Open Access
ARTICLE
Effect of Al2O3 Nanoparticles on the Compression Ignition Performances and Emitted Pollutants of a Diesel Engine
1
Electromechanical Engineering Department, University of Technology-Iraq, Baghdad, 65035, Iraq
2
Environment Research Center, University of Technology-Iraq, Baghdad, 65035, Iraq
3
Applied Science Department, University of Technology-Iraq, Baghdad, 65035, Iraq
4
Energy and Renewable Energies Technology Center, University of Technology-Iraq, Baghdad, 65035, Iraq
* Corresponding Author: Miqdam T. Chaichan. Email:
Fluid Dynamics & Materials Processing 2023, 19(11), 2847-2861. https://doi.org/10.32604/fdmp.2023.028874
Received 12 January 2023; Accepted 14 March 2023; Issue published 18 September 2023
Abstract
To improve the performances of diesel engines and to reduce the pollutants they emit, aluminum oxide nanoparticles in varying quantities (50, 100, 150 ppm) have been added to conventional diesel fuel. The results of such experimental tests have revealed that the addition of nano-Al2O3 particles to the diesel fuel reduces its consumption by 0.488%, 1.02%, and 1.377%, respectively and increases the brake thermal efficiency by 1.4%, 2.6%, and 3.8%, respectively. The concentrations of undesired gases decrease accordingly by 1.5%, 1.7%, and 2.8% for HC and by 5.88%, 11.7%, and 17.6%, respectively, for CO. For the same percentages of nanoparticles, NOx increases by 2.65, 4.36, and 5.37, while the TSP (Total Suspended Particulate) decreases by 22%, 34.66%, and 49.7%, respectively. In the same conditions, sulfur dioxide pollutants increase by 4.57%, 8.8% and 12.89%, and H2S levels by 4.7%, 7.81% and 8.9%, respectively.Keywords
Nomenclature
Al2O3 | Aluminum oxide |
HC | Unburnt hydrocarbons |
BMEP | Brake mean effective pressure |
H2S | Hydrogen sulfide |
BSFC | Brake specific fuel consumption |
NOx | Nitric oxides |
CO | Carbon monoxide |
PM | Particulate matter |
CO2 | Carbon dioxide |
ppm | Part per million |
CuO2 | Copper oxide |
SO2 | Sulfur dioxide |
For over fifty years, researchers and manufacturers have been in global consensus about reducing diesel brake specific fuel consumption (BSFC) and improving its combustion properties, to combat the real dangers to humanity posed by the excessive use of fossil fuels in the production of electric power and transportation. This is especially significant, as transport accounts for nearly 40% of the total pollutants emitted around the world. Numerous practical and theoretical solutions have been suggested since the end of the last century to address this issue. Adding fuel additives to fuels can alter their physical and chemical characteristics and boost performance and emission levels. These additives can include oxygenating materials like methanol, ethanol or biodiesel [1,2]. Additionally, a blend of diesel and water is sometimes employed to create a suspension [3]. Researchers have also explored the use of nanoparticles in diesel for similar purposes [4,5]. The result of these additives is an altered composition of the fuel, better combustion properties, and a drop in pollutants released.
Since the inception of their production, nanoparticles have become a prominent option for use in internal combustion engines because they have an ability to improve the fuel’s thermal conductivity. Higher thermal conductivity of fuel means less delay period, which expedites the conversion of fuel droplets into vapor that easily mixes with air. Thus, their application has shown promise in improving engine pollutants and emissions.
The addition of nanoparticles to diesel, biodiesels, or diesel-water emulsions can improve engine performance and decrease the emission of pollutants as suggested by many research articles [6,7]. If additionally implemented, fuel cetane number and heating value could be improved; however, some important parameters such as fuel flash point temperature, density, and viscosity are increased, which has a negative effect on fuel combustion [8,9]. It is theorized that nanomaterials can increase efficiency in combustion engines by decreasing the ignition delay period, accelerating the fuel burning process, and raising the released heat rate due to the increased pressure inside the combustion chamber [10,11]. Nanoparticles can lead to a decrease in the cloud point temperature and improved operational performance in colder temperatures. It has greater surface area-to-size ratio, which results in a better combustion process [12,13]. Studies have indicated that the incorporation of nanoparticles into the fuel-water emulsion can result in an increase in secondary explosions, which can lead to improved engine performance and reduced emissions [14]. In a study conducted by Sathiamurthi et al. [15] the effect of blending nano-Al2O3, also known as nano-aluminum oxide or nano-alumina, with diesel on engine performance and the amount of pollutants emitted was examined. The scientists infused 0.5 and 1 g of nano-Al2O3 into the fuel line while subjecting it to a magnetic field. The findings revealed a decrease in carbon dioxide levels by 52% and 57% respectively when compared to diesel alone. Without the use of a magnetic field, the levels of CO2 for the two cases of adding 0.5 and 1 g of nano-alumina to diesel were 22% and 52%, respectively. When 0.5 g of nano-Al2O3 was mixed with diesel under the influence of a magnetic field, the BSFC went down by 13% in comparison with diesel alone, while the reduction was 7% for the situation of adding 1 g of nano-Al2O3 [15].
