Open Access
ARTICLE
Synergizing Wind, Solar, and Biomass Power: Ranking Analysis of Off-Grid System for Different Weather Conditions of Iran
Energy and Environment Research Center, Shahrekord Branch, Islamic Azad University, Shahrekord, Iran
* Corresponding Author: Mehdi Jahangiri. Email:
Energy Engineering 2024, 121(6), 1381-1401. https://doi.org/10.32604/ee.2024.050029
Received 25 January 2024; Accepted 10 April 2024; Issue published 21 May 2024
Abstract
Nowadays, the use of renewable energies, especially wind, solar, and biomass, is essential as an effective solution to address global environmental and economic challenges. Therefore, the current study examines the energy-economic-environmental analysis of off-grid electricity generation systems using solar panels, wind turbines, and biomass generators in various weather conditions in Iran. Simulations over 25 years were conducted using HOMER v2.81 software, aiming to determine the potential of each region and find the lowest cost of electricity production per kWh. In the end, to identify the most suitable location, the Technique for Order Preference by Similarity to Ideal Solution (TOPSIS) method was employed to rank different stations based on simulation output parameters and some other influential factors. Considering the evaluation of various parameters, the stations in Yazd, Marand, and Dezful achieved the best results, while the stations in Ramsar, Shahrekord, and Gonbad presented the least favorable outcomes. In Yazd, the wind turbine is an economic priority, and a 100 kW wind turbine is utilized in the optimal system. In Yazd, where the simultaneous use of renewable energies is most prominent, the lowest pollutant production occurred with a quantity of 1174 kg/year. Annual energy losses are highest in Jask station and lowest in Yazd.Graphic Abstract
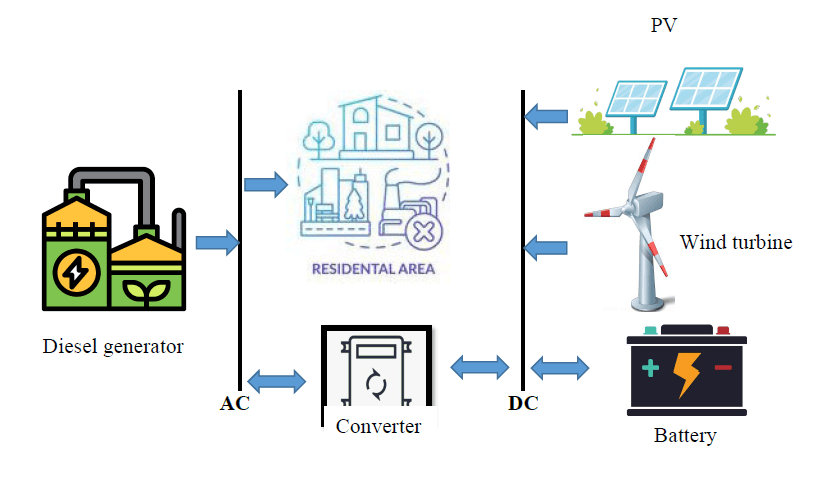
Keywords
The simultaneous use of renewable energies, biomass, wind, and solar, holds significant importance and necessity for residential electricity supply in various climates across Iran [1]. Given the country’s climatic diversity, leveraging these energies can contribute to improving residential electricity provision and reducing dependence on fossil fuels [2]. In the southern regions, characterized by scattered sunlight and strong wind resources, the installation of solar panels and wind turbines can aid in producing stable and clean electricity [3]. Additionally, in the northern areas abundant in biomass resources, utilizing biogas and biomass can contribute to electricity generation [4]. The use of these energies in residential electricity production not only reduces air pollution and greenhouse gas emissions but also helps decrease reliance on fossil fuels and lowers energy costs [5]. Furthermore, due to the independence of these energies from the power grid, residential electricity supply is guaranteed and stable even in the event of a grid outage [6].
Bioenergy is the largest renewable energy source, accounting for 12.6% of the total energy consumption in 2020. Globally, the predominant use of bioenergy has been for heating. In 2022, 672 TWh of electricity were generated from a wide range of biomass feedstocks, constituting 24% of the total electricity production. The total installed bioenergy capacity was 149 GW in 2022 (Fig. 1) [7].
