Open Access
ARTICLE
Market Drivers in India’s Smart Grid: Responsibilities and Roles of Stakeholders
1 Faculty of Management Studies, Sri Sri University, Cuttack, 754006, India
2 Institute of Power Engineering, National Energy University, Jalan Ikram-Uniten, Kajang, Selangor, 43000, Malaysia
3 Centre of Research Impact and Outcome, Chitkara University, Rajpura, 140417, Punjab, India
4 Department of Applied Mechanical Engineering, College of Applied Engineering, Muzahimiyah Branch, King Saud University, Riyadh, 11421, Saudi Arabia
5 College of Engineering, Lishui University, Lishui, 323000, China
6 Department of Mechanical Engineering, Graphic Era (Deemed to be University), Dehradun, 248002, Uttarakhand, India
7 Department of Mechanical Engineering, Faculty of Engineering, University of Malaya, Kuala Lumpur, 50603, Malaysia
8 Department of Mechanical Engineering, Faculty of Engineering and Architecture, Recep Tayyip Erdogan University, Zihni Derin Campus, Rize, 53100, Turkey
9 Center for Research Impact & Outcome, Chitkara University, Rajpura, 140401, Punjab, India
10 University Centre for Research and Development, Chandigarh University, Mohali, 140413, Punjab, India
* Corresponding Author: Erdem Cuce. Email:
Energy Engineering 2025, 122(1), 101-128. https://doi.org/10.32604/ee.2024.055105
Received 17 June 2024; Accepted 21 October 2024; Issue published 27 December 2024
Abstract
The emergence of smart grids in India is propelled by an intricate interaction of market dynamics, regulatory structures, and stakeholder obligations. This study analyzes the primary factors that are driving the widespread use of smart grid technologies and outlines the specific roles and obligations of different stakeholders, such as government entities, utility companies, technology suppliers, and consumers. Government activities and regulations are crucial in facilitating the implementation of smart grid technology by offering financial incentives, regulatory assistance, and strategic guidance. Utility firms have the responsibility of implementing and integrating smart grid infrastructure, with an emphasis on improving the dependability of the grid, minimizing losses in transmission and distribution, and integrating renewable energy sources. Technology companies offer the essential hardware and software solutions, which stimulate creativity and enhance efficiency. Consumers actively engage in the energy ecosystem by participating in demand response, implementing energy saving measures, and adopting distributed energy resources like solar panels and electric vehicles. This study examines the difficulties and possibilities in India's smart grid industry, highlighting the importance of cooperation among stakeholders to build a strong, effective, and environmentally friendly energy future.Keywords
Nomenclature
SG | Smart grid |
DG | Distributed generation |
ICT | Information and Comm. Technology |
EV | Electric vehicle |
DISCOMs | Distributed Company |
PGCIL | Power Grid Corp. of India Ltd. |
MNRE | Ministry of new and renewable energy |
SGT | Smart grid technology |
GSFG | Global smart grid federation |
MOP | Ministry of power |
SGCC | Smart grid consumer collaborative |
DSM | Demand side management |
EV | Electric vehicle |
NPV | Net present value |
PMU | Phasor measurement value |
PSC | Public sector comparator |
RTP | Real time pricing |
TSO | Transmission system operator |
IEM | Internal energy market |
PHEV | Plug in electric vehicle |
PPP | Public private partnership |
WAMS | Wide area monitoring system |
NGO | Non-government organization |
BEE | Bureau of energy efficiency |
NEF | National electricity fund |
APERC | Andhra Pradesh Electricity Regulatory Commission |
MG | Micro grid |
DR | Demand response |
GOI | Government of India |
GPS | Global positioning syst |
OA | Open access |
RTS | Rooftop solar |
PLCC | Power line carrier communication |
AMI | Advanced metering technology |
CPP | Critical peak pricing |
GOI | Govt of India |
CEA | Central electricity authority |
ToU | Time of use |
PPA | Power purchase agreement |
SERC | State Electricity Regulatory Commission |
SIPS | System integrated protection scheme |
STU | State transmission utility |
OMS | Outage Management System |
AMI | Advanced Metering Infrastructure |
PLMS | Peak Load Management System |
RE | Renewable Energy |
DRUM | Distribution Reform, Upgrades, and Management |
HEMS | Home Energy Management System |
DISCOM | Distribution Company |
R-APD&RP | Restructured Accelerated Power development and Reforms Program |
The stakeholders in the smart energy and smart grid (SG) [1] business are identified and their respective aims and tasks are defined. The discourse revolves around the regulatory framework, including both current and potential future regulatory alterations. The text outlines the framework for examining company and service models, and introduces the concepts of value chain, value network, and value creation principles. These studies encompass the depiction of potential businesses and services, principles for capturing value, principles for investment and pricing, and an examination of potential advantages and hazards linked to new businesses and services.
The vision for a ‘SG Vision for India’ aims to transform the power landscape in the country into a secure, adaptable, sustainable, and digitally empowered environment. This vision strives to provide reliable and high-quality power to all, aligning with the dynamic needs of stakeholders. Recognizing the increasing significance of SG technologies in the Indian energy sector [2], the India Government, based on recommendations from the India SG Task Force, has identified fourteen SG pilot projects for implementation in the power distribution sector.
In accordance with the ‘SG Roadmap for India,’ these pilot initiatives are anticipated to serve as technology test beds, establishing business cases, formulating policies, and offering regulatory recommendations for larger-scale projects in subsequent phases. Simultaneously, these pilots aim to showcase the applicability of SG across various aspects, including Advanced Metering Infrastructure (AMI), Peak Load Management System (PLMS), Outage Management System (OMS), RE Integration, and more [3].
The anticipated cost for these pilot projects is approximately around US$ 10 million. Fifty percent of this funding is expected to be provided by the Indian Government through the Restructured Accelerated Power development and Reforms Program (R-APD&RP). The remaining funds will be managed either by the utility undertaking the project or shared between the utility and its technology providers. This initiative underscores the commitment to leveraging SG technologies to enhance the efficiency, reliability, and sustainability of India’s power distribution infrastructure. Evaluating a country’s power system security requires assessing its capacity to endure contingency events without causing disruptions to normal operational conditions. Contingency occurrences are commonly defined by the magnitude of power flow on each transmission line and the voltage level at each electrical bus. An effective method for assessing the influence of a contingency event on a power system is to replicate the scenario using a power flow analysis. Due to the extensive amount of contingency events that need to be analyzed, it is not feasible to model each case in depth utilizing a complete AC power flow analysis. The commonly embraced method entails tackling the problem through two distinct stages: contingency selection and contingency analysis. Contingency selection involves the use of a quick screening technique to determine the most crucial contingency occurrences or to prioritize all instances according to their severity. The distribution factors technique is a frequently employed method for doing contingency screening. Real-time contingency ranking often relies on distribution variables for their quick assessment and storage, enabling speedy analysis. Contingency analysis is then conducted on the possibly important cases.
The advancement of intelligent power distribution networks in India is propelled by an intricate interaction of market dynamics, regulatory structures, and stakeholder obligations. This article analyzes the primary factors that are driving the widespread use of smart grid technologies and outlines the specific roles and duties of different stakeholders, such as government entities, utility corporations, technology suppliers, and consumers. Energy Storage Systems (ESS) are essential in the smart grid environment as they play a crucial role in maintaining a balance between the supply and demand of energy, improving the stability of the grid, and enabling the seamless integration of renewable energy sources [1,4]. Energy Storage Systems (ESS) mitigate variations in energy supply by storing surplus energy produced during periods of low demand and subsequently releasing it during peak periods. This process reduces dependence on fossil fuel-powered peaking power plants and enhances the overall efficiency of the grid. This study examines the difficulties and possibilities in India’s smart grid industry, highlighting the importance of cooperation among stakeholders to build a strong, efficient, and environmentally friendly energy future [5].
Contemporary literature on the energy market in India emphasizes various significant changes and advancements that are influencing the industry [6,7]. The prevailing focus is on the incorporation of renewable energy sources, particularly solar and wind power, into the energy composition. Research highlights the growing proportion of these sources in the energy mix, as well as the difficulties related to integrating them into the power system and ensuring its stability. Scientists have investigated how regulatory reforms might help facilitate market transitions, specifically examining the effects of legislation like the Electricity (Amendment) Bill 2020 [8]. This bill is designed to improve competition and give consumers more options. Another area of focus is the implementation of sophisticated metering infrastructure and smart grid technologies, which have been studied for their potential to enhance energy efficiency, minimize losses, and enable users to regulate their energy usage in real-time. Furthermore, there is an increasing amount of research on the economic feasibility and long-term viability of distribution businesses (DISCOMs), examining elements such as pricing models, government financial assistance, and the execution of UDAY (Ujwal DISCOM Assurance Yojana) reforms. Research also emphasizes the significance of energy storage systems and demand response programs in maximizing energy utilization and incorporating intermittent renewable sources [9,10]. In general, contemporary research emphasizes the complex and diverse characteristics of India’s power industry [11], which are influenced by improvements in technology, changes in regulations, and the pressing want for sustainable and dependable energy solutions.
This research presents a comprehensive approach that examines the interaction among various energy stakeholders [12]. Further it discusses advancements in energy consumption, RE sources, crowd energy, and SGTs, along with the benefits and challenges connected with their implementation. The paper analyzes the complex system, including both the positive and negative impact of the stakeholders. The framework [13] is mostly presented in the context of India, although it is also applicable to other locations. A communication plan include public awareness campaigns, social advertising, and customer education to actively advocate for the use of SGTs.
