Open Access
ARTICLE
Techno-Economic Optimization of Novel Stand-Alone Renewable Based Electric Vehicle Charging Station near Bahria Town Karachi, Sindh Pakistan
1 Department of Electrical Engineering, Mehran University of Engineering and Technology, Jamshoro, Pakistan
2 Department of Mechanical Engineering, Mehran University of Engineering and Technology, Jamshoro, Pakistan
* Corresponding Author: Laveet Kumar. Email:
Energy Engineering 2024, 121(6), 1439-1457. https://doi.org/10.32604/ee.2024.049977
Received 24 January 2024; Accepted 18 April 2024; Issue published 21 May 2024
Abstract
Electric vehicles (EVs) are the most interesting and innovative technology in the 21st century because of their enormous advantages, both technically and economically. Their emissions rate compared to fuel-based vehicles is negligible as they do not consume fuel and hence do not emit any harmful gases. However, their bulk production, adoption and lack of charging stations increase the stress of power stations due to modern-day lifestyles. If Electric vehicles demand increases drastically then conventional power stations will not bear their demand and if they generate electricity by conventional means it will be very costly and may further add greenhouse gases. Therefore, this research provides the techno-economic assessment of a stand-alone renewable-dependent electric vehicle charging station, excluding any burden on electrical utility. The proposed study is carried out in Bahria Town, Karachi, a city in Pakistan. In this study, HOMER Pro software was utilized for techno-economic assessment. A hybrid system comprising solar Photovoltaic/Wind Turbine/Fuel cells and battery storage is included in the model. Solar and wind resources were taken from NASA’s website, where charging stations will be integrated. The overall results show promising in terms of total Net Present Cost and the Cost of Energy which are 2.72M $ and 0.237 $, respectively. The total system generation is 3,598 Megawatt hours per year, and the total energy consumption is 885 Megawatt hours per year.Keywords
Nomenclature
NPC | Net present cost |
COE | Cost of energy |
WT | Wind turbine |
FC | Fuel cell |
CC | Charging cycle |
GHI | Global horizontal irradiance |
PVS | Photovoltaic system |
OP | Operating cost |
COH | Cost of hydrogen |
Today, electrical energy is a vital commodity. In many regions across the globe, the energy is obtained from fossil fuel sources. The conventional sources of obtaining energy have low energy conversion efficiency. The energy-balanced equation must be satisfied. The non-conventional resources of energy are an important aspect of balancing energy supply and demand. The supply and demand side management process can be a key part of energy balance. In the same way, electric vehicles (EVs) have many pros and can be crucial for supply and demand side management and will obviously help reduce the energy demand when they act as vehicle-to-grid technology. Electric vehicles nowadays have huge impacts over fuel-based vehicles for many reasons, including zero or negligible emissions, low cost, more efficient, reliable operation, and negligible maintenance costs [1,2]. It is worthwhile to note that by 2030, the sales of EVs will increase by 230 million units [3]. With such a huge surge, it is necessary to have electric charging stations everywhere therefore people can travel without any difficulty while charging their vehicles. On the other hand, this is also important that less electricity should be fetched from the grid, otherwise, it will be very difficult for grid operators to manage the increased amount of load and supply EV charging stations [4]. However, if they manage to do so, it will be very costly to charge the EVs. That is why it is necessary to install standalone renewable-based charging stations. This very concept will be very cost-effective, environmentally friendly, and easy to maintain. Considering the cost of energy, rate of NOx and SOx emissions, natural replenishment of fossil fuels, and lower efficiency of conventional plants, these EVs will eliminate all these factors efficiently when they are charged with non-conventional energy resources [5]. Renewable energy resources can beat non-conventional energy resources when they are used cumulatively with others, like a combination of solar, wind, batteries, and hydrogen storage systems. However, if they are not used collectively, they cannot manage huge power demand and will be very costly due to their variable nature, like solar and wind. Therefore, the hybridization of these renewable energy resources will be very economical, efficient, and more reliable than non-renewable energy resources [6]. Renewable energy resources depend on renewable sources like wind power and solar irradiance, which vary from time to time and location to location [7]. Pakistan is an under-developed country and manages 60% of its energy needs from fossil fuel-based energy resources [7]. Pakistan’s anticipated load up to 2030 will be 36,369 MW [7,8] and its generating capacity lies between 18,000 MW and 20,000 MW, so there is a deficiency of 5,000 MW to 7,000 MW of power [7,9], thus it will be beneficial for Pakistan to adopt and switch from non-renewable energy resources to renewable energy resources. The ADB (Asian Development Bank) has suggested that switching Pakistan’s remote areas to renewable energy resources can be a financial stability solution [7,10]. However, due to the unpredictable nature of solar irradiance, highest in daytime and zero in nighttime appeals some storage techniques must be employed, like batteries, hydrogen energy storage, and flywheels, with the combination of Battery Energy Storage and solar PV loss of power supply possibility at day and night can be reduced [7,11,12]. Considering the longer durability and cost value compared with lithium or sodium batteries hydrogen storage is a promising solution. Hydrogen produced by the electrolyzer during the day is accumulated in a hydrogen tank and used as fuel for fuel cells to overcome energy demand at night time [7,13]. Considering the above problems this research aim is to model the hybrid standalone energy system for EV Charging considering their techno-economic evaluations.