In Fayad et al.’s study [16], they discovered that adding nano-CuO2 to rapeseed methyl ester (RME) and diesel fuel increased the brake thermal efficiency (BTE) by 23.6% and reduced BSFC by 7.6% when the engine speed was varied. Alawi et al. examined the consequences of including nano-Al2O3 in diesel on a compression ignition engine outcome. Quantities corresponding to 100 and 150 ppm of nano-Al2O3 were added. An ultrasonication was employed to mix the nanoparticles with the diesel. The measurements revealed that as the amount of nano-Al2O3 in the blend increased, the BTE and BSFC were improved. The measurements of exhaust emissions across all loads revealed that particulate matter (PM) and nitric oxides (NOx) levels emitted by the nano-Al2O3 suspensions were lower than those produced by diesel fuel alone [17]. Fayaz et al. carried out a study to evaluate the impact of nano-Al2O3 in diesel fuel. The results indicated a rise in exhaust gas temperature and BTE by 9.83% as well as decrements in BSFC and carbon monoxide (CO), hydrocarbons (HC), and nitric oxides (NOx) emissions of about 5.98%, 27.89%, 30.68% and 10.37%, respectively [18]. Venkatesan et al. investigated the possibility of improving the BTE and decreasing the levels of HC, NOx, smoke, and CO emissions by incorporating alumina nanoparticles into diesel fuel. They examined the impacts of 100 and 150 ppm nano-Al2O3 on engine performance and emitted pollutants at a fixed engine speed of 1500 rpm and 400 bar injection pressure with various operating loads (6, 1.2, 2.4, 3, 3.5 bar). The tests’ measurements indicated that the nanoparticles addition led to a reduction in BSFC, higher BTE by 2.9%, and a decrease in all of the emissions CO, HC, soot and NOx by about 28.57%, 36.17%, 16.9%, and 30.67%, respectively [19].
In the Nouri et al. (2021) study, the influence of adding Al2O3 and Fe2O3 nanoparticles in mass fractions of 30, 60, and 90 ppm on the engine’s outcome characteristics was studied. The measurements showed that the introduction of Fe2O3 nanoparticles was more successful in increasing brake power and torque than incorporating nano-Al2O3, while nano-Al2O3 particles caused a reduction in BSFC and enhanced the BTE. As the concentration of the Al2O3 nanoparticles was increased, CO emission dropped by 21%, and a considerable decrease (up to 24%) in NOx emission was observed [20]. Using a two-step esterification process, [21] produced biodiesel from leachate oils and added nanoparticles of aluminum oxide and copper oxide. According to Khan et al. [22], engine performance and pollutant emissions were affected by the use of nanofuels when injection pressure was changed. Copper (III) oxide was added to a mixture of biofuel derived from waste plants (Eichhornia crassipes) mixed with diesel at a ratio of 30:70. In addition to improving maximum peak pressure, the proposed fuel accelerates the rate of heat release. When nanoparticles were added to the diesel-biodiesel blend with increasing injection pressures, HC emissions were reduced by 14% and NOx and PM emissions were reduced by 15%.
Chen et al. [23] ran experiments on a compression ignition engine (CIE) to examine the influence of Al2O3, carbon nanotubes and SiO2 nanoparticles mixed with diesel. Outcomes showed that BSFC was reduced by a maximum of 20% and BTE was enhanced by 19%. Additionally, nano-SiO2-diesel suspensions were more successful than nano-Al2O3 suspensions due to the enhancement of BSFC, BTE and CO emitted. Yet, blending diesel with carbon nanotubes raised NOx emissions [23]. Dhahad et al. tested the effects of mixing nano-ZnO and nano-Al2O3 with high sulfur content (Iraqi origin) diesel on the engine’s performance and emissions. No exhaust gas post-treatment equipment was used. Before the tests, the fuel and nanoparticles were suspended in determined amounts (50 and 100 ppm) and the suspensions were tested. It was revealed that SFC decreased by 8% while BTE was raised by 6% when nanoparticles’ added mass fraction was increased. As the nanoparticle dose increased, the effect intensified. In addition, CO, HC, and all types of PM were decreased with nanoparticle addition by 17%, 17%, and 26%, respectively. On the other hand, due to temperature generated increase inside the cylinder as a consequence of nanoparticle addition, NOx levels were increased about 10% [24].
Adzmi et al. [25] concluded that the use of nano-Al2O3 and nano-SiO2 suspensions in palm oil methyl ester (POME) increased the maximum pressure, peak torque, and engine power by 16.3%, 43%, and 44%, respectively, when compared to neat POME combustion tests. The study further revealed that the suspensions yielded better outcomes than neat POME. The emissions of nanoparticles fuel were also reduced by 10% for NOx, 6.3% for CO2, and 0.02% for CO. Channappagoudra [26] explored the impact of adding nano-aluminum oxide to diesel on both customized and unmodified diesel motors performance. It was determined that at maximum load, the BTE was increased by 9.33% and the heat release rate rose by 13.56% when the modified engine ran on a B2O-Al2O3+75 ppm fuel mixture in comparison to the baseline engine that ran solely on B20 fuel. Additionally, the modified engine running on the B20–75 ppm Al2O3 fuel decreased the BSFC by 16.56%, the HC by 22.5%, and the CO emission by 20.78%, in comparison to the baseline engine running solely on B20 fuel [26].