As per the Ministry of Power (MOP), India experiences one of the highest rates of transmission and distribution losses globally, with an average of 26 percent of total power generation and reaching as high as 62 percent in specific locations. These calamities specifically exclude non-technical losses like theft. If these losses are taken into account, the average losses can reach up to 50 percent. India experiences financial losses for each unit of power sold because to its very vulnerable electrical infrastructure, which is considered one of the most delicate in the world. Certain deficiencies in the Indian electricity grid include a poorly designed distribution network, excessive load on the system components, inadequate reactive power support and regulation services, low efficiency in metering, and poor bill collection.
Several crucial factors are propelling the growth of the SG industry (Fig. 1), which is reshaping the future of the electrical sector. One of the main factors behind this is the growing necessity to update and enhance the efficiency and dependability of outdated grid infrastructure. SGTs provide the real-time monitoring, control, and optimization of electricity flow, resulting in enhanced energy distribution efficiency and decreased wastage. Another factor contributing to this trend is the increasing incorporation of RE sources, such as solar and wind, into the power system. SGs aid in the management of the variability and unpredictability of these energy sources, guaranteeing a steady and dependable power provision. Moreover, the increasing requirement for electricity, particularly in emerging nations, is propelling the necessity for more effective and environmentally friendly energy alternatives, thereby intensifying the expansion of the SG industry. Regulatory assistance and government efforts play a crucial role in promoting the adoption of SGs. They offer incentives and cash to utilities and grid operators, encouraging them to invest in SGTs. In general, these factors are promoting the use of SGT on a global scale, revolutionizing the power industry and preparing the path for a more environmentally friendly and effective energy future.
Figure 1: Factors for successful SG industry
By integrating SGTs with RE will result in economic benefits, such as improved reliability and reduced emissions, leading to better public health [14]. Additionally, there will be long-term gains in both the economy and the environment due to the use of low-carbon electricity [15]. The adoption of RE sources reduces a nation’s reliance on imported fossil fuels, resulting in a substantial decrease in import quantities. This, in turn, enhances a country’s energy security and self-sufficiency. Additionally, the placement of the SGTs offers the potential for more efficient energy utilization within a country [16]. This is particularly crucial considering recent developments where oil prices have been utilized as a political tool, leading to instability. The integration of RE with SGT presents a compelling case for establishing a dependable network in the event of potential grid outages. Customers can lower their energy expenses by implementing sophisticated energy management techniques [17], such as consuming energy during non-peak hours, utilizing energy-efficient equipment, selling surplus generated energy, and opting for low-carbon energy sources.
2.1 Challenges in the Deployment of SGTs
The collaboration and coordination among various stakeholders (such as end-users, suppliers, and providers) is intricate due to the ongoing advancements in technology (Fig. 2). Comprehending and conveying the worth of deploying SGTs to each participant in the electrical supply chain can be challenging due to the substantial upfront expenses involved, coupled with the unpredictable outcomes it offers [18]. This is further exacerbated by the lack of an appropriate incentive system that aligns economic and regulatory policies with environmental objectives and encourages the efficient use of energy. The recognition of the positive effects of deploying SGTs, such as the generation of new employment opportunities and the promotion of highly skilled workers, is currently lacking [19]. Simultaneously, the absence of expertise, along with uncertainty over cost and performance, as well as non-technical concerns like data protection, provide further obstacles in effectively utilizing technologies [20].
Figure 2: Different challenges of SG
2.2 Indian Government Initiatives Towards a Smart Grid
In 2010, the GOI established the India SG Task Force as a cross-departmental team that is going to function as the central coordinating body for the government. The collaboration consists of professionals from multiple government agencies and is largely focused on comprehending and advocating for regulations related to SGTs [21,22].
The primary functions of the India SG Task Force are guaranteeing cognizance, synchronization, as well as amalgamation of varied operations pertaining to SGTs. Additionally, it supports the implementation of methods and services for the advancement of intelligent power grids, facilitates and integrates other pertinent activities amongst governments, cooperates on a framework for compatibility and connectivity, and evaluates and endorses suggestions from the India Smart Grid Forum [23].
2.2.1 India Smart Grid Forum (ISGF)
ISGF, established in 2010, is described by the GOI as a voluntary alliance of public and commercial participants operating on a not-for-profit framework within the primary objective of expediting the development of SGTs in the Indian energy sector [24]. ISGF has essential roles and responsibilities inside the organization.
The primary goal of the ISGF is to facilitate the implementation of Intelligent Grid technologies in India’s power sector in an effective, economical, inventive, and adaptive method [25]. This is achieved by bringing together all key stakeholders and promoting the adoption of cutting-edge technologies [26].
The ISGF will facilitate and assist prominent international and Indian organizations in adopting global best practices whenever feasible or beneficial and will highlight any deficiencies in these practices from an Indian perspective (Fig. 3).
Figure 3: Information flow and hierarchy diagram of the India SG task force and the ISGF
2.2.2 Distribution Reform, Upgrades, and Management (DRUM)
Contingencies that lead to power system failures are significant difficulties in power system operation [27]. While these occurrences are rare, they have the potential to escalate into widespread blackouts. Shoppers often have a limited level of understanding regarding the transmission of power to their houses. Prior to implementing SG concepts, it is important to educate consumers about the definition of a SG, its contribution to a low carbon economy [28], and the advantages that customers can derive from the SGs. Customers should be informed about the energy consumption patterns in their homes and workplaces. Policy makers and regulators should have a clear understanding of the future potential of SGs [29]. Utilities should give greater importance to the complete functionality and capacities of SGs, rather than solely focusing on the installation of smart meters.
The main objective of the DRUM Project is to observe economically feasible power delivery networks which deliver dependable power of adequate quality to users. In addition, the project’s objective is to create a business network and a replicable procedure supported by India’s financial institutions to offer non-recourse finance for DRUM activities and projects.
2.3 Re-Structured Accelerated Power Development and Reforms Program
The MOP, under the GOI, has initiated the R-APD&RP as part of its efforts to reform the power sector. This program has been launched within the framework of the five-year plan [30,31]. The initiative principally aims to decrease AT&C losses, implement dependable and automated sustainable methods for gathering standardized data, incorporate information technology in power accounting and consumer service, and enhances the delivery network of state power utilities. The plan will be executed in two phases. Part-A will include the efforts to build standardized data and IT solutions for energy bookkeeping/assessment, as well as IT-based customer support centres. Part-B will consist of traditional workouts designed to enhance blood flow.
The power-system security of a country is evaluated by its capacity to withstand potential incidents without causing harm to normal operations [32]. The parameters to be evaluated are typically the magnitude of the current flow through each branch as well as the voltage level on each transmission line. An effective method to evaluate the influence of a hypothetical circumstance on a power system is to model the scenario using a power flow analysis [33]. Due to the vast number of potential scenarios that need to be analysed, it is not practical to replicate each situation in its entirety using a comprehensive AC configuration. The widely recognized approach involves addressing the problem through two distinct stages: possibility selection and possibility analysis. During the process of possibility determination, a rapid screening technique is utilized to select the most extreme potential scenarios or to prioritize all the scenarios based on their levels of severity. During the process of possibility examination, specific power flow analyses are only conducted on the potential fundamental scenarios. Possibility screening is conducted using a distribution factors-based approach.
Transportation variables are commonly used consistently for placement due to the rapidity with which this assessment may be conducted after these factors have been evaluated as well as stored. Following this is a subsequent analysis, conducted on its potentially crucial instances. Static security is a fundamental component of power system security. Static security refers to the network’s ability to return to a specified secure state after a scenario. To address the security assessment issue, it is customary to first do a static security analysis, followed by a comprehensive security review. The static security evaluation evaluates the steady state condition of the system, disregarding transient behaviour and other time-dependent variations caused by changes in load generation conditions. On the other hand, the dynamic security assessment evaluates the temporal transition from the precontingent state to the post unanticipated state. Most Energy Management Systems mostly conduct static security analysis.
2.4 Smart Grid and Integration of Renewable Energy Sources
Sustainable power source assets vary significantly in terms of kind and flexibility. They employ biomass, waste, geothermal, hydro, sun, and wind as energy sources. Utilizing sustainable power source assets for autonomous or isolated power generation is beneficial, but their advantages are further amplified when they are incorporated into larger electric power grids [34]. By increasing the use of SGTs, it is possible to accommodate higher levels and rates of penetration [35]. Each item varies in its perspective from the grid, and some are more easily integrated than others [8,36]. The utilization of variable age, sourced from diverse RE sources, might present a difficulty to the operations of the electric grid. However, when integrated with intelligent grid strategies, responsive distributed generation can also provide benefits for grid operations by alleviating strain on the system [37,38].
As per the MOP, India experiences significant transmission and distribution losses, which are among the greatest globally. On average, these losses account for 26 percent of the total power production, and in certain places, they can reach as high as 62 percent. These calamities specifically exclude non-technical losses like theft. If these losses are taken into account, the average losses can reach up to 50 percent. India experiences financial losses for each unit of electricity sold due to its very vulnerable power infrastructure, which is considered one of the most frail in the world.
Few of the specific flaws in the Indian power grid include a poorly designed distribution network, excessive load on the system components, a lack of reactive power support and regulation services, low metering efficiency, and poor bill collection [39,40].