Nowadays Hydrogen is considered a renewable fuel source and can easily be obtained by different methods like steam methane reforming, coal gasification, partial oxidation of hydrocarbons, biomass gasification, thermochemical process, photochemical process, photo biological process, and electrolysis of water [14]. Many researchers have worked on renewable-based charging stations considering different sources like solar, wind, hydrogen, and biomass. For instance, the research conducted by [15] used the HOMER Pro software to design and optimize the wind-solar based hybrid energy charging station. The results revealed 44.4% generation from hybrid sources whereas 55% the electricity generation from solar energy alone. Cumulatively the model was contributing 843,150 kWh with 0.064 $/kWh production cost from hybrid source. Liu et al. concluded that EVs and distributed renewable resources can be cost-efficient compared to grid supply in the short term while vehicle to grid in the long term [16]. A solar recharging station was installed in Chile to overcome the CO2 emissions via fuel-based vehicles and improve the financial crisis by adopting these cost-efficient and reliable EVs [17]. Implementation of these renewable sources depends upon the topography of the site and weather conditions for solar PV the area selected must have a high degree of irradiance and for wind there must be constant wind power so that they can supply without interruptions.
Esfandiari et al. proposed a photovoltaic (PV) array that can be combined with battery energy storage to satisfy the electrical demand of lightweight electric vehicles comprised of a 63 m2 10.5 kW AC PV array, with a 9.6 kWh lithium-ion battery [18]. Schücking et al. examined and offered five possible charging strategies for two mobility apps that were used in a long-term, early-stage field test conducted in Germany from 2013 to 2015. The major goal of the test was to maximize the use of the available technology, respectively [19]. Hafez et al. presented the ideal layout for an electric car charging station that minimizes lifecycle costs while accounting for emissions into the environment [20]. Wahedi et al. carried out a techno-economic analysis to identify the best configuration for a new type of freestanding renewable energy charging facility to produce the necessary daily charging requirements. A turbine of 250 kW with 60 m hub height along with 450 kWp CPV/T and 500 kW electrolyze 200 kg chemical storage tank, 15 kW bio generator, and 100 kW H2 and NH3 FCs, 304e324 kW Li-ion battery storage and 299e335 for the chosen sites, a combination of kW converters is the best standalone design. The net present value of the best instances is between $2.53 and $2.92 million, while the cost of electricity is between $0.285 and $0.329 per kWh [4]. Oladigbolu et al. examined an innovative unplugged plan that integrates renewable energy sources to provide EV charging stations with clean electricity from a techno-economic perspective in Saudi Arabia [21]. Ihm et al. suggested the best renewable energy generation system for an EV charging station, concentrating on the use of the station’s actual load profile, taking carbon emissions and economic evaluation into account, and researching a particular case location in Korea [22]. Dastjerdi et al. presented the design and analysis of a transient zero energy off-grid hydrogen fuel cell vehicle refueling station that can also charge electric vehicles powered by batteries [23]. Pal et al. [24] suggested an off-grid framework comprised of solar electricity and hydrogen fuel cell for the production of green power in the northeastern state of India and optimized the results using HOMER software. Table 1 shows the cost of energy. It is shown that different energy resources have their own cost of energy. Table 2 shows the overview of previous literature. Different renewable energy sources and the cost of energy and net present cost for their models. The study recognizes that practical demand and applications in metropolitan locations are important concerns for on-site multi-renewable energy unified charging facility and the study will include an in-depth examination of these issues.
3 Novelty and Motivation of This Study
From the above previous literature, it is obvious that many authors have included at least one conventional source to power the charging stations. Conventional sources are not the complete solutions to resolve the energy problems, due to their many cons as compared to their pros. Therefore, in this study, all the energy sources are non-conventional (renewable) to power the charging stations. The main findings of this study are discussed below:
This research on the techno-economic optimization of a novel stand-alone renewable-based electric vehicle charging station near Bahria Town, Karachi, Sindh, Pakistan, comprising solar, wind, fuel cells, and batteries is an important and innovative way to charge electric vehicles with many benefits over conventional resources in terms of cost, feasibility, and environmental benefits. The implementation of fuel cells made it innovative. When solar and wind power are insufficient to provide energy, then power from hydrogen-fueled fuel cells will add in. Overall, the energy supplied by the hybrid combination of solar, wind, fuel cells, and batteries has never been performed at the prescribed location, therefore with the results of this research, it will be helpful for the researchers and energy management engineers to implement such environmentally friendly and cost-efficient resources. The inclusion of fuel cell technology in our study extends the capabilities of HOMER software by incorporating a wide range of sources of clean energy for holistic energy system analysis. The study finds all the technical parameters involved in the design of the proposed system, the different costs involved and functionality of the system during its lifetime.