Previous studies have largely focused on low or ultra-low sulfur diesel, which is used in most countries all over the world. However, in some developing countries like Iran, Pakistan, Afghanistan and Iraq, diesel with high sulfur content is still used till today [27]. Sulfur in fuel causes deterioration in fuel combustion and an increase in certain types of emissions, such as smoke and hazardous sulfur dioxide. Since removing the sulfur from fuel during distillation is a costly process, if the combustion process can be improved qualitatively (as in this study by adding nanoparticles to the fuel), this will reduce the effects of sulfur. This study examines the impact of adding nano-Al2O3 to conventional diesel fuel on BSFC and the resulting emissions. Iraqi diesel falls in the worst fuel category in the world, with a sulfur content of 1%–2.5% of the mass fraction. This fuel emits hazardous pollutants which are detrimental to human, animal, and planetary health. Engine performance parameters and emission levels were measured while the engine was run at varying loads and a constant speed. The goal of this work is to understand the influence of added nanoparticles on SO2 and H2S exhaust, alongside the NOx-PM tradeoff. Additionally, an effort is made to determine the mass fraction range of added nanoparticles which is most beneficial for diesel.
For this study, a four-cylinder, water-cooled, Fiat direct-injection diesel motor, was implemented. The specifications of the engine are listed in Table 1. A hydraulic dynamometer was employed to control the engine’s loads. Air is drawn into the combustion chamber from an air tank that eliminates pressure pulses in the air system. The consumed fuel is determined by timing how long it takes the engine to consume 200 ml of diesel. Fig. 1 shows a schematic diagram of the tested engine.
Figure 1: Schematic diagram of the engine rig
The addition of Nano-Al2O3 (the specifics of which are listed in Table 2) to Iraqi diesel fuel has led to the formation of a nanofluid. The investigation of developing a nanofluid with prolonged stability, where the thermophysical characteristics remain unchanged, is still in progress globally. As a result, the thermophysical properties of any developed nanofluid must be tested to verify its stability.
In this study, nano-Al2O3 was added to conventional Iraqi diesel employing an ultrasonic mixer. The mixing ran for three and a half hours continuously, in accordance with the results of Al-Waeli et al. [28], to ensure that the nanoparticles remained suspended in the mixture. The stability of the suspensions decreases with increasing the size of the nanoparticles or the added mass fraction. The nano-Al2O3 used is characterized by its low cost and availability in the local market. Surfactants were added to increase the stability of the fuel in very small amounts that do not affect the combustion properties of the suspension but increase the fluid stability. The added surfactant is ammonium cetyl cetyl (CTAB), based on the study of Jawad et al. [29]. Table 3 lists the specifications of the prepared fuel suspension.
Over a period of six months, the Zetasizer Nano (ZSN) was used to examine the stability of samples of suspensions of 50, 100, and 150 ppm of nano-Al2O3 that had been mixed by an ultrasonic shaker (Telsonic Ultrasonics CT-I2). A HOT DESK Tps 500 (KIJTALEY, Sweden) was employed to evaluate the thermal conductivity of the studied suspensions at weekly intervals. The viscosity was measured using a Brookfield LVDV-III viscometer, and a density meter for measuring density. To ensure accuracy and reliability, the measurements were repeated three times per test. An Emissions Analyzer (Model 4880) was used to detect the levels of CO2, CO, NOx, and HC. The G460 (made in Germany) was used to evaluate the amount of SO2 and H2S that were released from the engine exhaust. The GT-521 met model was employed to measure the total suspended particles discharged.
Due to the importance of fuel stability and the suspension of nanoparticles in diesel as long as possible, samples of fuel mixtures were sorted, and the sedimentation of nanoparticles was monitored daily. The results showed that the start of clear precipitation in the nanoparticles began after 73, 71, and 68 days for the samples of nano-Al2O3 additives at fractions of 50, 100, and 150 ppm, respectively.
The following equations were used to assess the engine’s performance qualities [30]:
Brake power (kW):
Brake means effective pressure (kN/m2):
Fuel mass flow rate (kg/sec):
Air mass flow rate (kg/sec):
BSFC (kg/kW.hr):
Total fuel heat (kW):
BTE (%):
The Gaussian equation was utilized to assess the uncertainty in the tests [31]:
In Table 4, the measured engine performance and emissions variables are assigned. Also, the thermophysical parameters tests’ uncertainty are added. The final uncertainty is 2.4%, which is ergonomically acceptable. It also demonstrates the accuracy and acceptability of the results obtained during the study.
