18. Li, Y., Wu, S., Dai, Y., Pang, L., Liu, Q. (2018). Investigation of sodium stearate organically modified LDHs effect on the anti aging properties of asphalt binder. Construction and Building Materials, 172, 509–518. https://doi.org/10.1016/j.conbuildmat.2018.03.181 [Google Scholar] [CrossRef]
19. Li, C., Xiao, Y., Wu, S., Chen, Z. (2017). Crack resistance of asphalt mixture with steel slag powder. Emerging Materials Research, 10(2), 20–25. https://doi.org/10.1680/jemmr.16.00009 [Google Scholar] [CrossRef]
20. Chen, Z., Leng, Z., Jiao, Y., Xu, F., Lin, J. et al. (2022). Innovative use of industrially produced steel slag powders in asphalt mixture to replace mineral fillers. Journal of Cleaner Production, 20(2), 344–347. https://doi.org/10.1016/j.jclepro.2022.131124 [Google Scholar] [CrossRef]
21. Xie, J., Wu, S., Zhang, L., Xiao, Y., Liu, Q. et al. (2017). Material characterization and performance evaluation of asphalt mixture incorporating basic oxygen furnace slag (BOF) sludge. Construction and Building Materials, 147(5), 362–370. https://doi.org/10.1016/j.conbuildmat.2017.04.131 [Google Scholar] [CrossRef]
22. Koodziej, K., Bichajo, L., Siwowski, T. (2021). Effects of aging on the physical and rheological properties of trinidad lake asphalt modified bitumen. Materials, 14(10), 24–28. https://doi.org/10.3390/ma14102532 [Google Scholar] [PubMed] [CrossRef]
Cite This Article
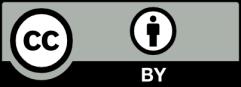
This work is licensed under a Creative Commons Attribution 4.0 International License , which permits unrestricted use, distribution, and reproduction in any medium, provided the original work is properly cited.