India is rapidly embracing RE sources [41] such as wind and solar electricity. India’s abundant solar potential is evident from its annual average of 300 sunny days, making it a highly promising source of energy. The legislature is offering subsidies for several solar applications as a means of encouraging solar power generation. In addition, they have established a goal for solar energy to account for 7 percent of India’s overall electricity generation by 2022. Given India’s lofty aspirations, solar energy will have a pivotal role in moulding the future of the country’s electricity sector.
An inherent limitation of sustainable assets is their intermittent availability. For instance, solar energy can only be used during daylight hours, whereas wind energy requires precise windy conditions. Furthermore, these variables are beyond human control. Due to the use of non-traditional energy sources for the grid, it is essential to develop a grid that can easily adjust to changes in market demand. India has significant potential for the development of SGs, as a dependable electricity supply is an essential infrastructural need for comprehensive progress [42].
Electric vehicles (EVs) and heat pumps greatly improve the efficient energy management of smart grids by offering essential demand-side flexibility and facilitating the incorporation of renewable energy sources [43]. Electric vehicles (EVs), with Vehicle-to-Grid (V2G) technology, have the capability to release stored energy back into the power grid when demand is highest [44]. This allows them to function as portable energy storage devices and contribute to the stability of the grid [45]. In addition, they engage in demand response initiatives by modifying their charging schedules in response to grid signals, thereby diminishing peak load and achieving equilibrium between supply and demand. Heat pumps also help by adjusting their operation to times when electricity demand is low or renewable energy output is high, thereby decreasing the strain on the power grid during peak load periods. When combined with thermal storage, heat pumps have the ability to store surplus heat or cold. This stored energy can then be utilized during times of high demand to reduce the strain on the power system. Electric vehicles (EVs) and heat pumps have the capability to offer ancillary services, such as frequency regulation and voltage support, which contribute to grid stability and enhance power quality [46]. Collectively, these technologies enable a more robust, effective, and environmentally-friendly energy system [47].
2.5 General View of the Smart Grid Market Drivers
To improve the efficiency of the power system and increase productivity and reliability, it is necessary for the SG to integrate digital technologies further (Table 1). This is supported by several market drivers and upcoming opportunities [48]. In the restructured state, the electric utility industry has been deregulated, allowing for the creation of a market that operates within regulatory boundaries and responds to the regular and daily changes in demand [49]. Profound financial markets:
1. Augment the transmission of electricity between the regions, that exacerbate the current aging power network and necessitate updated, real-time regulations.
2. Address the issue of grid congestion, enhance client collaboration, as well as reduce investment risk. This necessitates enhancing the grid’s capacity to handle requests reliably.
3. Efficiently incorporate RE systems and distributed generation. The substantial rise in the incorporation of cost-competitive distributed generating technologies has a direct effect on the power system. Stakeholders, along with framework administrators and policy-makers, are actively participating in the advancement of the SG [50]. Their specific commitments and theoretical understanding of the views to be adopted have been discussed below.
3 Stakeholders and Regulatory Environment
Stakeholders in the power supply chain encompass several entities, including government agencies, regulatory bodies, utilities, energy providers, technology vendors, customers, and environmental organizations [51,52]. Every stakeholder has a distinct function in influencing the energy industry, where government agencies and regulatory bodies establish rules, laws, and standards to guarantee the dependability, affordability, and sustainability of electricity provision [53]. Utilities and energy suppliers have the responsibility of producing, transmitting, and distributing electricity, while technology vendors supply the essential tools and solutions for updating the grid and enhancing efficiency. Consumers, including both residential and industrial users, have a significant impact on the demand patterns and behaviour of energy use [54,55]. Environmental organizations promote the adoption of sustainable practices and the integration of RE. Efficient cooperation and involvement among these parties are crucial for guaranteeing a dependable, durable, and environmentally-friendly power distribution network.
3.1.1 Traditional Electricity Stakeholders
The traditional players in the electrical industry consist of generation corporations, transmission system operators, distribution utilities, and regulatory organizations [56]. Generation businesses have the responsibility of creating power using a variety of sources, including coal, natural gas, hydro, and RE sources. Transmission system operators oversee the high-voltage transmission network to guarantee the dependable and effective transportation of electricity across large distances [57]. Distribution utilities have the responsibility of supplying power to end consumers, maintaining the low-voltage distribution network, and managing billing and customer support. Regulatory agencies supervise the electrical sector, establishing prices, laws, and standards to guarantee equitable competition, dependable service, and consumer safeguarding. These stakeholders have been crucial in the power industry for many years. However, with the introduction of SGT and the changing energy landscape, their responsibilities are adapting to address the future problems and opportunities.
The power supply chain can be categorized into multiple essential stages (Sanderson). The process commences with primary fuel sources, encompassing coal, natural gas, nuclear, hydropower, and RE sources like wind and solar. These principal fuel sources are utilized to produce electricity in power plants [58].
The subsequent phase entails the production of electricity in power plants. Subsequently, this electrical power is exchanged with suppliers, who subsequently transfer it to transmission networks. The transmission network is tasked with conveying electricity across extended distances, typically at elevated voltages, to regional distribution networks. Subsequently, the distribution network transports power to final customers, encompassing residential dwellings, commercial establishments, and industrial facilities. In this stage, transformers are employed to decrease voltage levels to ensure safe utilization within buildings. Ultimately, the supply stage encompasses the process of invoicing customers for their electricity consumption and delivering customer support [59].
Business stakeholders in the electrical supply chain encompass a wide array of companies engaged in the production, transmission, distribution, and provision of electricity (Fig. 4). Generation firms have the responsibility of creating electricity using a variety of sources, including coal, natural gas, nuclear power, hydroelectric power, and renewable sources such as wind and solar energy. Transmission system operators oversee the high-voltage transmission network, assuring the dependable and effective transportation of power across large distances. Distribution utilities have the responsibility of supplying energy directly to end users. They are also in charge of maintaining the low-voltage distribution network and performing tasks related to invoicing and customer care. Energy providers and retailers acquire power from generators or wholesale markets and distribute it to end users, providing a range of price options and services. Technology providers offer utilities and other stakeholder’s equipment [60], software, and services to update their infrastructure, enhance efficiency, and incorporate RE sources. Financial institutions offer financing and investment prospects for the development of infrastructure and energy projects. Consumers, including both residential and industrial users, play a crucial role in the electrical supply chain [61]. They have the power to shape demand patterns and affect energy consumption behaviour through their choices and actions. These commercial stakeholders collaborate to guarantee the dependable, cost-effective, and enduring provision of energy to fulfil the requirements of society.
Figure 4: Electricity supply chain
3.1.3 Non Business Stakeholders
Non-business stakeholders in the energy supply chain have significant influence over the development of laws, regulations, and practices that govern the sector. Regulators and politicians determine the parameters for the power business, defining regulations pertaining to pricing, competition, and environmental standards. Their role is to oversee the operations of utilities and energy suppliers, ensuring that they act in the best interest of the public. They also work towards fostering fair competition, protecting consumers, and ensuring environmental sustainability. Research institutes play a crucial role in advancing knowledge and technology in the electrical sector. They undertake studies and develop innovations that enhance the efficiency and sustainability of electricity generation, transmission, and distribution. Lobbyists and non-governmental organizations (NGOs) promote certain causes, such as the integration of RE, energy efficiency, or consumer rights, by influencing decision-makers and increasing awareness about important matters in the electricity sector [62]. These non-business stakeholders have a significant impact on the development of the power supply chain, making sure it meets societal demands and tackles environmental and economic obstacles [63].
Collaboration among the stakeholders involved leads to synergy and generates significant advantages for the system and each party involved [64]. These benefits include enhanced independence from fossil fuels, streamlined energy efficiency through various programs, attainment of RE targets, decreased carbon emissions, environmental protection, improved public health, and numerous economic benefits [65].
An in-depth understanding of the linkages and effects of stakeholders is important for a successful deployment of RE and sustainable green technologies. The electricity system is influenced by various stakeholders who can either have positive or negative effects on the implementation of renewable energy sources and small-scale generation technologies [66]. The framework encompasses not only the primary participants, but also the advantages and challenges, which aid in determining the direction of the impact.
Three distinct groups can be identified within the influential global organizations that have an impact on SGTs and RE implementation. These groups include technology development organizations like the Global SG Federation, organizations involved in shaping the direction of the energy industry such as the International Renewable Energy Agency, and environmental organizations like Greenpeace and WWF.
The primary goal of technology development organizations is to centralize all projects associated with SGTs on a global level and facilitate the dissemination of information worldwide. These organizations act as worldwide centres of knowledge on SGTs and policy issues, promoting cooperation between public and private sectors globally, and producing studies and summaries on important subjects. Organizations involved in developing strategies for the energy sector aim to assist countries worldwide in reaching their RE and efficiency goals by providing crucial data and statistics, advising on policy advancements, and offering expertise on financial matters and technology. Environmental organizations play an active role in advocating for sustainable energy and innovative technology, as well as providing guidance on energy policy [67]. These organizations offer concepts, solutions, computations, techniques, and tactics that assist in effectively addressing the primary challenges and resolving critical issues related to the implementation of RE and sustainable green technologies. Global organizations are demonstrating significant interest in the adoption of SGTs and exerting a favourable influence on their development [68].