3.1 Brake Specific Fuel Consumption
A study was conducted using a diesel engine to analyze the BSFC experiments, with pure diesel and three different mass fractions of nano-Al2O3 (50, 100, 150 ppm). The load on the diesel engine was adjusted from 50 to 350 kN/m2 while keeping the speed at 1500 rpm during each experiment.
Fig. 2 shows that when the load was decreased, the BSFC decreased for both diesel and the nano-emulsions blends. Furthermore, when the nano-Al2O3 concentration was increased from 50 to 150 ppm, BSFC was also reduced. This is attributed to the reduced ignition delay of the nanoparticles, which can be attributed to their increased surface area to volume ratio. Additionally, when the nanoparticle mass fraction was increased, BSFC went down at any given load. The addition of 50, 100, and 150 ppm of nano-Al2O3 to diesel resulted in a decrease of 0.488%, 1.02%, and 1.377% in BSFC, respectively. This decrease in BSFC can be attributed to the higher calorific value of the fuel due to the addition of nanoparticles, as well as the higher engine brake power that resulted. The fuel’s thermal conductivity was also increased while its delay period was reduced as well as its consumption at fixed loads. This implies a more efficient use of the fuel’s energy.
Figure 2: Effect of the mass fraction of added nanoparticles on the BSFC at variable engine loads
Fig. 3 shows the influence of the variation of engine load on the shift in BTE. As the engine load increased, the thermal proficiency also increased, and the mass fraction of nanoparticles used as fuel did too. The results for the suspensions were comparable to the BSFC results. This makes sense as the BTE is directly connected to BSFC.
Figure 3: Effect of the mass fractions of added nanoparticles on BTE at variable engine loads
Significantly greater BTE was achieved when the nanoparticle concentration was higher. The addition of 50, 100, and 150 ppm nano-Al2O3 to diesel resulted in higher BTE by 1.4%, 2.6%, and 3.8%, respectively. However, further experiments using higher concentrations of nano-Al2O3 must be carried out to fully understand the behaviors of the suspensions.
3.3 Carbon Monoxide and Unburnt Hydrocarbons
Figs. 4 and 5 show how the CO and HC emissions vary with engine load as different fuel mixtures are used. As the load rises, the CO and HC levels go down. When nano-Al2O3 is mixed in with the fuel at a concentration of 150 ppm, the combustion is improved, leading to reduced CO and HC emissions by 17.6% and 2.8%, respectively. This is likely due to the increased thermal conductivity, which allows for a more efficient burning of the fuel. These results are consistent with references [32–34] results.
Figure 4: Effect of the mass fractions of added nanoparticles on emitted CO levels at variable engine loads
Figure 5: Effect of the mass fractions of added nanoparticles on emitted HC levels at variable engine loads
Fig. 6 shows the influence that engine load has on NOx emission when various nanofuels mixtures were employed. As the engine load increased, the NOx emission also increased due to the higher temperature created within the combustion chamber from the additions of nanoparticles. The addition of nanoparticles to diesel cause a rise in the amount of heat being generated, which leads to an increase of temperature in the combustion chamber and an increase in NOx released. With 50, 100, and 150 ppm of nano-Al2O3, NOx emissions were increased by 2.65%, 4.39%, and 5.37%, respectively.
Figure 6: Effect of the mass fractions of added nanoparticles emitted NOx levels at variable engine loads
The high levels of sulfur present in Iraqi-origin fuel, both diesel and gasoline, makes it one of the worst types of fuel in the world [35]. This sulfur is sourced from Iraqi crude oil, as well as old and inefficient Iraqi refineries [36,37]. When nano-Al2O3 is added to diesel, it increases the sulfur dioxide levels released from the engine [38]. The SO2 levels are higher when the engine is running at low and high loads, and lower when it is running at medium loads. This is because the combustion heat is higher at medium loads, which leads to a better oxidation of sulfur and a reduction in the formation of aromatic compounds and PM. The addition of nanoparticles to diesel in concentrations of 50, 100, and 150 ppm resulted in an increase in SO2 levels of 4.57%, 8.8%, and 12.89%, respectively, compared to regular diesel, as shown in Fig. 7. This is at the expense of a decrease in TSP levels.
Figure 7: Effect of the mass fractions of added nanoparticles on emitted SO2 levels at variable engine loads
Humans should not be exposed to hydrogen sulfide for more than four hours at a concentration of 100 ppm or higher, as this could cause an immediate loss of consciousness [39]. Fig. 8 shows that when the engine runs on nano-Al2O3-diesel blends, the level of H2S emissions increases as BSFC increases. Specifically, when the engine runs on 50, 100, and 150 ppm nano-Al2O3-diesel blends, the levels of H2S emitted increase by 4.7%, 7.81%, and 8.9%, respectively, as compared to diesel [40]. Although the SO2 and H2S levels have not reached a dangerous level, they can accumulate in enclosed spaces such as tunnels and garages, which can be hazardous to people [41]. The increase in sulfur in the fuel leads to an increase in total suspended particles (TSP), which is an accumulation of carbon generated from the combustion [40,41].