The proposed design consists of a combination of solar, wind, lithium-ion batteries, and hydrogen-fueled fuel cells. The battery will be used as the energy storage medium. Hydrogen-fueled proton exchange membrane full cell will produce electricity during the night period. Solar energy will be utilized to generate electricity during the day, while wind turbines can generate electricity during the day and night, depending upon the availability of wind power. As Bahria Town Karachi is situated in the southern part of Pakistan, where solar irradiance and wind power are present the above sources will help generate the required amount of power for charging the EVs. The Eq. (1) calculates the power output of solar PV [4]. The proposed model can be seen in Fig. 1. In this proposed model, the power generated by solar PV will feed the electrolyzer as well as the chargers at the station. Electrolyzer with the help of applied power by the solar generate Hydrogen gas through the process of electrolysis (conversion of water into hydrogen and oxygen gas with the help of electrical energy). The hydrogen from the electrolyzer will be stored in hydrogen tanks, which will be used as fuel for fuel cells. In this way, energy generated by these resources will feed the charging station where EVs will get charged. The power obtained from the solar is Direct Current (DC). That power will be supplied to the charging station as well as the electrolyzer. No conversion is required to convert DC power obtained from the solar to Alternating Current (AC) power.
where, YPV = is the rated capacity of the PV array in kilowatts, FPV = derating factor of PV in percentage, GT = solar radiation incident of PV array in kilowatt, GT, STC = standard radiation at normal test circumstances,
Figure 1: Proposed model diagram
A WT is also included in the design to provide the required amount of power during the day and night as well. Wind energy is also a renewable source of power consuming no fossil fuel and is environment friendly. Therefore, it will be beneficial to convert wind power available in the wind into electrical power cumulatively with solar and hydrogens. Eq. (2) is accustomed to find out the wind speed [4].
where, Uhub = the wind speed at the hub height of the wind turbine (m/s), Uanem = the wind speed at anemometer height, Zhub = the hub height of wind turbine (m), Zanem = anemometer height (m) and α = power law exponent. Eq. (3) is accustomed to finding out the output power of the Wind Turbine [4].
where, PWTG = the WT power output (kilowatt), PWTG, STP = the WT power output at standard temperature and pressure,
A lithium-ion battery is also installed to store excess energy produced by solar power, wind power, and hydrogen and can provide power when needed. Its capacity can be calculated by the following equations [22]:
where, PT(t) defines the total power coming out of the system, PL(t) shows the total demand at the defined hour
Fuel cell transitions the chemical energy of hydrogen to electricity [42]. It is mostly used in electric vehicles to generate clean electrical energy which powers the EVs. In this research, a hydrogen-fueled PEM fuel cell is included in the design of the model. Hydrogen can be obtained by various pathways. However, biomass gasification is one of the very capacitive pathways in Pakistan to obtain Hydrogen gas which can be used as a fuel in fuel cells to generate clean energy. Pakistan can generate about 6.6 million tons of Hydrogen yearly with a biomass gasification pathway [43]. In this study Hydrogen for PEM fuel cells is obtained by electrolysis of H2O and will be stored in the tanks of hydrogen A chemical reactions occur at the anode and cathode is given by [44]. Moreover, Table 3 shows the specifications of different components used in the study.
The rate at which hydrogen flow can be obtained by an Eq. (9) [44].
The efficiency of the electrolysis process is also measured from [44].
5 Techno-Economic Optimization
In this present study, HOMER Pro software is employed to obtain the better optimum results of the proposed model as it is the best optimizing tool. HOMER was created by the NREL (National Renewable Energy Laboratory) in the US (United States) and is used by many researchers as a techno-economic optimization tool. It provides reliable and accurate optimizing results of any proposed model and avoids useless and complex things. HOMER is used in developed countries to asses RES system configurations [46]. The figure given below shows pre-HOMER evaluation and HOMER optimization. Fig. 2 represents HOMER evaluation. In this paper, the objective function is to find the optimal size and operating scenarios of the proposed design that reduces operating cost with increased lifetime of the parameters as shown in the figure. HOMER calculates the (NPC) and (COE) under each scenario on which least Net Present Cost and Cost of Energy can be chosen as the best optimum result.
Figure 2: HOMER pre evaluation
Fig. 3 shows main elements of optimization analysis. Eq. (11) is used to find the NPC by HOMER Pro software [4].
Figure 3: Main elements of optimization analysis
where, Ctotal annual = reflects the total annual expenses ($), n = the duration of an economic operation in years, and t = shows the number of years (1,2,3…). COE includes total cost of infrastructure and operating any production facility of all units produced by the plant in its whole lifetime. And is calculated by the equation below [4]:
where, IO = investing and spending expenses ($), At = the annual total cost ($), Me1 = the amount of electricity generated in the relevant year (kWh), i = the real discount rate (%), n = the economic operational lifetime in years, t = the years. The study’s objective is to reduce the objective function by keeping the constraints under consideration. The decision variable of the research includes the size of solar arrays, hub height, the capacity of hydrogen-fueled PEM fuel cells, and the capacity of the lithium-ion battery.