Figure 1: Global electricity generation capacity from biomass for each region worldwide [7]
With the addition of 243 GW of new facilities, the solar PV market continued its steady growth in 2022, reaching a total capacity of 1185 GW (Fig. 2) [7]. In 2022, global solar production averaged 6.2%, compared to 5% in 2021 [8]. For the tenth consecutive year, Asia dominated in new photovoltaic solar installations, claiming 64% of the added global capacity in 2022. Leading countries in cumulative installed PV solar capacity remained China, the United States, India, Brazil, and Spain. While solar PV panel production remains concentrated in China, many countries have strengthened import barriers and incentives for domestic production, led by initiatives in the United States and India [7].
Figure 2: Global electricity generation capacity from solar cells worldwide [7]
In 2022, more than 77 GW of wind energy were added to global grids, bringing the total grid-connected capacity to 906 GW (Fig. 3) [7]. The global increase in wind power grid connection decreased by more than 17%, primarily due to reduced speeds in China and the United States. Europe was the only region where an increase in this matter was observed. Countries worldwide increased their wind energy goals due to climate change, energy security, economic growth objectives, and the competitiveness of wind energy [9].
Figure 3: Global electricity generation capacity from wind turbines worldwide [7]
Table 1 evaluates recent studies in the field of wind, solar, and biomass energy use, with a focus on Iran. The purpose of presenting Table 1 is to clearly articulate the existing scientific gap, in addition to providing a brief overview of the work and areas of investigation conducted by others. The aim is to highlight the distinctiveness of the current study in comparison to the work of others.
1.5 Innovation of the Current Work
As evident in Table 1 and based on the information provided by the authors of the present study, selected stations in eight diverse climates of Iran have not been previously investigated in the context of electricity generation. Previous studies either had different scales, employed different methods, or focused on different locations and objectives. In this study, for the first time, the performance of an off-grid renewable electricity generation system, utilizing wind, solar, and biomass, was examined at eight selected stations in Iran. A 25-year simulation was conducted using HOMER v2.81 software to not only serve as a roadmap for decision-makers in the renewable energy sector but also provide a suitable estimation for potential investors. To identify the best station, analytical analyses were performed using the TOPSIS method with eight independent parameters, including energy, environmental, economic, population, and natural disaster risk analyses. The most suitable station was then selected based on these criteria.
In Fig. 4, the locations of Iran in the world and the positions of the studied stations are indicated on the map of Iran [20]. It also shows the climate zone to which each station belongs. Other climate-related information required for simulation is provided in Table 2, including geographical longitude and latitude, annual average of wind, solar, and biomass, annual average air clearness index, and elevation above sea level.
Figure 4: Location of the studied stations on the map of Iran [20]
The available biomass, extracted from reference [15], is divided by the ratio of urban population to the total population of the province. This is because the biomass value cited in reference [15] pertains to the entire province, not just one city.
3.1 Energy-Economic-Environmental Analysis
In Fig. 5, the diagram of the relevant data for assessing solar, wind, and biomass power using the HOMER software is presented. The software outputs are also described. HOMER software, designed by the National Renewable Energy Laboratory of the United States, is tasked with analyzing and optimizing renewable hybrid systems, providing rankings based on financial analysis [21,22].
Figure 5: Diagram of input and output parameters of HOMER software
Table 3 presents the equations governing the HOMER software, including solar cell power, biogas generator efficiency, battery performance equation, and economic calculations.
Various multi-criteria decision-making (MCDM) methods, such as TOPSIS, were employed to prioritize the examined panels. The formulas that guide the TOPSIS approach are outlined by previous studies [30].
Step 1: Calculation of Normalized Matrix.
Step 2: Calculation of weighted Normalized Matrix.
Step 3: Computing the ideal best and ideal worst values, denoted as “V+” and “V–” for non-beneficial criteria (minimum and maximum of Vij), and “V–” and “V+” for beneficial criteria (minimum and maximum of Vij).
Step 4: Calculation of the Euclidean distance from the ideal best and ideal worst.
Step 5: Calculation of Performance Score.
The schematic diagram of the system under investigation is presented in Fig. 6. For backup power in emergency conditions, a combination of batteries and a biomass generator has been utilized. The objective is to supply electricity to an area with 100 households [31].
Figure 6: Schematic diagram of the system under investigation
Table 4 presents the simulation data, which includes pricing and technical information on the equipment used in the study.