3.1.5 Local Non-Profit and Non-Governmental Organizations
In the energy sector, numerous non-profit organizations (NPOs) and non-governmental organizations (NGOs) establish associations to consolidate their efforts and resources, thereby augmenting their authority and sway on policy-making entities. Non-profit organizations (NPOs) and non-governmental organizations (NGOs) can positively influence the implementation of sustainable green technologies and RE by providing relevant initiatives to the policymakers.
Technology platforms and task forces are separate classifications of stakeholders [69]. Technology stages serve as dedicated spaces where participants gather, process, and disseminate information and capabilities. Technology platforms serve as a strategic guide for the development of SGTs for various stakeholders. These platforms facilitate the collaboration of policymakers, Transmission System Operators (TSOs), Distribution System Operators (DSOs), manufacturers, large- and small-scale power generators, prosumer organizations, research centres, academics, and other relevant stakeholders [70].
3.1.6 Associations of Local and Regional Authorities
Local as well as regional authorities have the option to collaborate and actively contribute towards the accomplishment of government energy-related objectives. As the most immediate connection to the inhabitants, they possess direct access to them and have a deeper understanding of their needs (Fig. 5). This enables them to coordinate various initiatives that involve private houses and small businesses [71].
Figure 5: Regulation and collaboration between different authorities
3.1.7 Interplay of Consumers, Suppliers and Appliance Makers
The interactions among consumers, suppliers, and appliance manufacturers constitute a triangular structure that depicts the unique system in which the explicit and implicit regulations, guidelines, and legislation take shape and become tangible. This system serves as an indication to determine if the regulations and policies of other stakeholders are in harmony and free from contradictions [72]. Even when all stakeholders have good influence and genuine interest, the outcome may still be unfavourable. The fundamental issue resides in congruence. Multiple stakeholders can exert a beneficial influence. However, the absence of synergy and alignment across various activities, visions (particularly in task prioritizing), and actions might result in the opposite outcome and exacerbate preexisting issues.
4 Stakeholder Roles and Functions
It is imperative to give due attention to the fundamental aspects, such as the historical context, pertaining to the identification of key stakeholders and their contributions towards the progress of the power grid. Partners transition from being creators of utility and vitality to becoming purchasers, intermediaries, technology providers, and consultants. One crucial aspect of recognizing the SG is the comprehensive acquisition, encompassing all relevant elements. The system entails government producers and controllers who have the responsibility of assuring the efficacy of policies for industrial modernization projects and satisfying the comprehensive requirements [73]. The main advantage of SG enhancement for these stakeholders is the lowering of energy expenses, decreased reliance on foreign oil, improved efficiency, and higher reliability of electricity provision [74]. The creation of the intelligent grid involves multiple players, such as government agencies, manufacturers, research institutes, and other relevant parties. The Department of Energy presents a comprehensive review of the different facets associated with the advancement of SGT in their article called “The Smart Grid: An Introduction” [75]. SG is an innovative solution that uses principles, concepts, and technologies from the internet to transform the electric business. This conversion enables the updating of the electrical grid [76]. The primary characteristics of a SG are computerized communication, plug and play capabilities, sophisticated metering infrastructure for customer integration, facilities for growing customer coverage, interoperability based on standards, and cost-effective communication and equipment [77]. This project focuses on integrating and advancing grid representation technology in order to improve overall grid awareness. This entails the immediate integration of sensor data, weather information, and grid modelling with geographical data [78].
4.1 Drivers for Smart Grid for Different Stakeholders in India
The forces currently propelling the advancement of the SG are diverse and compelling (Fig. 6). The growing global awareness of environmental issues is fuelling the advancement of RE on a larger scale than ever before. The widespread deployment of wind, solar, and other RE sources poses operational challenges due to the intermittent nature of these sources. A framework capable of effectively managing a combination of different age groups, along with a significant level of sustainability, will become essential for these technologies to achieve their full potential. The drivers [79] encompass a range of factors.
Figure 6: Drivers for SG involving different stakeholders
It is essential for utilities to decrease transmission and distribution (T&D) losses to less than 15 percent in order to improve efficiency and reduce energy waste. Efficient peak load management guarantees the system’s ability to handle periods of high demand without sacrificing stability. In addition, reducing the cost of purchasing power is crucial for achieving economic sustainability, as it enables more efficient allocation of resources and facilitates investment in infrastructure enhancements. Deploying sophisticated grid technology promotes the development of a more robust and self-repairing grid, capable of autonomously detecting and rectifying defects. The incorporation of renewable energy (RE) sources into the power grid not only promotes sustainability objectives but also diminishes dependence on fossil fuels, hence enhancing the resilience and effectiveness of the energy system.
Customers benefit from a substantial improvement in the dependability of their power supply, which eliminates the necessity for power outages, diesel generator sets (DG sets), and inverters. The enhanced supply quality eliminates the need for voltage stabilizers, guaranteeing a more reliable and uniform electrical flow. An interface that is easy to use and direct enables consumers to effectively control their energy consumption and seamlessly include renewable energy sources into their energy blend. The enhanced control and decision-making capacity leads to a better-informed and actively involved consumer base, facilitating a transition towards sustainable energy practices.
4.1.3 Government and Regulators
Governments and regulators prioritize client pleasure to enhance the financial stability of utilities. For fair and equal pricing, it is crucial to adopt a tariff-neutral framework overhaul and focus on continual development. Minimizing carbon emissions and other harmful substances is an essential objective that helps create a more pristine environment and improves the well-being of the population. Smart Grids (SGs) are crucial for achieving the overall goals of the energy and power framework in India. They improve efficiency and performance in the country’s power system. The motivation for change arises from both external considerations, such as the anticipation of a low-carbon future and the reduction of greenhouse gas emissions, as well as internal factors, such as the necessity to replace deteriorating infrastructure.
4.1.4 Wide-Area Situational Awareness
It guarantees that administrators of India’s extensive energy infrastructure have the necessary equipment to obtain a complete understanding of their systems, allowing them to efficiently monitor and operate them.
4.1.5 Coordination of the Bulk Power Systems with New and Emerging Technologies
This Identifies principles of progress that facilitate the adaptation and expansion of renewable resources, demand response, and energy storage to handle various difficulties in the mass power system. Similarly, differentiate standards that facilitate the adoption of emerging technology, such as electric mobility.
The introduction of open access (OA) was intended to introduce competition in the electricity sector by allowing eligible users to purchase power from other providers at reasonable prices, instead of relying solely on DISCOMs. This was done with the consideration of economic feasibility [80].
DISCOMs have stated that the deployment of OA has resulted in significant financial consequences. The participants emphasized that tariff design is a significant obstacle, as the retail tariff system disproportionately favors consumers who pay lower tariffs due to cross-subsidization. Furthermore, the current tariff designing may not accurately represent the actual costs incurred through the DISCOMs for both fixed and variable charges borne by consumers. This results in a situation where customers are not paying enough to cover the costs, which is caused by their migration to OA [81]. Consumers, particularly industrial and commercial ones, as well as DISCOMs, have raised this issue with several commissioners during public hearings on energy rate. The Andhra Pradesh Electricity Regulatory Commission (APERC) and Uttar Pradesh Electricity Regulatory Commission (UPERC), among other regulatory commissions, have recognized these difficulties and have requested a detailed explanation or discussion paper to facilitate an informed decision-making process.
DISCOMs expressed worries about the insufficient compensation provided by the OA charges to cover their losses. On the other hand, consumers highlighted concerns that these charges are making it economically unfeasible for them to buy power through the OA route. The imposition of these fees has dissuaded customers from embracing the choice of OA. Presently, the expense of electricity acquired via OA exceeds the officially set rate for the Distribution Companies (DISCOMs).
Therefore, customers find it financially feasible to meet their energy needs by purchasing power from the DISCOMs [82]. The analysis compares the cost of electricity purchased from the exchange, assuming a price of INR 4 per unit and a quantity of 100 MW for 24 h, with the tariff offered by DISCOMs to 11-kV industrial consumers. The comparison is conducted using data from April 2017 and relies on the IEX landed cost tool. Upon comparison, it is evident that the expense of supplying power to the 11-kV industrial consumer through OA is more than the rate that needs to be paid to the Distribution Companies (DISCOMs).
Certain states offer extra financial assistance to industrial users [83], making the OA approach even more economically impractical.
Every OA consumer is required to utilize the distribution licensee network in order to access electricity through the OA route until it reaches the point of consumption. The withdrawal behavior of OA clients, if it deviates, might have a detrimental impact on the scheduling and load management of the distribution licensee.
The expansion in the number of OA consumers would lead to a significant problem regarding grid management [84]. Due to operational challenges, stakeholders have recommended examining and resolving these issues from a risk management standpoint. This means ensuring that the responsibility for managing the risks is appropriately distributed among the relevant stakeholders.
Consumers have noticed a significant delay in the response time for online applications. Most applications are primarily refused owing to technical restrictions caused by network congestion [85]. One significant limitation is the lack of accessible information regarding the availability of transmission and distribution networks, as well as the clearances granted to OA applications. This hinders the ability to present a strong argument against the rejection of these applications. The absence of technical data complicates the presentation of their argument. There is a need for a universal framework to facilitate the management of OA. This unified interface has the potential to streamline the process of approving applications.
The matter of consumer knowledge and capacity building is a substantial concern voiced by all stakeholders, encompassing both DISCOMs and consumers [86]. Further, DISCOMs have reported that the majority of OA applications are denied due to procedural difficulties, specifically related to documentation. They indicated that consumers had a limited understanding of the application process, resulting in frequent rejections owing to incomplete submissions. Consumers and DISCOM officials alike were perceived to have a lack of information regarding the application process for OA. The main disadvantages are a lack of expertise in technical matters and a lack of thorough explanation about the grounds for rejecting OA applications [87].