In this research, current net present expenses, Cost of Energy consumption, COH, operation, and maintenance cost, and salvage values are to be found using HOMER Energy Pro software and are calculated by HOMER Pro software as given below [47,48]:
where, NPC is the net present cost ($), Ctotal is the total cost of the energy system annually ($/year), i = annual interest rate (%), and TP = Project lifetime. Eq. (14) measures the capital recovery factor.
Table 4 shows the specifications of the converter and specifications of the electrolyzer, respectively. While Table 5 shows the details and attributes of the commonly used EVs and their batteries [49].
where, n = total number of years set for new investment.
where, COE = cost of energy in ($/kilowatt-hour).
where, COH = cost of hydrogen in ($/kg), Mhydrogen = the mass of hydrogen generated per year (kg/year), electricity = revenue of yearly electricity sale ($/year).
The solar GHI resource at the proposed location is seen in Fig. 4 below, from the figure it is shown that the average solar GHI resource at Bahria Town Karachi was found to be 5.16 kWh/m2/day they are represented as red bars, and the Clearness Index to be 6–804 as represented by the green line. Fig. 5 represents the mean velocity of wind, and for the proposed site it was found to be 5.63 m/s.
Figure 4: Solar GHI resource
Figure 5: Wind resource
Fig. 6 represents the Google map view of the site where the charging station is proposed to be installed. It is near the Bahria Town Karachi gate terminal having suitable resources and plenty of Solar and Wind resources. Therefore, it will be feasible to employ the proposed system design. The picture of the location is taken from Google Maps.
Figure 6: Google map view of Bahria Town Karachi
The modeling, simulation, and optimization procedure in HOMER Pro will be covered in this study section and the results will be analyzed and discussed. In this study, only a single scenario was analyzed containing solar PV, Hydrogen fuel cell, Battery storage, Wind turbine, Electrolyzer, and System converter the optimized results based on NPC and LCOE of the given system are elaborated below.
8.1 Off-Grid System Configuration
As the system of this study is off-grid, an economic evaluation of a hybrid energy system not connected to the grid optimization is discussed below in Table 6, thus according to this table the ideal hybridization configuration of NPC and LCOE is 2.72M $ and 0.237 $, respectively. The ratings of the renewable energy resources with solar PV of 1,947 kW wind turbine 10 kW, fuel cell of 250 kW, Hydrogen storage 8 kg along with the converter rating of 324 kW. The amount of power generated with these resources will supply the EV charging demand. The surplus power can be supplied to the grid if necessary but this study considers off-grid configuration. The cost of energy and net present cost both are feasible compared to previously conducted studies.
The yearly production of each selected element of the proposed system. It displays that 3,598,094 kWh of energy should be generated to meet the required load demand. From the total generation, 87% is obtained from solar PV 12.2% is obtained from fuel cells and finally 0.793% of the total wind turbine provides the energy as shown in Fig. 7. The maximum generation will be from solar PV as the proposed location has a very high amount of solar GHI resources.
Figure 7: Total generation from each system element
The monthly production of solar PV systems can be seen in Fig. 8. It is seen that solar generates maximum power in April which is 405.96 kilowatts and the lowest in December which is 320.62 kilowatts. This is because in December the solar GHI resources at the location will be reduced. However, with the availability of wind turbines and fuel cells, the EV charging demand will be compensated.
Figure 8: Solar PV monthly generation
The monthly generation from the fuel cell can be observed in Fig. 9. It is depicted that the electrical energy obtained from fuel cells is monthly in kilowatts. The fuel cell generates maximum power in October which is 57.29 kilowatts and the lowest in February which is 43.20 kilowatts. The average power generated from the fuel cell is above 45 kilowatts whole year. Fuel cell generation depends upon the availability of the fuel which is Hydrogen. If Hydrogen generated from the electrolysis is stored in a Hydrogen tank then there will be no interruption in power generated from the fuel cell. The technology is shifting to produce power from hydrogen-fueled fuel cells. Fuel cell converts the chemical energy of the fuel (Hydrogen) into electrical energy.
Figure 9: Fuel cell monthly generation
The monthly generation from the wind turbine can be observed in Fig. 10 that wind turbines have the maximum generation of 5.22 kW in June and the lowest generation is 1.28 in November. The energy generated from the wind turbine depends upon the availability of the wind. In June wind speed at the proposed location is maximum as compared to other months. The data on wind speed and solar GHI resources is obtained from NASA’s website. The power obtained from the wind turbine is lowest in November compared to other months. However other hybrid energy resources will meet the required load of EV charging stations. The data shows that during summer the wind speed is high, therefore it is obvious that wind turbines will constantly generate high power. The output from the wind turbine depends on the wind speed that is the input to the wind turbine.