Other necessary simulation data include the price of each ton of biomass equal to $18 [35], the project’s lifetime of 25 years [36], and an annual real interest rate of 18% [37]. Penalties for pollutants including CO2, CO, SO2, and NOx are considered in the simulations with respective values of $3.1, $57, $560, and $184 per ton [35]. Fig. 7 illustrates the most crucial simulation data, i.e., the required electricity, with an average annual electricity demand of 1488 kWh/day. To account for random variations, a 15% coefficient is applied for daily changes and a 20% coefficient for hourly variations.
Figure 7: The electricity consumption profile throughout the year (upper chart) and over 24 h (lower chart)
Regarding the validation of the present work’s results, it should be noted that the accuracy of the software results has been previously assessed by the National Renewable Energy Laboratory (NREL) of the United States, showing good agreement between simulation results and experimental data [38]. References [39–41] also indicate that the maximum simulation error with HOMER software compared to experimental results is below 10%. Furthermore, HOMER software results for reproducibility require precise input data such as annual electricity load profiles, monthly average solar radiation profiles, monthly average wind speed profiles, and random variability coefficients, which can be challenging to obtain. These types of data are not typically included in the published articles.
The number of simulations performed for each station, considering the number of size taken into account for each of the components, is equal to 226,270 (10 cases for PV, 11 cases for wind turbine, 11 cases for battery, 17 cases for electric converter, and 11 cases for biomass generator), with an average CPU time of 20 min for eight stations under investigation.
Table 5 presents the simulation results for the studied stations in various climates across Iran. The results indicate that in all scenarios, the economical priority is given to the 500 kW biomass generator. The highest and lowest usage of solar panels is observed in Jask (550 kW) and Yazd (300 kW), respectively. Additionally, only in the Yazd station, the economic optimum includes the use of a wind turbine, with a 100 kW wind turbine being employed in the optimal system.
The price per kWh of generated electricity for the examined system varies between 0.615 $/kWh (Jask) to 0.660 $/kWh (Ramsar). The average electricity generation cost for the studied stations is 0.643 $/kWh. Considering that Yazd has the highest simultaneous utilization of various renewable energies (wind turbines and solar panels), it exhibits the lowest pollutant production with 1174 kg/year. In contrast, Ramsar has the highest pollutant production, reaching 1446 kg/year.
Based on the results, it can be generally stated that the higher the electricity generation cost, the lower the corresponding amount of wind and solar power. This is evident as the highest utilization of electricity from biomass is observed in Ramsar with a quantity of 534,217 kWh/year, corresponding to the most expensive electricity generation.
Additionally, the highest solar power production is 1,066,504 kWh/year in Jask, which has the least expensive electricity production. The simultaneous use of wind and solar, resulting in a total annual electricity production of 650,868 kWh, compensates for the weaknesses of wind and solar energies, leading to the lowest biomass electricity usage in Yazd with a quantity of 433,434 kWh/year.
Regarding annual energy losses, Jask, which has the highest renewable DC power production, is in the first rank. An interesting point about Yazd is that it has the lowest energy losses, with a quantity of 44,796 kWh/year. This indicates that wind and solar energies are used when they can compensate for each other’s shortages and deficiencies. Consequently, there is less stored electricity, resulting in fewer losses.
Another point from Table 5 is regarding surplus electricity production, which can generate significant income through selling to the grid or exporting to neighboring countries. However, the authors of the present study suggest converting this surplus electricity into hydrogen for long-term usability. The highest and lowest annual surplus electricity production levels are 914,336 kWh (Jask) and 486,936 kWh (Marand), respectively. As renewable DC power production decreases, surplus electricity production also decreases. In other words, the highest and lowest renewable DC power production correspondingly results in the highest and lowest surplus electricity production.
In Fig. 8, the weights and types of the parameters under consideration are presented. To calculate the weights in Fig. 8, the average opinions of 10 experts were utilized, and then the weights were normalized and presented in Fig. 8. Normalization has been performed in such a way that the size of each parameter is divided by the square root of the sum of the squares of the parameters. Out of the 8 parameters under investigation, 5 are simulation output parameters, and 3 are influential parameters in the problem (land price, population, and risk index), which have been added for final decision-making. These three parameters are influential as they were not applicable in the simulations conducted by the HOMER software but have a significant impact on the performance and the decision-making process of investors in selecting the superior station. The parameters LCOE, Emission, Land price, and Population carry the highest weights, while Excess electricity and Losses have the lowest weights. Non-Beneficial parameters are those that, the higher they are, have a more negative impact, whereas Beneficial parameters are those that, the higher they are, have a more positive impact. Out of the 8 parameters under consideration, LCOE, Emissions, Losses, Land price, and Risk index are considered Non-Beneficial, and the rest are Beneficial. The weights of the parameters are highly effective in calculating the rank of each station as multiplying by the value of that parameter leads to scoring and improving the rank of that station.