Figure 8: Effect of the mass fractions of added nanoparticles on emitted H2S levels at variable engine loads
When oxygen is present in the area of the combustion chamber (as it is with diesel engines), and the fuel has a high thermal conductivity which improves its vaporization and blending with air, the oxidation process is improved, and the levels of PM are reduced [42]. As Fig. 9 shows, when nano-Al2O3 is added to diesel, the TSP lessens with the added mass fractions of the nanoparticle. The presence of extra oxygen in the combustion chamber oxidizes the carbon and sulfur atoms, which in turn decreases the total suspended particulate matter. In comparison to diesel, the TSP decreased by 22%, 43.66%, and 40.7% when nano-Al2O3 was added in the amounts of 50, 100, and 150 ppm, respectively. These results are consistent with references [43,44] findings.
Figure 9: Effect of the mass fractions of added nanoparticles on emitted TSP levels at variable engine loads
The trade-off concept means giving up one advantage for the sake of another and expresses the trade-off between bias and variance; it is a balance between having a bias that is too high or too complex (high variance). This relationship strives to find a balance between the two, resulting in accurate and generalizable results. Fig. 10 illustrates the complicated dilemma of the NOx and PM trade-off; any action taken to reduce NOx causes higher PM, and vice versa. This trade-off is significant as it reveals whether the fuel used leads to a rise or decrease in both pollutants. The graph shows that in comparison to diesel, the studied suspensions led to a considerable decrease in PM and only a minor reduction in NOx. These results agree with references [45,46].
Figure 10: NOx-PM trade-off for the mass fractions of the added nanoparticles
This study examined the effects of adding nano-alumina to Iraqi diesel, which is a poor fuel due to its high sulfur content. The engine was rotated at a constant speed (1500 rpm) and changing applied loads were tested to evaluate the performance and emissions of the mixtures. The physicochemical properties of the mixtures were studied, which showed an increase in thermal conductivity with a limited increase in density and viscosity. Additionally, measurements of the suspensions’ stability showed that the tested samples were highly stable (more than three months).
Fuel consumption decreased by 0.488%, 1.02%, and 1.377% when adding 50, 100, and 150 nano-alumina, respectively. The thermal efficiency increased the BTE by 1.4%, 2.6%, and 3.8% as a direct result of the improved thermal conductivity of the fuel. This addition decreased the CO levels by 5.88%, 11.7%, and 17.6%, and the HC concentrations decreased by a limited extent of 1.5%, 1.7%, and 2.8%, respectively. NOx levels increased by 2.65%, 4.36%, and 5.37% for the same addition percentages, respectively. TSP decreased by 22%, 34.66%, 49.7% for the same addition percentages, respectively. Sulfur dioxide pollutants increased by 4.57%, 8.8%, and 12.89%, and H2S levels increased by 4.7%, 7.81%, and 8.9% for the same percentages, respectively. The results showed that when the suspensions were compared to diesel, there was a significant decrease in PM and only a slight increase in NOx.
Future experiments on diesel-biodiesel mixtures containing aluminum oxide nanoparticles are very important. Sulfur content of the final mixture will be reduced when high sulfur diesel is mixed with sulfur free biodiesel. It will be possible to create high-performance fuel with low emissions by adding nanoparticles to fuel.
Funding Statement: The authors received no specific funding for this study.
Conflicts of Interest: The authors declare that they have no conflicts of interest to report regarding the present study.
References
1. Pandey, S. (2022). A critical review: Application of methanol as a fuel for internal combustion engines and effects of blending methanol with diesel/biodiesel/ethanol on performance, emission, and combustion characteristics of engines. Heat Transfer, 51(4), 3334–3352. https://doi.org/10.1002/htj.22453 [Google Scholar] [CrossRef]
2. Chaichan, M. T. (2018). Combustion and emission characteristics of E85 and diesel blend in conventional diesel engine operating in PPCI mode. Thermal Science and Engineering Progress, 7(12), 45–53. https://doi.org/10.1016/j.tsep.2018.04.013 [Google Scholar] [CrossRef]
3. Dhahad, H. A., Chaichan, M. T., Megaritis, T. (2019). Performance, regulated and unregulated exhaust emission of a stationary compression ignition engine fueled by water-ULSD emulsion. Energy, 181(10), 1036–1050. https://doi.org/10.1016/j.energy.2019.05.200 [Google Scholar] [CrossRef]