Figure 10: Wind turbine monthly generation
Table 7 represents the pricing description of the overall system components, it is noted that the overall system has a total cost of 2.72 M $, salvage value 9,148.01 $, fuel cost of 0 $, O&M cost 1,545.48 $, replacement cost 80152.04 $ and capital cost of 2,646,178.22 $, respectively. Table 8 represents the yearly consumption of each proposed element of the proposed model. It is observed that the economics of the proposed hybrid system is feasible. The capital cost of solar PV is the highest among all other resources. The operation and maintenance costs of wind turbines are the highest. The cost of fuel is none for all the resources as all the resources consume no fuel. However, Hydrogen as a fuel for fuel cells is also obtained by renewable energy-supplied electrolysis process.
Table 9 compares the cost of energy for different systems and the results obtained during this study. It is self-explanatory that the results obtained in this study are suitable for the current hybrid system comprising solar, wind, hydrogen fuel cells, and batteries. In this study, many renewable energy resources are incorporated, yet the best results are obtained.
In this research, an extensive techno-economic assessment of distinctive freestanding based on renewable resources charging stations comprised of solar power, wind power, fuel cells, and storage systems was optimized using HOMER Pro software to find the best NPC and COE of the proposed system configuration. It is further to add that there was a total of 3,662 solutions simulated, of which only 1,986 were found feasible, and 1,676 were infeasible due to capacity shortage constraints. A techno-economic analysis of the proposed location was done. It was found that the overall system configuration comprised a fuel cell of 250 kilowatts, an electrolyzer of 100 kilowatts, solar PV panels of 1,947 kilowatts, a Lithium-ion battery of 1 kilowatt hour, a hydrogen tank capacity of 8 kilograms, a wind turbine of 10 kilowatts, a system converter of 324 kilowatts with a HOMER charging cycle were the best-optimized configuration. The above energy resources are installed at Bahria Town Karachi to charge electric vehicles. It can be realized that the total energy generated from the system configuration was 3,598,094 kilowatt hours per year, out of which solar PV shared 87%, fuel cells shared 12.2%, and a total of 0.793% of energy was shared by wind turbines. However, total energy consumption was 885,735 kilowatt hours per year. Meanwhile, the total NPC and COE for the proposed framework were shown to be 2.72 million dollars and 0.237 dollars, respectively. The optimization was done through Homer Software. The key to optimizing renewable energy resources was to optimally generate the power to continuously feed the load of EVs. The cost of energy, as well as the total energy generated by these resources were considered. The proposed system meets the load requirement and validates the results from the previously obtained results.
Acknowledgement: All persons who have made substantial contributions to the work presented in the manuscript (e.g., writing and editing assistance, general support, and technical help), are named in authorship. They made stern contributions to the manuscript. They are being acknowledged.
Funding Statement: This study received no external funding.
Author Contributions: Mahesh Rathi: Conceptualization, Software, Formal analysis, Data curation. Amir Mahmood Soomro: Validation, Writing-original draft. Laveet Kumar: Supervision, Resources, Funding acquisition, Writing-review & editing. Aneel Kumar: Methodology, Made the work to completion.
Availability of Data and Materials: Data can be provided upon email/request.
Conflicts of Interest: The authors declare that they have no conflicts of interest to report regarding the present study.
References
1. Mahesh, K., Nallagownden, P., Elamvazuthi, I. (2016). Advanced pareto front non-dominated sorting multi-objective particle swarm optimization for optimal placement and sizing of distributed generation. Energies, 9(12), 982. 10.3390/en9120982 [Google Scholar] [CrossRef]
2. Kumar, M. (2020). Social, economic, and environmental impacts of renewable energy resources. IntechOpen. 10.5772/intechopen.89494 [Google Scholar] [CrossRef]
3. Nurdiawati, A., Agrawal, T. K. (2022). Creating a circular EV battery value chain: End-of-life strategies and future perspective. Resources, Conservation and Recycling, 185, 106484. [Google Scholar]