Figure 8: Weights and types of parameters considered for ranking
In Table 6, using Eqs. (1) and (2), the normalized matrix for the 8 parameters under consideration, considering the weight of each parameter, is formed. This matrix is used to calculate the parameters V+ and V− (Step 3 of Section 3.2). It should be noted that in the calculation of parameters V+ and V− in Table 7, the type of parameter under consideration (Beneficial or Non-Beneficial) should be taken into account.
In Table 8, using Eqs. (3) and (4), the parameters Si+ and Si− have been calculated. Then, with the use of Eq. (5), the final score for each of the investigated stations has been calculated. According to the results in Table 8, it is evident that Yazd, Marand, and Dezful are the top-performing stations, while Ramsar, Shahrekord, and Gonbad are the least suitable stations, respectively. An interesting observation is that, despite Jask having the lowest cost of electricity production, it does not rank among the top three stations. This can be attributed to the high electricity losses in Jask. The choices of Yazd and Ramsar were predictable since Yazd excelled in terms of pollutant emissions, losses, and surplus electricity production, while Ramsar performed poorly in pollutant emissions and the price of each kWh of energy.
Fig. 9 provides an energy analysis for the most critical day of the year, January 30, when using the wind-solar-biomass system in Yazd, the most suitable station. The critical day refers to the day with the highest electricity demand, which, based on a detailed examination and in accordance with Fig. 7, is in January, specifically the thirtieth day. The results in Fig. 9 indicate that the maximum battery charging occurs when the biomass generator comes into operation, primarily during nighttime. It is noteworthy that during the most critical time of the year when 170 kW of electricity is required, the biomass generator and PV produce approximately 200 and 270 kW of electricity respectively at their maximum capacity. At the same time, the wind turbine generates around 90 kW of electricity. According to the findings in Fig. 9 and, as observed in Table 5, wind power has the lowest contribution, followed by biomass and solar power. An important note is the significant and uniform demand for biomass power on critical days throughout the year. According to the results in Fig. 9, the maximum battery charge on critical days throughout the year ranges between 80%–90%.
Figure 9: Energy analysis for the most critical day of the year (January 30) when using the optimal scenario in the city of Yazd
– Since the current work is on a residential scale and in an off-grid scenario, it is suggested that in future works, other scales such as industrial and transportation be investigated, and also the impact of grid connection be studied [42,43].
– Performing sensitivity analysis on influential parameters is another task that can be done in the continuation of the current work [42].
– Due to the greater importance of CAPEX compared to OPEX in Iran due to the lower OPEX costs in Iran, in the present work, the LCOE parameter was selected for ranking. It is recommended that in future works, the OPEX parameter also be considered in ranking [44].
– Since the TOPSIS method was used for ranking in the current work, it is suggested that in future works, other ranking methods such as WASPAS, GRA, etc., be utilized [45].
– Due to the lack of use of solar trackers and not considering the effect of temperature on the performance of PVs in the current work, it is recommended that these aspects be taken into account in future studies and their impact be assessed.
– To diversify renewable energy sources in Iran, it is recommended to incorporate hydropower alongside other types of renewable energy in future endeavors [42,46].
Finding a system capable of providing sustainable energy under different weather conditions and acting as a self-sufficient system is crucial in a country like Iran with high climatic diversity. This study investigates a solar, wind, and biomass system for electricity generation in eight different climates of Iran using HOMER v2.81 software. The system is designed for use in areas not connected to the power grid. After conducting energy-economic-environmental analyses and obtaining five influential output parameters, a decision-making matrix is formed using three other influential parameters (land price, population, and risk index). Finally, the results are ranked using the TOPSIS method, determining the top-performing stations. The key findings of this study include:
– The use of a 500 kW biomass generator is prioritized as an economic choice in all scenarios.
– The highest and lowest usage of solar cells is observed in Jask (550 kW) and Yazd (300 kW), respectively.
– Only in Yazd is the wind turbine considered an economic priority and a 100 kW wind turbine is used in the optimal system.
– The price of electricity varies between $0.615/kWh (Jask) and $0.660/kWh (Ramsar).
– In Yazd, where the most simultaneous use of renewable energies occurs, the lowest pollutant production of 1174 kg/year is observed, with the highest being 1446 kg/year in Ramsar.