4. Bitire, S. O., Jen, T. C. (2022). The role of a novel green synthesized nanoparticles added parsley biodiesel blend on the performance-emission characteristics of a diesel engine. South African Journal of Chemical Engineering, 41(1), 161–175. https://doi.org/10.1016/j.sajce.2022.06.007 [Google Scholar] [CrossRef]
5. Ekaab, N. S., Hamza, N. H., Chaichan, M. T. (2019). Performance and emitted pollutants assessment of diesel engine fuelled with biokerosene. Case Studies in Thermal Engineering, 13(3), 100381. https://doi.org/10.1016/j.csite.2018.100381 [Google Scholar] [CrossRef]
6. Venkatesan, H., Sivamani, S., Sampath, S., Gopi, V., Kumar, D. (2017). A comprehensive review on the effect of nano metallic additives on fuel properties, engine performance and emission characteristics. International Journal of Renewable Energy Research-IJRER, 7(2), 825–843. [Google Scholar]
7. Khan, O., Khan, M. Z., Bhatt, B. K., Alam, M. T., Tripathi, M. (2022). Multi-objective optimization of diesel engine performance, vibration and emission parameters employing blends of biodiesel, hydrogen and cerium oxide nanoparticles with the aid of response surface methodology approach. International Journal of Hydrogen Energy, 10(95), 1. https://doi.org/10.1016/j.ijhydene.2022.04.044 [Google Scholar] [CrossRef]
8. Duan, X., Lai, M. C., Jansons, M., Guo, G., Liu, J. (2021). A review of controlling strategies of the ignition timing and combustion phase in homogeneous charge compression ignition (HCCI) engine. Fuel, 285, 119142. https://doi.org/10.1016/j.fuel.2020.119142 [Google Scholar] [CrossRef]
9. Liang, D., Wu, Z., Jiang, Y., Ren, K., Zhou, M. et al. (2022). Effects of solids’ concentration and oleic acid dispersant on the stability and combustion characteristics of aluminum/bioethanol nanofluid fuel. Powder Technology, 398(6), 117108. https://doi.org/10.1016/j.powtec.2022.117108 [Google Scholar] [CrossRef]
10. Lawrence, K. R., Huang, Z., Nguyen, X. P., Balasubramanian, D., Gangula, V. R. et al. (2022). Exploration over combined impacts of modified piston bowl geometry and tert-butyl hydroquinone additive-included biodiesel/diesel blend on diesel engine behaviors. Fuel, 322(2), 124206. https://doi.org/10.1016/j.fuel.2022.124206 [Google Scholar] [CrossRef]
11. Huang, Z., Huang, J., Luo, J., Hu, D., Yin, Z. (2022). Performance enhancement and emission reduction of a diesel engine fueled with different biodiesel-diesel blending fuel based on the multi-parameter optimization theory. Fuel, 314(3), 122753. https://doi.org/10.1016/j.fuel.2021.122753 [Google Scholar] [CrossRef]
12. Ranjan, A., Dawn, S. S., Jayaprabakar, J., Nirmala, N., Saikiran, K. et al. (2018). Experimental investigation on effect of MgO nanoparticles on cold flow properties, performance, emission and combustion characteristics of waste cooking oil biodiesel. Fuel, 220, 780–791. https://doi.org/10.1016/j.fuel.2018.02.057 [Google Scholar] [CrossRef]
13. Chaichan, M. T., Kadhum, A. A. H., Al-Amiery, A. A. (2017). Novel technique for enhancement of diesel fuel: Impact of aqueous alumina nano-fluid on engine’s performance and emissions. Case Studies in Thermal Engineering, 10(7), 611–620. https://doi.org/10.1016/j.csite.2017.11.006 [Google Scholar] [CrossRef]
14. Dhahad, H. A., Hasan, A. M., Chaichan, M. T., Kazem, H. A. (2022). Prognostic of diesel engine emissions and performance based on an intelligent technique for nanoparticle additives. Energy, 238(1), 121855. https://doi.org/10.1016/j.energy.2021.121855 [Google Scholar] [CrossRef]
15. Sathiamurthi, P., Vinith, K. K., Sivakumar, A. (2019). Performance and emission test in CI engine using magnetic fuel conditioning with nano additives. International Journal of Recent Technology and Engineering (IJRTE), 8(3), 7823–7826. https://doi.org/10.35940/ijrte.C6213.098319 [Google Scholar] [CrossRef]
16. Fayad, M. A., Abed, A. M., Omran, S. H., Jaber, A. A., Radhi, A. A. et al. (2022). Influence of renewable fuels and nanoparticles additives on engine performance and soot nanoparticles characteristics. International Journal of Renewable Energy Development, 11(4), 1068–1077. https://doi.org/10.14710/ijred.2022.45294 [Google Scholar] [CrossRef]
17. Alawi, O. A., Sidik, N. A. C., Xian, H. W., Kean, T. H., Kazi, S. N. (2018). Thermal conductivity and viscosity models of metallic oxides nanofluids. International Journal of Heat and Mass Transfer, 116, 1314–1325. https://doi.org/10.1016/j.ijheatmasstransfer.2017.09.133 [Google Scholar] [CrossRef]