4. Wahedi, A. A., Bicer, Y. (2022). Techno-economic optimization of novel stand-alone renewables-based electric vehicle charging stations in Qatar. Energy, 243, 123008. [Google Scholar]
5. Huang, Y. (2014). Electricity distribution network planning considering distributed generation. In: KTH school of electrical engineering. Sweden: KTH. [Google Scholar]
6. Kumar, K., Soomro, A. M., Kumar, M., Kumar, L., Arici, M. (2023). Sustainable on-grid solar photovoltaic feasibility assessment for industrial load. Journal of Central South University, 30(11), 3575–3585. 10.1007/s11771-023-5488-0 [Google Scholar] [CrossRef]
7. Urf Manoo, M., Shaikh, F., Kumar, L., Arıcı, M. (2023). Comparative techno-economic analysis of various stand-alone and grid-connected (solar/wind/fuel cell) renewable energy systems. International Journal of Hydrogen Energy, 52, 397–414. 10.1016/j.ijhydene.2023.05.258 [Google Scholar] [CrossRef]
8. Raza, M. A., Khatri, K. L., Haque, M. I. U. (2022). Holistic and scientific approach to the development of sustainable energy policy framework for energy security in Pakistan. Energy Reports, 8, 4282–4302. 10.1016/j.egyr.2022.03.044 [Google Scholar] [CrossRef]
9. Lin, B., Raza, M. Y. (2021). Analysis of electricity consumption in Pakistan using index decomposition and decoupling approach. Energy, 214, 118888. [Google Scholar]
10. Shah, S., Valasai, G. D., Memon, A. A., Laghari, A. N., Jalbani, N. B. et al. (2018). Techno-economic analysis of solar PV electricity supply to rural areas of Balochistan, Pakistan. Energies, 11(17), 1777. [Google Scholar]
11. Rahman, M. M., Oni, A. O., Gemechu, E., Kumar, A. (2020). Assessment of energy storage technologies: A review. Energy Conversion and Management, 223, 113295. [Google Scholar]
12. Chen, Z., Ghosh, A., Lopez, N. S. A. (2023). Optimisation of a standalone photovoltaic electric vehicle charging station using the loss of power supply probability. Heliyon, 9(10). 10.1016/j.heliyon.2023.e20836 [Google Scholar] [PubMed] [CrossRef]
13. Jin, L., Wang, X., Zhu, J., Wang, C., Zhou, T. et al. (2021). Sensitivity analysis of proton exchange membrane fuel cell performance to operating parameters and its applicability assessment under different conditions. Energy Conversion and Management, 228, 113727. [Google Scholar]
14. Ghosh, A. (2020). Possibilities and challenges for the inclusion of the electric vehicle (EV) to reduce the carbon footprint in the transport sector: A review. Energies, 13(10), 2602. 10.3390/en13102602 [Google Scholar] [CrossRef]
15. Ekren, O., Canbaz, C. H., Güvel, Ç. B. (2021). Sizing of a solar-wind hybrid electric vehicle charging station by using HOMER software. Journal of Cleaner Production, 279, 123615. [Google Scholar]
16. Liu, J., Zhong, C. (2019). An economic evaluation of the coordination between electric vehicle storage and distributed renewable energy. Energy, 186, 115821. [Google Scholar]
17. Girard, A., Roberts, C., Simon, F., Ordoñez, J. (2019). Solar electricity production and taxi electrical vehicle conversion in Chile. Journal of Cleaner Production, 210, 1261–1269. 10.1016/j.jclepro.2018.11.092 [Google Scholar] [CrossRef]
18. Esfandyari, A., Norton, B., Conlon, M., McCormack, S. J. (2019). Performance of a campus photovoltaic electric vehicle charging station in a temperate climate. Solar Energy, 177, 762–771. 10.1016/j.solener.2018.12.005 [Google Scholar] [CrossRef]
19. Schücking, M., Jochem, P., Fichtner, W., Wollersheim, O., Stella, K. (2017). Charging strategies for economic operations of electric vehicles in commercial applications. Transportation Research Part D: Transport and Environment, 51, 173–189. 10.1016/j.trd.2016.11.032 [Google Scholar] [CrossRef]
20. Hafez, O., Bhattacharya, K. (2017). Optimal design of electric vehicle charging stations considering various energy resources. Renewable Energy, 107, 576–589. 10.1016/j.renene.2017.01.066 [Google Scholar] [CrossRef]
21. Oladigbolu, J. O., Mujeeb, A., Turki, Y. A. A., Rushdi, A. M. (2023). A novel doubly-green stand-alone electric vehicle charging station in Saudi Arabia: An overview and a comprehensive feasibility study. IEEE Access, 11, 37283–37312. 10.1109/ACCESS.2023.3266436 [Google Scholar] [CrossRef]
22. Ihm, J., Amghar, B., Chun, S., Park, H. (2023). Optimum design of an electric vehicle charging station using a renewable power generation system in South Korea. Sustainability, 15(13), 9931. [Google Scholar]