– Annual energy losses in Jask (with the highest renewable DC power generation) rank first, while Yazd has the lowest annual energy losses.
– Yazd, Marand, and Dezful are the top-performing stations, while Ramsar, Shahrekord, and Gonbad are the least suitable.
– Weighting influential parameters using the average opinions of 10 experts showed that LCOE, Emission, Land price, and Population have the highest weights.
– Energy losses in Jask are very high, potentially impacting the final decision regarding the superiority of this station.
Acknowledgement: I would like to extend my heartfelt appreciation to the anonymous peer reviewers who generously dedicated their time and expertise to review and provide constructive feedback on this research paper. Their insightful comments and suggestions have significantly enhanced the quality and rigor of this paper.
Funding Statement: The authors received no specific funding for this study.
Author Contributions: The authors confirm contribution to the paper as follows: Study conception and design: R. Keshavarzi, M. Jahangiri; Data collection: R. Keshavarzi; Analysis and interpretation of results: M. Jahangiri; Draft manuscript preparation: R. Keshavarzi, M. Jahangiri. All authors reviewed the results and approved the final version of the manuscript.
Availability of Data and Materials: The authors confirm that the data supporting the findings of this study are available within the article.
Conflicts of Interest: The authors declare that they have no conflicts of interest to report regarding the present study.
References
1. Razeghi, M., Hajinezhad, A., Naseri, A., Noorollahi, Y., Moosavian, S. F. (2023). An overview of renewable energy technologies for the simultaneous production of high-performance power and heat. Future Energy, 2(2), 1–11. https://doi.org/10.55670/fpll.fuen.2.2.1 [Google Scholar] [CrossRef]
2. Solaymani, S. (2021). A review on energy and renewable energy policies in Iran. Sustainability, 13(13), 7328. https://doi.org/10.3390/su13137328 [Google Scholar] [CrossRef]
3. Shahbazi, R., Kouravand, S., Hassan-Beygi, R. (2023). Analysis of wind turbine usage in greenhouses: Wind resource assessment, distributed generation of electricity and environmental protection. Energy Sources, Part A: Recovery, Utilization, and Environmental Effects, 45(3), 7846–7866. https://doi.org/10.1080/15567036.2019.1677810 [Google Scholar] [CrossRef]
4. Mostafaeipour, A., Sedaghat, A., Kasaeian, A., Hedayatpour, M, Jahangiri, M., (2020). Location planning for production of bioethanol fuel from agricultural residues in the south of Caspian Sea. Environmental Development, 33, 100500. https://doi.org/10.1016/j.envdev.2020.100500 [Google Scholar] [CrossRef]
5. Kumi, E. N., Mahama, M. (2023). Greenhouse gas (GHG) emissions reduction in the electricity sector: Implications of increasing renewable energy penetration in Ghana’s electricity generation mix. Scientific African, 21, e01843. https://doi.org/10.1016/j.sciaf.2023.e01843 [Google Scholar] [CrossRef]
6. Dos Santos, M. E. M., Castro, R., Costa, H. (2023). Contradictory electric energy policies and its impacts on the renewability of the electric matrix: Brazil as a case study. Heliyon, 9(10), e19309. https://doi.org/10.1016/j.heliyon.2023.e19309 [Google Scholar] [PubMed] [CrossRef]
7. REN21 (2023). Renewables 2023 global status report collection, renewables in energy supply. Paris: REN21 Secretariat. [Google Scholar]
8. Pourasl, H. H., Barenji, R. V., Khojastehnezhad, V. M. (2023). Solar energy status in the world: A comprehensive review. Energy Reports, 10, 3474–3493. https://doi.org/10.1016/j.egyr.2023.10.022 [Google Scholar] [CrossRef]
9. Li, L., Zhu, C., Zou, X., Hu, J., Kang, Y. et al. (2023). Review of frequency regulation requirements for wind power plants in international grid codes. Renewable and Sustainable Energy Reviews, 187, 113731. https://doi.org/10.1016/j.rser.2023.113731 [Google Scholar] [CrossRef]