Smart grid operation involves many modes, aims, and services aimed at maximizing energy efficiency, improving system dependability, and integrating renewable energy sources. Smart grids function in several modes, such as regular operation, emergency response, and islanding mode. Smart grids function by optimizing the distribution of power, maintaining a real-time balance between supply and demand, and minimizing losses in transmission and distribution. Smart grids have the ability to promptly identify and separate impacted regions, redirect electricity, and reinstate utilities in times of emergencies, hence guaranteeing resilience. During islanding mode, specific sections of the electrical grid have the capability to function autonomously by utilizing local renewable energy sources and storage devices. This allows for a continuous power supply, even in the event of widespread power outages.
The main goals of smart grid operation are to enhance grid dependability and stability, decrease operational expenses, and improve energy security. Smart grids accomplish these goals by utilizing sophisticated monitoring and control systems, implementing proactive maintenance strategies, and integrating distributed energy resources (DERs). These systems utilize up-to-the-minute data and advanced analysis to predict and tackle possible problems before they worsen, thereby minimizing periods of inactivity and lowering expenses related to maintenance. Smart grids provide a range of services, including demand response, which encourages consumers to decrease or adjust their energy consumption during periods of high demand, and ancillary services, which help maintain the stability and efficiency of the grid. Frequency regulation and voltage management are vital for ensuring the integrity of the grid. Smart grids offer these services by utilizing automated technologies and sophisticated algorithms.
Recent instances of smart grid implementation demonstrate their efficacy and adaptability. The Smart Grid Investment Grant (SGIG) program in the United States has provided funding for several projects that have shown substantial enhancements in grid performance. An exemplary initiative is the implementation of advanced metering infrastructure (AMI) in Texas, which has led to a decrease in peak demand and a reduction in energy usage. The INTERFLEX project in France and Germany has investigated novel methods to include renewable energy sources, enhance demand response capabilities, and enhance grid automation throughout Europe. The Smart Grid Pilot initiatives effort in India has successfully implemented multiple initiatives in different states, with a specific focus on areas such as Advanced Metering Infrastructure (AMI), outage management systems, and distributed generation. An example of this is the Puducherry Smart Grid project, which has effectively showcased the advantages of smart meters and automated demand response. This has resulted in enhanced energy efficiency and increased customer satisfaction. These examples demonstrate the varied and ever-changing character of smart grid operations, highlighting their capacity to adapt to the changing requirements of modern power systems while promoting sustainability and resilience objectives.
A primary goal of the Act is to enhance competition by facilitating OA. As a result, the relevant commissions have informed on the regulations for OA that apply across states and inside a single state. Despite the presence of these restrictions, numerous concerns have arisen during the execution of OA. In order to foster competitiveness through OA, these issues must be resolved [88].
One significant regulatory obstacle highlighted by the DISCOMs is the absence of a clear definition of the ‘competent authority’ responsible for certifying the indicated criteria. Currently, several DISCOMs have varying procedures regarding the competent authority responsible for certifying captive status under the Rule 3(1) of the Electricity Rules, 2005.
The power distribution companies (DISCOMs) also highlighted the difficulty of verifying the constantly changing ownership structure (26% of total ownership) of group captive users. Presently, the authorities perform an annual verification of the distribution of shares to ascertain the qualification for the benefits granted to captive consumers. Nevertheless, DISCOMs have observed that the majority of group captive clients alter their ownership structure within one year of being designated as captive, thereby taking advantage of the incentives for the entire year [89].
OA consumers also emphasized the lack of consistency in the state legislation. The regulations identified various significant challenges, such as the necessity for advanced technology and infrastructure, the requirement for a no objection certificate (NOC), the procedure for bill and DSM settlement [90], specific time slots for power trading, and restrictions on bidding quantities, among other issues. Stakeholders stressed the need to reassess regulations in response to the impact of OA on financial and operational aspects, as well as the existing regulatory difficulties [91]. They held the belief that establishing uniform laws with a well-defined structure is crucial to guarantee the seamless implementation and general prosperity of OA in the Indian power industry [92].
The electrical market in India encompasses a wide range of important participants, each fulfilling a crucial function in the growth and functioning of the sector. The national and state governments are key stakeholders, responsible for establishing policies and regulatory frameworks through entities such as the Ministry of Power and the National Electricity Regulatory Commission (CERC) (Fig. 8, Tables 2–4). Utility firms, encompassing both publicly-owned and privately-owned organizations, have the duty of producing, transmitting, and distributing electricity, with the goal of maintaining a dependable power supply and overseeing grid infrastructure. Technology providers and equipment manufacturers play a crucial role in offering cutting-edge technologies and solutions that are necessary for the upgrading of the power grid and the incorporation of renewable energy sources. Financial institutions and investors play a vital role in infrastructure projects by providing the required funds and ensuring the financial stability of the sector. Consumers, including individuals, businesses, and industries, are becoming more involved in energy conservation efforts and participating in programs that allow them to adjust their energy usage based on demand. NGOs and research institutes play a crucial role in promoting sustainable energy policies and performing essential research to support policy and technological progress. Collectively, these participants constitute an intricate and interdependent network that propels the expansion, durability, and adaptability of India’s electrical industry.
Figure 7: Smartgrid driver for different stakeholders
Figure 8: Map showing list of key stakeholders
The progress of smart grids in India is propelled by a confluence of market dynamics, technological innovations, and governmental initiatives, with the objective of updating the nation’s energy infrastructure. Primary market drivers encompass the increasing demand for dependable and efficient energy distribution, the incorporation of renewable energy sources, and the necessity to minimize transmission losses. Accelerated urbanization, enhanced electrification of rural regions, and the shift towards greener energy sources are driving the demand for more intelligent grid solutions. The increase in energy consumption resulting from industrial and economic expansion has rendered the implementation of smart grids crucial for optimizing energy distribution and maintaining grid stability.
Participants in India’s smart grid ecosystem, comprising governmental entities, energy utilities, private enterprises, and consumers, each assume a crucial role. The government is chiefly accountable for establishing legislative frameworks, providing regulatory assistance, and offering financial incentives that promote the implementation of smart grid technologies. Energy utilities are responsible for deploying these technologies while maintaining grid dependability, cost-effectiveness, and the incorporation of renewable energy. Private technology companies boost operational efficiency by creating novel solutions, like smart meters, enhanced communication systems, and grid management software. Ultimately, both industrial and residential consumers are anticipated to engage in demand-side management strategies and actively participate in the energy market via distributed generation and storage systems. A cooperative strategy among these stakeholders is essential for the success of India’s smart grid ambition. The coordination of duties, investments, and incentives across sectors would facilitate the rapid implementation of smart grids, so assuring a more robust, sustainable, and economical energy system that aligns with India’s long-term energy objectives.
Acknowledgement: The authors extend their appreciation to Faculty of Management Studies, Sri Sri University, Cuttack, India.
Funding Statement: The authors received no specific funding for this study.
Author Contributions: Study conception and design: Abhay Sanatan Satapathy, Suresh Kumar Sahoo; data collection: Abhay Sanatan Satapathy; analysis and interpretation of results: Abhay Sanatan Satapathy, Asit Mohanty, Suresh Kumar Sahoo; draft manuscript preparation: Abhay Sanatan Satapathy, Suresh Kumar Sahoo, Asit Mohanty, Yasser Fouad, Manzoore Elahi Mohammad Soudagar, Erdem Cuce. All authors reviewed the results and approved the final version of the manuscript.
Availability of Data and Materials: Not applicable.
Ethics Approval: Not applicable.
Conflicts of Interest: The authors declare that they have no conflicts of interest to report regarding the present study.