23. Dastjerdi, S. M., Mosammam, Z. M., Ahmadi, P., Houshfar, E. (2023). Transient analysis and optimization of an off-grid hydrogen and electric vehicle charging station with temporary residences. Sustainable Cities and Society, 97, 104742. [Google Scholar]
24. Pal, P., Mukherjee, V. (2021). Off-grid solar photovoltaic/hydrogen fuel cell system for renewable energy generation: An investigation based on techno-economic feasibility assessment for the application of end-user load demand in North-East India. Renewable and Sustainable Energy Reviews, 149, 111421. [Google Scholar]
25. Nfah, E. M., Ngundam, J. M., Vandenbergh, M., Schmid, J. (2008). Simulation of off-grid generation options for remote villages in Cameroon. Renewable Energy, 33(5), 1064–1072. 10.1016/j.renene.2007.05.045 [Google Scholar] [CrossRef]
26. Nandi, S. K., Ghosh, H. R. (2009). A wind-PV-battery hybrid power system at Sitakunda in Bangladesh. Energy Policy, 37(9), 3659–3664. 10.1016/j.enpol.2009.04.039 [Google Scholar] [CrossRef]
27. Nfah, E. M., Ngundam, J. M. (2009). Feasibility of pico-hydro and photovoltaic hybrid power systems for remote villages in Cameroon. Renewable Energy, 34(6), 1445–1450. 10.1016/j.renene.2008.10.019 [Google Scholar] [CrossRef]
28. Nandi, S. K., Ghosh, H. R. (2010). Prospect of wind-PV-battery hybrid power system as an alternative to grid extension in Bangladesh. Energy, 35(7), 3040–3047. 10.1016/j.energy.2010.03.044 [Google Scholar] [CrossRef]
29. Willis, H. L. (2000). Analytical methods and rules of thumb for modeling DG-distribution interaction. Power Engineering Society Summer Meeting, pp. 1643–1644. Seattle, WA, USA. 10.1109/PESS.2000.868774 [Google Scholar] [CrossRef]
30. Kusakana, K., Vermaak, H. J. (2014). Cost and performance evaluation of hydrokinetic-diesel hybrid systems. Energy Procedia, 61, 2439–2442. 10.1016/j.egypro.2014.12.019 [Google Scholar] [CrossRef]
31. Asrari, A. A., Ghasemi, A., Javidi, M. H. (2012). Economic evaluation of hybrid renewable energy systems for rural electrification in Iran—A case study. Renewable and Sustainable Energy Reviews, 16(5), 3123–3130. 10.1016/j.rser.2012.02.052 [Google Scholar] [CrossRef]
32. Khalid, F., Dincer, I., Rosen, M. A. (2016). Analysis and assessment of an integrated hydrogen energy system. International Journal of Hydrogen Energy, 41(19), 7960–7967. 10.1016/j.ijhydene.2015.12.221 [Google Scholar] [CrossRef]
33. Khare, V., Nema, S., Baredar, P. (2016). Optimization of hydrogen based hybrid renewable energy system using HOMER, BB-BC and GAMBIT. International Journal of Hydrogen Energy, 41(38), 16743–16751. 10.1016/j.ijhydene.2016.06.228 [Google Scholar] [CrossRef]
34. Dursun, B. (2012). Determination of the optimum hybrid renewable power generating systems for Kavakli campus of Kirklareli University, Turkey. Renewable and Sustainable Energy Reviews, 16(8), 6183–6190. 10.1016/j.rser.2012.07.017 [Google Scholar] [CrossRef]
35. Silva, S. B., Severino, M. M., Oliveira, M. A. G. (2013). A stand-alone hybrid photovoltaic, fuel cell and battery system: A case study of Tocantins, Brazil. Renewable Energy, 57, 384–389. 10.1016/j.renene.2013.02.004 [Google Scholar] [CrossRef]
36. Arévalo, P., Benavides, D., García, J. L., Jurado, F. (2020). Energy control and size optimization of a hybrid system (photovoltaic-hidrokinetic) using various storage technologies. Sustainable Cities and Society, 52, 101773. [Google Scholar]
37. Okundamiya, M. S., Wara, S. T., Obakhena, H. I. (2022). Optimization and techno-economic analysis of a mixed power system for sustainable operation of cellular sites in 5G era. International Journal of Hydrogen Energy, 47(39), 17351–17366. 10.1016/j.ijhydene.2022.03.207 [Google Scholar] [CrossRef]
38. Das, H. S., Tan, C. W., Yatim, A. H. M., Lau, K. Y. (2017). Feasibility analysis of hybrid photovoltaic/battery/fuel cell energy system for an indigenous residence in East Malaysia. Renewable and Sustainable Energy Reviews, 76, 1332–1347. 10.1016/j.rser.2017.01.174 [Google Scholar] [CrossRef]
39. Tazay, A. F., Samy, M. M., Barakat, S. (2020). A techno-economic feasibility analysis of an autonomous hybrid renewable energy sources for university building at Saudi Arabia. Journal of Electrical Engineering & Technology, 15(6), 2519–2527. 10.1007/s42835-020-00539-x [Google Scholar] [CrossRef]
40. Kalinci, Y., Hepbasli, A., Dincer, I. (2015). Techno-economic analysis of a stand-alone hybrid renewable energy system with hydrogen production and storage options. International Journal of Hydrogen Energy, 40(24), 7652–7664. 10.1016/j.ijhydene.2014.10.147 [Google Scholar] [CrossRef]