10. Vahdatpour, S., Behzadfar, S., Siampour, L., Veisi, E., Jahangiri, M. (2017). Evaluation of off-grid hybrid renewable systems in the four climate regions of Iran. Journal of Renewable Energy and Environment, 4(1), 61–70. https://doi.org/10.30501/jree.2017.70107 [Google Scholar] [CrossRef]
11. Aghapouramin, K. (2020). Technical, economical, and environmental feasibility of hybrid renewable electrification systems for off-grid remote rural electrification areas for East Azerbaijan Province, Iran. Technology and Economics of Smart Grids and Sustainable Energy, 5(1), 20. https://doi.org/10.1007/s40866-020-00093-5 [Google Scholar] [CrossRef]
12. Jahangir, M. H., Cheraghi, R. (2020). Economic and environmental assessment of solar-wind-biomass hybrid renewable energy system supplying rural settlement load. Sustainable Energy Technologies and Assessments, 42, 100895. https://doi.org/10.1016/j.seta.2020.100895 [Google Scholar] [CrossRef]
13. Shorabeh, S. N., Argany, M., Rabiei, J., Firozjaei, H. K., Nematollahi, O. (2021). Potential assessment of multi-renewable energy farms establishment using spatial multi-criteria decision analysis: A case study and mapping in Iran. Journal of Cleaner Production, 295, 126318. https://doi.org/10.1016/j.jclepro.2021.126318 [Google Scholar] [CrossRef]
14. Jahangiri, M., Karimi Shahmarvandi, F., Alayi, R. (2021). Renewable energy-based systems on a residential scale in southern coastal areas of Iran: Trigeneration of heat, power, and hydrogen. Journal of Renewable Energy and Environment, 8(4), 67–76. https://doi.org/10.30501/jree.2021.261980.1170 [Google Scholar] [CrossRef]
15. Dehkordi, M. H. R., Isfahani, A. H. M., Rasti, E., Nosouhi, R., Akbari, M. et al. (2022). Energy-economic-environmental assessment of solar-wind-biomass systems for finding the best areas in Iran: A case study using GIS maps. Sustainable Energy Technologies and Assessments, 53, 102652. https://doi.org/10.1016/j.seta.2022.102652 [Google Scholar] [CrossRef]
16. Mohseni, M., Moosavian, S. F., Hajinezhad, A. (2022). Feasibility evaluation of an off-grid solar-biomass system for remote area electrification considering various economic factors. Energy Science & Engineering, 10(8), 3091–3107. https://doi.org/10.1002/ese3.1202 [Google Scholar] [CrossRef]
17. Tehrani, M. M., Akhtari, M., Kasaeian, A., Rad, M. A. V., Toopshekan, A. et al. (2023). Techno-economic investigation of a hybrid biomass renewable energy system to achieve the goals of SDG-17 in deprived areas of Iran. Energy Conversion and Management, 291, 117319. https://doi.org/10.1016/j.enconman.2023.117319 [Google Scholar] [CrossRef]
18. Jahangir, M. H., Bazdar, E., Kargarzadeh, A. (2022). Techno-economic and environmental assessment of low carbon hybrid renewable electric systems for urban energy planning: Tehran-Iran. City and Environment Interactions, 16, 100085. https://doi.org/10.1016/j.cacint.2022.100085 [Google Scholar] [CrossRef]
19. Mousavi, S. A., Toopshekan, A., Mehrpooya, M., Delpisheh, M. (2023). Comprehensive exergetic performance assessment and techno-financial optimization of off-grid hybrid renewable configurations with various dispatch strategies and solar tracking systems. Renewable Energy, 210, 40–63. https://doi.org/10.1016/j.renene.2023.04.018 [Google Scholar] [CrossRef]
20. Jahangiri, M., Yousefi, Y., Pishkar, I., Hosseini Dehshiri, S. J., Hosseini Dehshiri, S. S. et al. (2023). Techno-econo–enviro energy analysis, ranking and optimization of various building-integrated photovoltaic (BIPV) types in different climatic regions of Iran. Energies, 16(1), 546. https://doi.org/10.3390/en16010546 [Google Scholar] [CrossRef]
21. Boruah, D., Chandel, S. S. (2024). Techno-economic feasibility analysis of a commercial grid-connected photovoltaic plant with battery energy storage-achieving a net zero energy system. Journal of Energy Storage, 77, 109984. https://doi.org/10.1016/j.est.2023.109984 [Google Scholar] [CrossRef]