References
1. M. Fotopoulou, P. Pediaditis, N. Skopetou, D. Rakopoulos, S. Christopoulos and A. Kartalidis, “A review of the energy storage systems of non-interconnected European Islands,” Sustainability, vol. 16, no. 4, 2024, Art. no. 1572. doi: 10.3390/su16041572. [Google Scholar] [CrossRef]
2. T. Teng, X. Zhang, H. Dong, and Q. Xue, “A comprehensive review of energy management optimization strategies for fuel cell passenger vehicle,” Int. J. Hydrogen Energy, vol. 45, no. 39, pp. 20293–20303, Aug. 2020. doi: 10.1016/j.ijhydene.2019.12.202. [Google Scholar] [CrossRef]
3. A. Arif, Z. Wang, J. Wang, and C. Chen, “Power distribution system outage management with co-optimization of repairs, reconfiguration, and DG dispatch,” IEEE Trans. Smart Grid, vol. 9, no. 5, pp. 4109–4118, Sep. 2018. doi: 10.1109/TSG.2017.2650917. [Google Scholar] [CrossRef]
4. H. F. Habib, C. R. Lashway, and O. A. Mohammed, “A review of communication failure impacts on adaptive microgrid protection schemes and the use of energy storage as a contingency,” IEEE Trans. Ind. Appl., vol. 54, no. 2, pp. 1194–1207, 2018. doi: 10.1109/TIA.2017.2776858. [Google Scholar] [CrossRef]
5. A. Mohanty, A. K. Ramasamy, R. Verayiah, and S. Mohanty, “Neighborhood and community battery projects: A systematic analysis of their current state and future prospects,” J. Energy Storage, vol. 95, 2024, Art. no. 112525. doi: 10.1016/j.est.2024.112525. [Google Scholar] [CrossRef]
6. M. Panteli and P. Mancarella, “The grid: Stronger, bigger, smarter?: Presenting a conceptual framework of power system resilience?” IEEE Power Energy Mag., vol. 13, no. 3, pp. 58–66, 2015. doi: 10.1109/MPE.2015.2397334. [Google Scholar] [CrossRef]
7. R. Kappagantu and S. A. Daniel, “Challenges and issues of smart grid implementation: A case of Indian scenario,” J. Electr. Syst. Inf. Technol., vol. 5, no. 3, pp. 453–467, 2018. doi: 10.1016/j.jesit.2018.01.002. [Google Scholar] [CrossRef]
8. A. P. Heynen, P. A. Lant, S. Smart, S. Sridharan, and C. Greig, “Off-grid opportunities and threats in the wake of India’s electrification push,” Energy Sustain. Soc., vol. 9, no. 1, 2019. doi: 10.1186/s13705-019-0198-z. [Google Scholar] [CrossRef]
9. S. R. Das, P. K. Ray, A. Mohanty, and T. K. Panigrahi, “Application of artificial intelligence techniques for improvement of power quality using hybrid filters,” in Advances in Intelligent Systems and Computing, Singapore: Springer, 2019, pp. 719–729. doi: 10.1007/978-981-13-8676-3_61. [Google Scholar] [CrossRef]
10. S. R. Paital, P. K. Ray, and A. Mohanty, “A review on stability enhancement in SMIB system using artificial intelligence based techniques,” in 2018 IEEMA Eng. Infinite Conf. (eTechNxT), New Delhi, India, IEEE, 2018. doi: 10.1109/etechnxt.2018.8385324. [Google Scholar] [CrossRef]
11. A. Mohanty and M. Viswavandy, “A novel ANN based UPFC for voltage stability and reactive power management in a remote hybrid system,” Proc. Comput. Sci., vol. 48, pp. 555–560, 2015. doi: 10.1016/j.procs.2015.04.135. [Google Scholar] [CrossRef]
12. S. D. Comello, S. J. Reichelstein, A. Sahoo, and T. S. Schmidt, “Enabling mini-grid development in Rural India,” World Dev., vol. 93, pp. 94–107, 2017. doi: 10.1016/j.worlddev.2016.12.029. [Google Scholar] [CrossRef]
13. M. H. Maruf, M. A. ul Haq, S. K. Dey, A. Al Mansur, and A. S. M. Shihavuddin, “Adaptation for sustainable implementation of smart grid in developing countries like Bangladesh,” Energy Rep., vol. 6, pp. 2520–2530, 2020. doi: 10.1016/j.egyr.2020.09.010. [Google Scholar] [CrossRef]
14. S. Borlase, Smart Grids: Infrastructure, Technology, and Solutions. CRC Press, 2017. doi: 10.1201/b13003. [Google Scholar] [CrossRef]
15. P. Palensky and F. Kupzog, “Smart grids,” Annu Rev. Environ. Resour., vol. 38, no. 1, pp. 201–226, 2013. doi: 10.1146/annurev-environ-031312-102947. [Google Scholar] [CrossRef]
16. S. Luthra, S. Kumar, R. Kharb, M. F. Ansari, and S. L. Shimmi, “Adoption of smart grid technologies: An analysis of interactions among barriers,” Renew. Sustain. Energy Rev., vol. 33, pp. 554–565, 2014. doi: 10.1016/j.rser.2014.02.030. [Google Scholar] [CrossRef]
17. P. Siano, “Demand response and smart grids—A survey,” Renew. Sustain. Energy Rev., vol. 30, pp. 461–478, 2014. doi: 10.1016/j.rser.2013.10.022. [Google Scholar] [CrossRef]
18. M. Fadaeenejad, A. M. Saberian, M. Fadaee, M. A. M. Radzi, H. Hizam and M. Z. A. AbKadir, “The present and future of smart power grid in developing countries,” Renew. Sustain. Energy Rev., vol. 29, pp. 828–834, 2014. doi: 10.1016/j.rser.2013.08.072. [Google Scholar] [CrossRef]
19. A. Luque, M. Colin, S. Marvin, “Smart urbanism: Cities, grids and alternatives?” in After Sustainable Cities. Routledge, pp. 86–102, 2014. doi: 10.4324/9780203074602-11. [Google Scholar] [CrossRef]
20. B. Shen, G. Ghatikar, Z. Lei, J. Li, G. Wikler and P. Martin, “The role of regulatory reforms, market changes, and technology development to make demand response a viable resource in meeting energy challenges,” Appl. Energy., vol. 130, pp. 814–823, 2014. doi: 10.1016/j.apenergy.2013.12.069. [Google Scholar] [CrossRef]
21. J. A. Cardenas, L. Gemoets, J. H. Ablanedo Rosas, and R. Sarfi, “A literature survey on Smart grid distribution: An analytical approach,” J. Clean. Prod., vol. 65, pp. 202–216, 2014. doi: 10.1016/j.jclepro.2013.09.019. [Google Scholar] [CrossRef]
22. J. Thakur and B. Chakraborty, “Intelli-grid: Moving towards automation of electric grid in India,” Renew Sustain. Energy Rev., vol. 42, pp. 16–25, 2015. doi: 10.1016/j.rser.2014.09.043. [Google Scholar] [CrossRef]
23. J. C. Stephens, E. J. Wilson, and T. R. Peterson, Smart Grid (R)Evolution: Electric Power Struggles. Cambridge: Cambridge University Press, 2015. doi: 10.1017/CBO9781107239029. [Google Scholar] [CrossRef]
24. A. K. Rohit and S. Rangnekar, “An overview of energy storage and its importance in Indian renewable energy sector: Part II—Energy storage applications, benefits and market potential,” J. Energy Storage, vol. 13, pp. 447–456, 2017. doi: 10.1016/j.est.2017.07.012. [Google Scholar] [CrossRef]
25. P. Barman and L. Dutta, “Charging infrastructure planning for transportation electrification in India: A review,” Renew Sustain. Energy Rev., vol. 192, 2024, Art. no. 114265. doi: 10.1016/j.rser.2023.114265. [Google Scholar] [CrossRef]
26. T. Ahmad, R. Madonski, D. Zhang, C. Huang, and A. Mujeeb, “Data-driven probabilistic machine learning in sustainable smart energy/smart energy systems: Key developments, challenges, and future research opportunities in the context of smart grid paradigm,” Renew. Sustain. Energy Rev., vol. 160, 2022, Art. no. 112128. doi: 10.1016/j.rser.2022.112128. [Google Scholar] [CrossRef]
27. O. Ellabban and H. Abu-Rub, “Smart grid customers’ acceptance and engagement: An overview,” Renew. Sustain. Energy Rev., vol. 65, pp. 1285–1298, 2016. doi: 10.1016/j.rser.2016.06.021. [Google Scholar] [CrossRef]
28. K. Sharma and L. Mohan Saini, “Performance analysis of smart metering for smart grid: An overview,” Renew. Sustain. Energy Rev., vol. 49, pp. 720–735, 2015. doi: 10.1016/j.rser.2015.04.170. [Google Scholar] [CrossRef]
29. O. Majeed Butt, M. Zulqarnain, and T. Majeed Butt, “Recent advancement in smart grid technology: Future prospects in the electrical power network,” Ain Shams Eng. J., vol. 12, no. 1, pp. 687–695, 2021. doi: 10.1016/j.asej.2020.05.004. [Google Scholar] [CrossRef]
30. C. Pang, P. Dutta, and M. Kezunovic, “BEVs/PHEVs as dispersed energy storage for V2B uses in the smart grid,” IEEE Trans. Smart Grid, vol. 3, no. 1, pp. 473–482, 2012. doi: 10.1109/TSG.2011.2172228. [Google Scholar] [CrossRef]
31. M. E. El-hawary, “The smart grid—State-of-the-art and future trends,” Electr. Power Components Syst., vol. 42, no. 3–4, pp. 239–250, 2014. doi: 10.1080/15325008.2013.868558. [Google Scholar] [CrossRef]
32. A. Al-Badi, R. Ahshan, N. Hosseinzadeh, R. Ghorbani, and E. Hossain, “Survey of smart grid concepts and technological demonstrations worldwide emphasizing on the oman perspective,” Appl. Syst. Innov., vol. 3, no. 1, 2020, Art. no. 5. doi: 10.3390/asi3010005. [Google Scholar] [CrossRef]