41. Lata-García, J., Jurado, F., Ramírez, L. M. F., Sainz, H. S. (2018). Optimal hydrokinetic turbine location and techno-economic analysis of a hybrid system based on photovoltaic/hydrokinetic/hydrogen/battery. Energy, 159, 611–620. 10.1016/j.energy.2018.06.183 [Google Scholar] [CrossRef]
42. Manoharan, Y., Hosseini, S. E., Butler, B., Alzhahrani, H., Senior, B. T. F. et al. (2019). Hydrogen fuel cell vehicles; current status and future prospect. Applied Sciences, 9(11), 2296. [Google Scholar]
43. Gondal, I. A., Masood, S. A., Khan, R. (2018). Green hydrogen production potential for developing a hydrogen economy in Pakistan. International Journal of Hydrogen Energy, 43(12), 6011–6039. 10.1016/j.ijhydene.2018.01.113 [Google Scholar] [CrossRef]
44. Hai, T., Aziz, K. H. H., Zhou, J., Dhahad, H. A., Sharma, K. et al. (2023). Neural network-based optimization of hydrogen fuel production energy system with proton exchange electrolyzer supported nanomaterial. Fuel, 332, 125827. [Google Scholar]
45. Wahedi, A. A., Bicer, Y. (2019). Assessment of a stand-alone hybrid solar and wind energy-based electric vehicle charging station with battery, hydrogen, and ammonia energy storages. Energy Storage, 1(5), 125827. [Google Scholar]
46. Bahramara, S., Moghaddam, M. P., Haghifam, M. R. (2016). Optimal planning of hybrid renewable energy systems using HOMER: A review. Renewable and Sustainable Energy Reviews, 62, 609–620. 10.1016/j.rser.2016.05.039 [Google Scholar] [CrossRef]
47. Federico, J., Gonzalez, V., Lyram, C. (2005). Learning classifiers shape reactive power to decrease losses in power distribution networks. Power Engineering Society General Meeting, pp. 557–562. San Francisco, CA, USA. 10.1109/PES.2005.1489731 [Google Scholar] [CrossRef]
48. Li, C., Ge, X., Zheng, Y., Xu, C., Ren, Y. et al. (2013). Techno-economic feasibility study of autonomous hybrid wind/PV/battery power system for a household in Urumqi, China. Energy, 55, 263–272. 10.1016/j.energy.2013.03.084 [Google Scholar] [CrossRef]
49. Mojumder, M. R. H., Antara, F. A., Hasanuzzaman, M., Alamri, B., Alsharef, M. (2022). Electric vehicle-to-grid (V2G) technologies: Impact on the power grid and battery. Sustainability, 14(21), 13856. [Google Scholar]
50. Khan, M. J., Lqbal, M. T. (2005). Pre-feasibility study of stand-alone hybrid energy systems for applications in Newfoundland. Renewable Energy, 30(6), 835–854. 10.1016/j.renene.2004.09.001 [Google Scholar] [CrossRef]
51. Alzola, J. A., Vechiu, I., Camblong, H., Santos, M., Sall, M. et al. (2009). Microgrids project, Part 2: Design of an electrification kit with high content of renewable energy sources in Senegal. Renewable Energy, 34(10), 2151–2159. 10.1016/j.renene.2009.01.013 [Google Scholar] [CrossRef]
52. Prodromidis, G. N., Coutelieris, F. A. (2010). Simulation and optimization of a stand-alone power plant based on renewable energy sources. International Journal of Hydrogen Energy, 35(19), 10599–10603. 10.1016/j.ijhydene.2010.07.065 [Google Scholar] [CrossRef]
53. Silva, S. B., Oliveira, M. A. G., Severino, M. M. (2010). Economic evaluation and optimization of a photovoltaic-fuel cell-batteries hybrid system for use in the Brazilian Amazon. Energy Policy, 38(11), 6713–6723. 10.1016/j.enpol.2010.06.041 [Google Scholar] [CrossRef]
54. Shiroudi, A., Rashidi, R., Gharehpetian, G. B., Mousavifar, S. A., Foroud, A. A. (2012). Case study: Simulation and optimization of photovoltaic-wind-battery hybrid energy system in Taleghan-Iran using homer software. Journal of Renewable and Sustainable Energy, 4(5). 10.1063/1.4754440 [Google Scholar] [CrossRef]
55. Ram, K., Chand, S. S., Prasad, R., Mohammadi, A., Cirrincione, M. (2024). Microgrids for green hydrogen production for fuel cell buses–A techno-economic analysis for Fiji. Energy Conversion and Management, 300, 117928. [Google Scholar]
56. Ennemiri, N., Berrada, A., Emrani, A., Abdelmajid, J., Mrabet, R. E. (2024). Optimization of an off-grid PV/biogas/battery hybrid energy system for electrification: A case study in a commercial platform in Morocco. Energy Conversion and Management: X, 21, 100508. [Google Scholar]
57. Abdolmaleki, L., Berardi, U. (2024). Hybrid solar energy systems with hydrogen and electrical energy storage for a single house and a midrise apartment in North America. International Journal of Hydrogen Energy, 52, 1381–1394. [Google Scholar]
Cite This Article
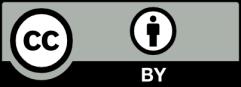