22. Kumar, P. H., Gopi, R. R., Rajarajan, R., Vaishali, N. B., Vasavi, K. et al. (2024). Prefeasibility techno-economic analysis of hybrid renewable energy system. e-Prime-Advances in Electrical Engineering, Electronics and Energy, 7, 100443. https://doi.org/10.1016/j.prime.2024.100443 [Google Scholar] [CrossRef]
23. Ennemiri, N., Berrada, A., Emrani, A., Abdelmajid, J., Mrabet, R. El (2024). Optimization of an off-grid PV/biogas/battery hybrid energy system for electrification: A case study in a commercial platform in Morocco. Energy Conversion and Management: X, 21, 100508. https://doi.org/10.1016/j.ecmx.2023.100508 [Google Scholar] [CrossRef]
24. Alavi, S. M. S., Maleki, A., Noroozian, A., Khaleghi, A. (2024). Simultaneous optimal site selection and sizing of a grid-independent hybrid wind/hydrogen system using a hybrid optimization method based on ELECTRE: A case study in Iran. International Journal of Hydrogen Energy, 55, 970–983. https://doi.org/10.1016/j.ijhydene.2023.11.110 [Google Scholar] [CrossRef]
25. Modu, B., Abdullah, M. P., Bukar, A. L., Hamza, M. F., Adewolu, M. S. (2024). Operational strategy and capacity optimization of standalone solar-wind-biomass-fuel cell energy system using hybrid LF-SSA algorithms. International Journal of Hydrogen Energy, 50, 92–106. https://doi.org/10.1016/j.ijhydene.2023.07.215 [Google Scholar] [CrossRef]
26. Terkes, M., Arikan, O., Gokalp, E. (2024). The effect of electric vehicle charging demand variability on optimal hybrid power systems with second-life lithium-ion or fresh Na-S batteries considering power quality. Energy, 288, 129760. https://doi.org/10.1016/j.energy.2023.129760 [Google Scholar] [CrossRef]
27. Aboutalebi, G. R., Khalili, M., Jahangiri, M. (2023). Effect of temperature coefficient and efficiency of PV technologies on 3E performance and hydrogen production of on-grid PV system in a very hot and humid climate. Journal of Solar Energy Research, 8(4), 1715–1727. https://doi.org/10.22059/jser.2024.362287.1326 [Google Scholar] [CrossRef]
28. Abdolmaleki, L., Berardi, U. (2024). Hybrid solar energy systems with hydrogen and electrical energy storage for a single house and a midrise apartment in North America. International Journal of Hydrogen Energy, 52, 1381–1394. https://doi.org/10.1016/j.ijhydene.2023.11.222 [Google Scholar] [CrossRef]
29. Pamuk, N. (2024). Techno-economic feasibility analysis of grid configuration sizing for hybrid renewable energy system in Turkey using different optimization techniques. Ain Shams Engineering Journal, 15(3), 102474. https://doi.org/10.1016/j.asej.2023.102474 [Google Scholar] [CrossRef]
30. Ali, G., Musbah, H. N., Aly, H. H., Little, T. (2023). Hybrid renewable energy resources selection based on multi criteria decision methods for optimal performance. IEEE Access, 11, 26773–26784. https://doi.org/10.1109/ACCESS.2023.3254532 [Google Scholar] [CrossRef]
31. Jahangiri, M., Mostafaeipour, A., Rahman Habib, H. U., Saghaei, H., Waqar, A. (2021). Effect of emission penalty and annual interest rate on cogeneration of electricity, heat, and hydrogen in Karachi: 3E assessment and sensitivity analysis. Journal of Engineering, 2021, 6679358. https://doi.org/10.1155/2021/6679358 [Google Scholar] [CrossRef]
32. Liu, J., Jian, L., Wang, W., Qiu, Z., Zhang, J. et al. (2021). The role of energy storage systems in resilience enhancement of health care centers with critical loads. Journal of Energy Storage, 33, 102086. https://doi.org/10.1016/j.est.2020.102086 [Google Scholar] [CrossRef]
33. Pishkar, I., Mirzakhani, A. (2022). Energetic, economic and environmental (3E) evaluation of grid-connected wind-powered electric vehicle (EV) charging station: Effect of wind turbine type. International Journal of Smart Electrical Engineering, 11(4), 245–255. https://doi.org/10.30495/ijsee.2022.1955652.1190 [Google Scholar] [CrossRef]
34. Valikhani Dehaghani, M., Khalili Samani, M., Mohamadi Janaki, D. (2022). Management and environmental assessment of simultaneous production of solar electricity and heat (Case Study: Sar Agha Seyed Rural Health Center). International Journal of Smart Electrical Engineering, 11(4), 191–203. https://doi.org/10.30495/ijsee.2022.1957192.1195 [Google Scholar] [CrossRef]