18. Fayaz, H., Mujtaba, M. A., Soudagar, M. E. M., Razzaq, L., Nawaz, S. et al. (2021). Collective effect of ternary nano fuel blends on the diesel engine performance and emissions characteristics. Fuel, 293, 120420. https://doi.org/10.1016/j.fuel.2021.120420 [Google Scholar] [CrossRef]
19. Venkatesan, S. P., Kadiresh, P. N. (2015). Influence of aluminum oxide nanoparticle additive on performance and exhaust emissions of diesel engine. International Journal of Applied Engineering Research, 10(3), 5741–5749. [Google Scholar]
20. Nouri, M., Isfahani, A. H. M., Shirneshan, A. (2021). Effects of Fe2O3 and Al2O3 nanoparticle-diesel fuel blends on the combustion, performance and emission characteristics of a diesel engine. Clean Technologies and Environmental Policy, 23(8), 2265–2284. https://doi.org/10.1007/s10098-021-02134-8 [Google Scholar] [CrossRef]
21. Khan, O., Emran Khan, M., Parvez, M., Ahmed, K. A. A. R., Ahmad, I. (2022). Extraction and experimentation of biodiesel produced from leachate oils of landfills coupled with nano-additives aluminium oxide and copper oxide on diesel engine. In: Nanomaterials for innovative energy systems and devices, pp. 319–332. Singapore: Springer Nature Singapore. [Google Scholar]
22. Khan, O., Khan, M. Z., Khan, E., Bhatt, B. K., Afzal, A. et al. (2022). An enhancement in diesel engine performance, combustion, and emission attributes fueled with Eichhornia crassipes oil and copper oxide nanoparticles at different injection pressures. Energy Sources, Part A: Recovery, Utilization, and Environmental Effects, 44(3), 6501–6522. https://doi.org/10.1080/15567036.2022.2100014 [Google Scholar] [CrossRef]
23. Chen, A. F., Adzmi, M. A., Adam, A., Othman, M. F., Kamaruzzaman, M. K. et al. (2018). Combustion characteristics, engine performances and emissions of a diesel engine using nanoparticle-diesel fuel blends with aluminum oxide, carbon nanotubes and silicon oxide. Energy Conversion and Management, 171(2), 461–477. https://doi.org/10.1016/j.enconman.2018.06.004 [Google Scholar] [CrossRef]
24. Dhahad, H. A., Chaichan, M. T. (2020). The impact of adding nano-Al2O3 and nano-ZnO to Iraqi diesel fuel in terms of compression ignition engines’ performance and emitted pollutants. Thermal Science and Engineering Progress, 18(2), 100535. https://doi.org/10.1016/j.tsep.2020.100535 [Google Scholar] [CrossRef]
25. Adzmi, M. A., Abdullah, A., Abdullah, Z., Mrwan, A. G., Effect of Al2O3 and SiO2 metal oxide nanoparticles blended with POME on combustion, performance and emissions characteristics of a diesel engine. International Journal of Automotive and Mechanical Engineering, 16(3), 6859–6873. [Google Scholar]
26. Channappagoudra, M. (2021). Influence of the aluminum oxide (Al2O3) nanoparticle additive with biodiesel on the modified diesel engine performance. International Journal of Ambient Energy, 42(15), 1776–1784. https://doi.org/10.1080/01430750.2019.1614992 [Google Scholar] [CrossRef]
27. Fayad, M. A., Chaichan, M. T., Dhahad, H. A., Al-Amiery, A. A., Wan Isahak, W. N. R. (2022). Reducing the effect of high sulfur content in diesel fuel on NOx emissions and PM characteristics using a PPCI mode engine and gasoline-diesel blends. ACS Omega, 7(42), 37328–37339. https://doi.org/10.1021/acsomega.2c03878 [Google Scholar] [PubMed] [CrossRef]
28. Al-Waeli, A. H., Sopian, K., Kazem, H. A., Chaichan, M. T. (2020). Evaluation of the electrical performance of a photovoltaic thermal system using nano-enhanced paraffin and nanofluids. Case Studies in Thermal Engineering, 21(6), 100678. https://doi.org/10.1016/j.csite.2020.100678 [Google Scholar] [CrossRef]
29. Jawad, Q. A., Mahdy, A. M., Khuder, A. H., Chaichan, M. T. (2020). Improve the performance of a solar air heater by adding aluminum chip, paraffin wax, and nano-SiC. Case Studies in Thermal Engineering, 19(3), 100622. https://doi.org/10.1016/j.csite.2020.100622 [Google Scholar] [CrossRef]
30. Dhahad, H. A., Ali, S. A., Chaichan, M. T. (2020). Combustion analysis and performance characteristics of compression ignition engines with diesel fuel supplemented with nano-TiO2 and nano-Al2O3. Case Studies in Thermal Engineering, 20(5), 100651. https://doi.org/10.1016/j.csite.2020.100651 [Google Scholar] [CrossRef]
31. Hamadi, A. S., Khidhir, A. G. (2019). Environmental pollution control emitted from diesel engines by Al2O3 nanoparticles. IOP Conference Series: Materials Science and Engineering, 579(1), 012013. https://doi.org/10.1088/1757-899X/579/1/012013 [Google Scholar] [CrossRef]
32. Venu, H., Madhavan, V. (2017). Effect of diethyl ether and Al2O3 nano additives in diesel-biodiesel-ethanol blends: Performance, combustion and emission characteristics. Journal of Mechanical Science and Technology, 31(1), 409–420. https://doi.org/10.1007/s12206-016-1243-x [Google Scholar] [CrossRef]