33. M. Meliani, A. El Barkany, I. El Abbassi, A. M. Darcherif, and M. Mahmoudi, “Energy management in the smart grid: State-of-the-art and future trends,” Int. J. Eng. Bus. Manag., vol. 13, 2021, Art. no. 184797902110329. doi: 10.1177/18479790211032920. [Google Scholar] [CrossRef]
34. J. Vasiljevska, J. Douw, A. Mengolini, and I. Nikolic, “An agent-based model of electricity consumer: Smart metering policy implications in Europe,” J. Artif. Soc. Soc. Simul., vol. 20, no. 1, 2017. doi: 10.18564/jasss.3150. [Google Scholar] [CrossRef]
35. A. Mohanty, M. Viswavandya, P. K. Ray, T. K. Panigrahi, and S. Mohanty, “Stability and optimisation of direct drive permanent magnet synchronous generator based tidal turbine,” Vacuum, vol. 166, pp. 341–350, 2019. doi: 10.1016/j.vacuum.2018.10.052. [Google Scholar] [CrossRef]
36. F. Caputo, B. Buhnova, and L. Walletzký, “Investigating the role of smartness for sustainability: Insights from the smart grid domain,” Sustain. Sci., vol. 13, no. 5, pp. 1299–1309, 2018. doi: 10.1007/s11625-018-0555-4. [Google Scholar] [CrossRef]
37. M. A. Raza, M. M. Aman, A. G. Abro, M. A. Tunio, K. L. Khatri and M. Shahid, “Challenges and potentials of implementing a smart grid for Pakistan’s electric network,” Energy Strateg. Rev., vol. 43, 2022, Art. no. 100941. doi: 10.1016/j.esr.2022.100941. [Google Scholar] [CrossRef]
38. S. R. Das, P. K. Ray, and A. Mohanty, “Improvement of power quality using advanced artificial neural network algorithm,” in 2018 IEEE Int. Conf. on Power Electron., Drives and Energy Syst. (PEDES), Chennai, India, IEEE, 2018. doi: 10.1109/pedes.2018.8707591. [Google Scholar] [CrossRef]
39. S. Reddy and J. P. Painuly, “Diffusion of renewable energy technologies—Barriers and stakeholders’ perspectives,” Renew. Energy, vol. 29, no. 9, pp. 1431–1447, 2004. doi: 10.1016/j.renene.2003.12.003. [Google Scholar] [CrossRef]
40. M. A. Ponce-Jara, E. Ruiz, R. Gil, E. Sancristóbal, C. Pérez-Molina and M. Castro, “Smart grid: Assessment of the past and present in developed and developing countries,” Energy Strateg. Rev., vol. 18, pp. 38–52, 2017. doi: 10.1016/j.esr.2017.09.011. [Google Scholar] [CrossRef]
41. C. L. Stimmel, Big Data Analytics Strategies for the Smart Grid. Auerbach Publications, 2014. doi: 10.1201/b17228. [Google Scholar] [CrossRef]
42. T. Ahmad et al., “Energetics systems and artificial intelligence: Applications of Industry 4. 0,” Energy Rep., vol. 8, pp. 334–361, 2022. doi: 10.1016/j.egyr.2021.11.256. [Google Scholar] [CrossRef]
43. A. Pan, M. Tian, B. Tang, and X. Yang, “Urban electric vehicle (EV) charging point design based on user travel big data,” IOP Conf. Ser. Earth Environ. Sci., vol. 300, no. 4, 2019, Art. no. 42091. doi: 10.1088/1755-1315/300/4/042091. [Google Scholar] [CrossRef]
44. X. Lei, H. Yu, Z. Shao, and L. Jian, “Optimal bidding and coordinating strategy for maximal marginal revenue due to V2G operation: Distribution system operator as a key player in China’s uncertain electricity markets,” Energy, vol. 283, 2023, Art. no. 128354. doi: 10.1016/j.energy.2023.128354. [Google Scholar] [CrossRef]
45. Q. Dang, D. Wu, and B. Boulet, “Community microgrid energy storage sizing considering EV Fleet batteries as supplemental resource,” in 2020 IEEE Electr. Power and Energy Conf. (EPEC), Edmonton, AB, Canada, IEEE, 2020. doi: 10.1109/epec48502.2020.9320089. [Google Scholar] [CrossRef]
46. X. Lei, H. Yu, B. Yu, Z. Shao, and L. Jian, “Bridging electricity market and carbon emission market through electric vehicles: Optimal bidding strategy for distribution system operators to explore economic feasibility in China’s low-carbon transitions,” Sustain. Cities Soc., vol. 94, 2023, Art. no. 104557. doi: 10.1016/j.scs.2023.104557. [Google Scholar] [CrossRef]
47. L. Colarullo and J. Thakur, “Second-life EV batteries for stationary storage applications in local energy communities,” Renew. Sustain. Energy Rev., vol. 169, 2022, Art. no. 112913. doi: 10.1016/j.rser.2022.112913. [Google Scholar] [CrossRef]
48. L. F. M. van Summeren, A. J. Wieczorek, G. J. T. Bombaerts, and G. P. J. Verbong, “Community energy meets smart grids: Reviewing goals, structure, and roles in Virtual Power Plants in Ireland, Belgium and the Netherlands,” Energy Res. Soc. Sci., vol. 63, 2020, Art. no. 101415. doi: 10.1016/j.erss.2019.101415. [Google Scholar] [CrossRef]
49. M. Emmanuel and R. Rayudu, “Communication technologies for smart grid applications: A survey,” J. Netw. Comput. Appl., vol. 74, pp. 133–148, 2016. doi: 10.1016/j.jnca.2016.08.012. [Google Scholar] [CrossRef]
50. F. Rohde and S. Hielscher, “Smart grids and institutional change: Emerging contestations between organisations over smart energy transitions,” Energy Res. Soc. Sci., vol. 74, 2021, Art. no. 101974. doi: 10.1016/j.erss.2021.101974. [Google Scholar] [CrossRef]
51. P. Guo, V. O. K. Li, and J. C. K. Lam, “Smart demand response in China: Challenges and drivers,” Energy Policy, vol. 107, pp. 1–10, 2017. doi: 10.1016/j.enpol.2017.04.019. [Google Scholar] [CrossRef]
52. G. G. Dranka and P. Ferreira, “Towards a smart grid power system in Brazil: Challenges and opportunities,” Energy Policy, vol. 136, 2020, Art. no. 111033. doi: 10.1016/j.enpol.2019.111033. [Google Scholar] [CrossRef]
53. B. K. Chaturvedi, A. Nautiyal, T. C. Kandpal, and M. Yaqoot, “Projected transition to electric vehicles in India and its impact on stakeholders,” Energy for Sustain. Dev., vol. 66, pp. 189–200, 2022. doi: 10.1016/j.esd.2021.12.006. [Google Scholar] [CrossRef]
54. M. Hashmi, S. Hanninen, and K. Maki, “Survey of smart grid concepts, architectures, and technological demonstrations worldwide,” in 2011 IEEE Pes Conf. On Innov. Smart Grid Technol. Latin America (ISGT LA), Medellin, Colombia, IEEE, 2011. doi: 10.1109/isgt-la.2011.6083192. [Google Scholar] [CrossRef]
55. S. Junlakarn, P. Kokchang, and K. Audomvongseree, “Drivers and challenges of peer-to-peer energy trading development in Thailand,” Energies, vol. 15, no. 3, 2022, Art. no. 1229. doi: 10.3390/en15031229. [Google Scholar] [CrossRef]
56. S. Nyborg and I. Røpke, “Constructing users in the smart grid—Insights from the Danish eFlex project,” Energy Effic., vol. 6, no. 4, pp. 655–670, 2013. doi: 10.1007/s12053-013-9210-1. [Google Scholar] [CrossRef]
57. D. Pramangioulis, K. Atsonios, N. Nikolopoulos, D. Rakopoulos, P. Grammelis and E. Kakaras, “A methodology for determination and definition of key performance indicators for smart grids development in Island energy systems,” Energies, vol. 12, no. 2, 2019, Art. no. 242. doi: 10.3390/en12020242. [Google Scholar] [CrossRef]
58. C. -H. Lo and N. Ansari, “The progressive smart grid system from both power and communications aspects,” IEEE Commun. Surv. Tutorials, vol. 14, no. 3, 2011. doi: 10.1109/SURV.2011.072811.00089. [Google Scholar] [CrossRef]
59. N. P. Rana, S. Luthra, S. K. Mangla, R. Islam, S. Roderick and Y. K. Dwivedi, “Barriers to the development of smart cities in indian context,” Inf. Syst. Front., vol. 21, no. 3, pp. 503–525, 2018. doi: 10.1007/s10796-018-9873-4. [Google Scholar] [CrossRef]
60. A. Datta and P. Mohanty, “Enterprise GIS and smart electric grid for India’s power sector,” in 2013 IEEE PES Innov. Smart Grid Technol. Conf. (ISGT), IEEE, 2013. doi: 10.1109/isgt.2013.6497806. [Google Scholar] [CrossRef]
61. E. Espe, V. Potdar, and E. Chang, “Prosumer communities and relationships in smart grids: A literature review, evolution and future directions,” Energies, vol. 11, no. 10, 2018, Art. no. 2528. doi: 10.3390/en11102528. [Google Scholar] [CrossRef]
62. S. Trommer, “Transformations in trade politics: West African civil society participation in economic partnership negotiations with the european union,” Doctoral dissertation, Univ. of Helsinki, Finland, 2012. doi: 10.5040/9781474201377.ch-021. [Google Scholar] [CrossRef]
63. M. A. Brown, S. Zhou, and M. Ahmadi, “Smart grid governance: An international review of evolving policy issues and innovations,” WIREs Energy Environ., vol. 7, no. 5, 2018. doi: 10.1002/wene.290. [Google Scholar] [CrossRef]