35. Jahangiri, M., Khorsand Dehkordi, M., Khorsand Dehkordi, S. (2023). Potential measurement of electricity supply. International Journal of Low-Carbon Technologies, 18, 1067–1076. https://doi.org/10.1093/ijlct/ctac072 [Google Scholar] [CrossRef]
36. Das, D., Chakraborty, I., Bohre, A. K., Kumar, P., Agarwala, R. (2024). Sustainable integration of green hydrogen in renewable energy systems for residential and EV applications. International Journal of Energy Research, 2024, 8258624. https://doi.org/10.1155/2024/8258624 [Google Scholar] [CrossRef]
37. Pishkar, I., Beigi, S. M. (2024). A comprehensive evaluation of renewable energy systems with a backup to provide a part of electrical needs of a hospital: A case study in grid-connected mode. Iranica Journal of Energy & Environment, 15(1), 1–9. https://doi.org/10.5829/ijee.2024.15.01.01 [Google Scholar] [CrossRef]
38. Das, B., Prasad, G. S., Saibabu, C., Sadangi, S. P. (2019). Validating simulation result of solar hybrid system for domestic consumers using HOMER software. International Journal of Innovative Technology and Exploring Engineering, 8(11), 1428–1431. https://doi.org/10.35940/ijitee.J9772.0981119 [Google Scholar] [CrossRef]
39. Alhousni, F. K., Alnaimi, F. B. I., Okonkwo, P. C., Belgacem, I. Ben, Mohamed, H. et al. (2023). Photovoltaic power prediction using analytical models and homer-pro: Investigation of results reliability. Sustainability, 15(11), 8904. https://doi.org/10.3390/su15118904 [Google Scholar] [CrossRef]
40. Roberts, J. J., Cassula, A. M., Junior, J. F., Prado, P. O. (2015). Simulation and validation of photovoltaic system performance models. XI Latin-American Congress on Electricity Generation and Transmission, São José dos Campos, São Paulo, Brazil, pp. 1–9. [Google Scholar]
41. Hoseinzadeh, S., Groppi, D., Nastasi, B., Bruschi, D., Garcia, D. A. (2024). Designing high-share 50% and 100% renewable energy scenarios for Ragusa by sustainable energy toolkit application. Sustainable Energy Technologies and Assessments, 63, 103645. https://doi.org/10.1016/j.seta.2024.103645 [Google Scholar] [CrossRef]
42. Hoseinzadeh, S., Garcia, D. A., Huang, L. (2023). Grid-connected renewable energy systems flexibility in Norway islands’ Decarbonization. Renewable and Sustainable Energy Reviews, 185, 113658. https://doi.org/10.1016/j.rser.2023.113658 [Google Scholar] [CrossRef]
43. Avezova, N. R., Khaitmukhamedov, A. E., Usmanov, A. Y., Boliyev, B. B. (2017). Solar thermal power plants in the world: The experience of development and operation. Applied Solar Energy, 53(1), 72–77. https://doi.org/10.3103/S0003701X17010030 [Google Scholar] [CrossRef]
44. Hill, S. J. P., Bamisile, O., Hatton, L., Staffell, I., Jansen, M. (2024). The cost of clean hydrogen from offshore wind and electrolysis. Journal of Cleaner Production, 445, pp. 1141162. https://doi.org/10.1016/j.jclepro.2024.141162 [Google Scholar] [CrossRef]
45. Shahgholian, S., Taheri, M., Jahangiri, M. (2023). Investigating the cost-effectiveness of solar electricity compared to grid electricity in the capitals of middle eastern countries: A residential scale case study. International Journal of Photoenergy, 8028307. https://doi.org/10.1155/2023/8028307 [Google Scholar] [CrossRef]
46. Kefif, N., Melzi, B., Hashemian, M., Assad, M. E. H., Hoseinzadeh, S. (2022). Feasibility and optimal operation of micro energy hybrid system (hydro/wind) in the rural valley region. International Journal of Low-Carbon Technologies, 17, 58–68. https://doi.org/10.1093/ijlct/ctab081 [Google Scholar] [CrossRef]
Cite This Article
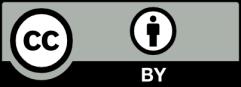
This work is licensed under a Creative Commons Attribution 4.0 International License , which permits unrestricted use, distribution, and reproduction in any medium, provided the original work is properly cited.