33. Khan, O., Khan, M. Z., Ahmad, N., Qamer, A., Alam, M. T. et al. (2021). Performance and emission analysis on palm oil derived biodiesel coupled with Aluminum oxide nanoparticles. Materials Today: Proceedings, 46(1), 6781–6786. https://doi.org/10.1016/j.susoc.2022.01.006 [Google Scholar] [CrossRef]
34. Khan, O., Yadav, A. K., Khan, M. E., Parvez, M. (2021). Characterization of bioethanol obtained from Eichhornia Crassipes plant; its emission and performance analysis on CI engine. Energy Sources, Part A: Recovery, Utilization, and Environmental Effects, 43(14), 1793–1803. https://doi.org/10.1080/15567036.2019.1648600 [Google Scholar] [CrossRef]
35. Kadhem, J. A., Reza, K. S., Ahmed, W. K. (2017). Alternative fuel use in Iraq: A way to reduce air pollution. European Journal of Engineering and Technology Research, 2(5), 20–30. [Google Scholar]
36. Al-sareji, O. J., Grmasha, R. A., Hashim, K. S., Salman, J. M., Al-Juboori, R. A. (2022). Personal exposure and inhalation doses to PM1 and PM2.5 pollutions in Iraq: An examination of four transport modes. Building and Environment, 212, 108847. https://doi.org/10.1016/j.buildenv.2022.108847 [Google Scholar] [CrossRef]
37. Asmel, N. K., Muhammed, F. I., Hassan, S. I., Ganiyu, A. A., Lakkaboyana, S. K. (2022). Assessment of ambient air quality in urban places of Mosul City, Iraq. International Journal of Environmental Science and Technology, 20, 1–18. https://doi.org/10.1007/s13762-022-04197-6 [Google Scholar] [CrossRef]
38. Smith, M., Filipi, Z., Schihl, P., Assanis, D. (2010). Effect of high sulfur military JP-8 fuel on heavy duty diesel engine emissions and EGR cooler condensate. Internal Combustion Engine Division Fall Technical Conference, ICEF2010-35001, pp. 99–110. https://doi.org/10.1115/ICEF2010-35001 [Google Scholar] [CrossRef]
39. Di, Y. G., Zhang, J. J., Cheung, C. S., Miao, X. L., Zheng, J. B. et al. (2022). Comparative study on combustion and particulate emissions for diesel-biodiesel and diesel-diglyme blends. Fuel, 313(1), 122710. https://doi.org/10.1016/j.fuel.2021.122710 [Google Scholar] [CrossRef]
40. Oguntoke, O., Yussuf, A. S. (2010). Air pollution arising from vehicular emissions and the associated human health problems in abeokuta metropolis, Nigeria. ASSET: An International Journal (Series A), 8(2), 119–132. [Google Scholar]
41. Dhahad, H. A., Fayad, M. A., Chaichan, M. T., Jaber, A. A., Megaritis, T. (2021). Influence of fuel injection timing strategies on performance, combustion, emissions and particulate matter characteristics fueled with rapeseed methyl ester in modern diesel engine. Fuel, 306(5), 121589. https://doi.org/10.1016/j.fuel.2021.121589 [Google Scholar] [CrossRef]
42. Hamza, N. H., Ekaab, N. S., Chaichan, M. T. (2020). Impact of using Iraqi biofuel-kerosene blends on coarse and fine particulate matter emitted from compression ignition engines. Alexandria Engineering Journal, 59(3), 1717–1724. https://doi.org/10.1016/j.aej.2020.04.031 [Google Scholar] [CrossRef]
43. Dhahad, H. A., Chaichan, M. T. (2023). The effect of load and injection timing on the emitted pollutants concentrations by engine fuelled by biofuel. Proceedings of the 7th International Conference on Production, Energy and Reliability, pp. 295–302. Singapore: Springer. [Google Scholar]
44. Zhu, Y., Zhou, W., Xia, C., Hou, Q. (2022). Application and development of selective catalytic reduction technology for marine low-speed diesel engine: Trade-off among high sulfur fuel, high thermal efficiency, and low pollution emission. Atmosphere, 13(5), 731. [Google Scholar]
45. Al Ezzi, A., Fayad, M. A., Al Jubori, A. M., Jaber, A. A., Alsadawi, L. A. et al. (2022). Influence of fuel injection pressure and RME on combustion, NOx emissions and soot nanoparticles characteristics in common-rail HSDI diesel engine. International Journal of Thermofluids, 15(5), 100173. https://doi.org/10.1016/j.ijft.2022.100173 [Google Scholar] [CrossRef]
46. Fayad, M. A., Ibrahim, S. I., Omran, S. H., Martos, F. J., Badawy, T. et al. (2023). Experimental effect of CuO2 nanoparticles into the RME and EGR rates on NOX and morphological characteristics of soot nanoparticles. Fuel, 331(1), 125549. https://doi.org/10.1016/j.fuel.2022.125549 [Google Scholar] [CrossRef]
Cite This Article
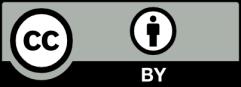
This work is licensed under a Creative Commons Attribution 4.0 International License , which permits unrestricted use, distribution, and reproduction in any medium, provided the original work is properly cited.