64. K. Shenai and K. Shah, “Smart DC micro-grid for efficient utilization of distributed renewable energy,” in IEEE 2011 EnergyTech, Cleveland, OH, USA, IEEE, 2011. doi: 10.1109/energytech.2011.5948505. [Google Scholar] [CrossRef]
65. M. A. Brown and S. Zhou, “Smart-grid policies,” in Advances in Energy Systems: The Large-Scale Renewable Energy Integration Challenge, 2019, pp. 127–147. doi: 10.1002/9781119508311.ch8. [Google Scholar] [CrossRef]
66. W. Yuan, J. Wang, F. Qiu, C. Chen, C. Kang and B. Zeng, “Robust optimization-based resilient distribution network planning against natural disasters,” IEEE Trans. Smart Grid, vol. 7, no. 6, pp. 2817–2826, 2016. doi: 10.1109/TSG.2015.2513048. [Google Scholar] [CrossRef]
67. J. G. Bhatt and O. K. Jani, “Smart grid: Energy backbone of smart city and e-democracy,” in E-Democracy for Smart Cities, Singapore: Springer, 2017, pp. 319–366. doi: 10.1007/978-981-10-4035-1_11. [Google Scholar] [CrossRef]
68. F. Ullah, S. Qayyum, M. J. Thaheem, F. Al-Turjman, and S. M. E. Sepasgozar, “Risk management in sustainable smart cities governance: A TOE framework,” Technol Forecast. Soc. Change., vol. 167, 2021, Art. no. 120743. doi: 10.1016/j.techfore.2021.120743. [Google Scholar] [CrossRef]
69. P. Suresh et al., “Cloud-based big data analysis tools and techniques towards sustainable smart city services,” in Adv. in Computat. Intell. and Robot., 2021, pp. 63–90. doi: 10.4018/978-1-7998-7468-3.ch004. [Google Scholar] [CrossRef]
70. Y. Hertig and S. Teufel, “Prosumer cooperation behavior: Implications for prosumer community design,” J. Electron. Sci. Technol., vol. 14, no. 4, pp. 298–310, 2016. doi: 10.11989/JEST.1674-862X.605291. [Google Scholar] [CrossRef]
71. H. Busch and K. McCormick, “Local power: Exploring the motivations of mayors and key success factors for local municipalities to go 100% renewable energy,” Energy Sustain. Soc., vol. 4, no. 1, pp. 1–15, 2014. doi: 10.1186/2192-0567-4-5. [Google Scholar] [CrossRef]
72. W. Feng, K. Sun, Y. Guan, J. M. Guerrero, and X. Xiao, “Active power quality improvement strategy for grid-connected microgrid based on hierarchical control,” IEEE Trans. Smart Grid, vol. 9, no. 4, pp. 3486–3495, 2018. doi: 10.1109/tsg.2016.2633298. [Google Scholar] [CrossRef]
73. B. Warbroek, T. Hoppe, H. Bressers, and F. Coenen, “Testing the social, organizational, and governance factors for success in local low carbon energy initiatives,” Energy Res. Soc. Sci., vol. 58, Dec. 2019. doi: 10.1016/J.ERSS.2019.101269. [Google Scholar] [CrossRef]
74. N. Safaei, D. Banjevic, and A. K. S. Jardine, “Workforce planning for power restoration: An integrated simulation-optimization approach,” IEEE Trans. Power Syst., vol. 27, no. 1, pp. 442–449, 2012. doi: 10.1109/tpwrs.2011.2166090. [Google Scholar] [CrossRef]
75. B. K. Bose and F. (Fred) Wang, “Energy, environment, power electronics, renewable energy systems, and smart grid,” in Power Electronics in Renewable Energy Systems and Smart Grid. Wiley, 2019, pp. 1–83. doi: 10.1002/9781119515661.ch1. [Google Scholar] [CrossRef]
76. M. Shahidehpour and M. Fotuhi-Friuzabad, “Grid modernization for enhancing the resilience, reliability, economics, sustainability, and security of electricity grid in an uncertain environment,” Sci. Iran., vol. 23, no. 6, pp. 2862–2873, 2016. doi: 10.24200/sci.2016.3995. [Google Scholar] [CrossRef]
77. A. Paaso, D. Kushner, S. Bahramirad, and A. Khodaei, “Grid modernization is paving the way for building smarter cities [technology leaders],” IEEE Electrif. Mag., vol. 6, no. 2, pp. 6–108, 2018. doi: 10.1109/MELE.2018.2816848. [Google Scholar] [CrossRef]
78. N. Erdogan, D. B. Murray, J. Giebhardt, M. Wecker, and J. Donegan, “Real-time hardware emulation of a power take-off model for grid-connected tidal energy systems,” in 2019 IEEE Int. Electr. Mach.& Drives Conf. (IEMDC), San Diego, CA, USA, IEEE, 2019. doi: 10.1109/iemdc.2019.8785358. [Google Scholar] [CrossRef]
79. A. Hirsch, Y. Parag, and J. Guerrero, “Microgrids: A review of technologies, key drivers, and outstanding issues,” Renew. Sustain. Energy Rev., vol. 90, pp. 402–411, Jul. 2018. doi: 10.1016/j.rser.2018.03.040. [Google Scholar] [CrossRef]
80. E. A. Martínez Ceseña, N. Good, A. L. A. Syrri, and P. Mancarella, “Techno-economic and business case assessment of multi-energy microgrids with co-optimization of energy, reserve and reliability services,” Appl. Energy, vol. 210, pp. 896–913, Jan. 2018. doi: 10.1016/j.apenergy.2017.08.131. [Google Scholar] [CrossRef]
81. À Alonso, J. de la Hoz, H. Martín, S. Coronas, and J. Matas, “Individual vs. Community: Economic assessment of energy management systems under different regulatory frameworks,” Energies, vol. 14, no. 3, Feb. 2021. doi: 10.3390/EN14030676. [Google Scholar] [CrossRef]
82. A. L. S. de Sa, P. S. Lavieri, Y. T. Cheng, E. Hajhashemi, and G. J. M. Oliveira, “Modelling driver’s response to demand management strategies for electric vehicle charging in Australia,” Energy Res. Soc. Sci., vol. 103, Sep. 2023. doi: 10.1016/j.erss.2023.103218. [Google Scholar] [CrossRef]
83. E. Vanadzina, G. Mendes, S. Honkapuro, A. Pinomaa, and H. Melkas, “Business models for community microgrids,” in Int. Conf. Eur. Energy Mark, Ljubljana, Slovenia, EEM, Sep. 2019. doi: 10.1109/EEM.2019.8916368. [Google Scholar] [CrossRef]
84. A. Paudel, K. Chaudhari, C. Long, and H. B. Gooi, “Peer-to-peer energy trading in a prosumer-based community microgrid: A game-theoretic model,” IEEE Trans. Ind. Electron., vol. 66, no. 8, pp. 6087–6097, 2019. doi: 10.1109/TIE.2018.2874578. [Google Scholar] [CrossRef]
85. V. Z. Gjorgievski, S. Cundeva, and G. E. Georghiou, “Social arrangements, technical designs and impacts of energy communities: A review,” Renew. Energy, vol. 169, pp. 1138–1156, May 2021. doi: 10.1016/j.renene.2021.01.078. [Google Scholar] [CrossRef]
86. K. Sperling, “How does a pioneer community energy project succeed in practice? The case of the SamsØ Renewable Energy Island,” Renew. Sustain. Energy Rev., vol. 71, pp. 884–897, 2017. doi: 10.1016/j.rser.2016.12.116. [Google Scholar] [CrossRef]
87. I. F. G. Reis, I. Gonçalves, M. A. R. Lopes, and C. Henggeler Antunes, “Business models for energy communities: A review of key issues and trends,” Renew. Sustain. Energy Rev., vol. 144, Jul. 2021. doi: 10.1016/J.RSER.2021.111013. [Google Scholar] [CrossRef]
88. P. Asmus, “Microgrids, virtual power plants and our distributed energy future,” Electr. J., vol. 23, no. 10, pp. 72–82, Dec. 2010. doi: 10.1016/j.tej.2010.11.001. [Google Scholar] [CrossRef]
89. J. Guerrero, D. Gebbran, S. Mhanna, A. C. Chapman, and G. Verbič, “Towards a transactive energy system for integration of distributed energy resources: Home energy management, distributed optimal power flow, and peer-to-peer energy trading,” Renew. Sustain. Energy Rev., vol. 132, Oct. 2020. doi: 10.1016/J.RSER.2020.110000. [Google Scholar] [CrossRef]
90. A. Umar, D. Kumar, and T. Ghose, “Blockchain-based decentralized energy intra-trading with battery storage flexibility in a community microgrid system,” Appl. Energy, vol. 322, 2022. Art. no. 119544. doi: 10.1016/j.apenergy.2022.119544. [Google Scholar] [CrossRef]
91. Y. Deng, Y. Zhang, F. Luo, and Y. Mu, “Hierarchical energy management for community microgrids with integration of second-life battery energy storage systems and photovoltaic solar energy,” IET Energy Syst. Integr., vol. 4, no. 2, pp. 206–219, Jun. 2022. doi: 10.1049/esi2.12055. [Google Scholar] [CrossRef]
92. Y. Zhou and P. D. Lund, “Peer-to-peer energy sharing and trading of renewable energy in smart communities—Trading pricing models, decision-making and agent-based collaboration,” Renew. Energy, vol. 207, pp. 177–193, 2023. doi: 10.1016/j.renene.2023.02.125. [Google Scholar] [CrossRef]
Cite This Article
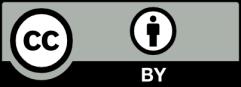
This work is licensed under a Creative Commons Attribution 4.0 International License , which permits unrestricted use, distribution, and reproduction in any medium, provided the original work is properly cited.