Open Access
ARTICLE
Bibliometric Exploration of Conversion of Sugars to Furan Derivatives 2,5-Dimethylfuran by Catalytic Process
1 Faculty of Engineering, Chiang Mai University, Chiang Mai, 50200, Thailand
2 Department of Mechanical Engineering, Faculty of Engineering, Chiang Mai University, Chiang Mai, 50200, Thailand
* Corresponding Author: Nakorn Tippayawong. Email:
Energy Engineering 2024, 121(12), 3649-3665. https://doi.org/10.32604/ee.2024.054862
Received 10 June 2024; Accepted 30 August 2024; Issue published 22 November 2024
Abstract
This study investigated the conversion of sugars into furan derivatives, specifically 2,5-dimethylfuran, through catalytic processes using bibliographic analysis. This method evaluates scientific outcomes and impact within a specific field by analyzing data such as publication trends, references, collaborative models, leading authors, and institutions. The study utilized data from the reliable Scopus database and conducted analysis using the visualization of similarity (VOS) viewer program to gain in-depth insights into the current state of research on this topic. The findings revealed that “5 hydroxymethyl furfural” was the most used keyword, followed by “biomass” and “catalysis.” The research trend remained stable and popular from 2006 to 2022, with a decline beginning in 2023. The growing number of publications indicates increasing interest and importance of these topics. Notably, China led in the number of publications, with 80% more than the second-ranked United States, followed closely by India in the third place. The study also highlighted citation linkages between authors and countries, providing a comprehensive overview of research on converting sugars to furan derivatives, particularly 2,5-dimethylfuran, through catalytic processes.Keywords
Over the years, concerns regarding both environmental and economic implications stemming from the depletion of petroleum reserves and the emission of carbon dioxide have intensified [1–3]. Presently, there is a growing emphasis on the evaluation of lignocellulosic biomass as a viable strategy for substituting fossil resources, garnering increasing attention [4–6]. Catalytic methodologies have emerged as promising approaches for converting substantial quantities of biomass resources into platform chemicals and advanced biofuels [7,8]. Among these, 5-hydroxymethylfurfural (HMF) stands out as a crucial precursor obtained through acid catalysis of cellulosic biomass, pivotal for the synthesis of valuable chemicals and biofuels. The reactive structural components inherent in HMF, such as C=O, C=C, C–O, and furan rings, facilitate its facile conversion into a spectrum of chemical derivatives. Notably, notable derivatives including 2,5-dimethylfuran (DMF), 2,5-bis(hydroxymethyl)furan (BHMF), 2,5 dimethyltetrahydrofuran (DMTHF), and 2-hydroxymethyl-5-methylfuran (MFA), can be synthesized through reactions initiated from HMF [9]. Catalytic hydrogenation/hydrogenolysis represents a prominent route for such transformations [10].
Among these derivatives, DMF shows remarkable promise in serving as an alternative liquid fuel through selective catalytic hydrogenation of HMF. Its advantageous chemical and physical properties, such as high energy density, minimal water solubility, wide boiling range, and elevated research octane values, position DMF as an attractive candidate for the next generation of biofuels [9].
Additionally, research into its combustion characteristics and greenhouse gas emissions has demonstrated DMF’s viability as a biofuel derived from biomass, with its environmental impact found to be notably favorable compared to conventional gasoline. These distinctive attributes highlight DMF’s potential as a renewable fuel option for transportation. However, a significant hurdle in the conversion of HMF into highly effective DMF lies in the selective breaking of C–O bonds [11].
Traditional methodologies for obtaining upgraded biofuels or chemical intermediates typically rely on a variety of catalysts, including homogeneous, heterogeneous, or biological agents such as enzymes, microorganisms, and yeasts [12]. The process of chemically converting lignocellulose into DMF involves multiple sequential steps. Initially, lignocellulose is subjected to pretreatment to generate glucose, which is then transformed through acid-catalyzed dehydration into the glucose isomer, fructose, and subsequently, into HMF. Following this, the catalytic hydrodeoxygenation of HMF is performed to yield DMF [13].
Bibliometrics focusing on the conversion of lignocellulosic biomass into biofuels and valuable chemicals offers several significant benefits. Firstly, it provides valuable insights into the trends and patterns of research within this field, including publication output, citation impact, and collaboration networks associated with catalytic methodologies and biofuel production. Secondly, by analyzing bibliometric indicators such as citation counts and journal impact factors, researchers can effectively evaluate the impact of studies investigating biomass conversion, identifying influential research and assessing overall contributions. Additionally, bibliometrics enables the identification of key contributors, including researchers, institutions, and countries involved in biomass conversion research, facilitating collaboration opportunities and resource allocation. Furthermore, bibliometric analysis allows for mapping the knowledge landscape of biomass conversion, highlighting key concepts, research areas, and emerging topics to guide future research directions. Moreover, bibliometric data can inform policy and funding decisions related to biofuel production and environmental sustainability, supporting decision-makers in allocating resources and designing effective policies. Finally, by facilitating communication and collaboration among researchers with shared interests, bibliometrics fosters interdisciplinary collaborations and knowledge exchange, further enhancing research outcomes and impact in the field of biomass conversion [14–17]. Hence, the objective of this study was to undertake a bibliometric analysis aimed at identifying trends and correlations among research studies concerning the catalytic conversion of sugars into furan derivatives, particularly 2,5-dimethylfuran.
2.1 Conversion of Glucose and Fructose to 5-Hydroxymethyfurfural
In adherence to green chemistry principles, catalytic endeavors should prioritize the cost-effective, swift, and environmentally benign production of furan chemicals derived from convertible sugars. Of particular significance are hydrogenated derivatives like 5-HMF, 2,5-BHMF, and 2,5-DMF, esteemed as fine chemicals. There exists an urgent need for efficient synthesis of these compounds originating from hexoses via catalytic dehydration and hydrogenation reactions [18]. The catalytic hydrogenation process involves reducing the aldehyde group and furan ring, with meticulous control of conditions enhancing the selectivity of 2,5-BHMF. Comparable yields are achievable in the synthesis of either 2,5-BHMF or 2,5-bis(hydroxymethyl)-tetrahydrofuran, facilitated by common hydrogenation catalysts and diverse supported metal catalysts utilizing H2O as a solvent under elevated temperature and pressure [19].
The production of 2,5-DMF necessitates the elimination of five oxygen atoms from a hexose molecule. This compound presents an optimal boiling point suitable for liquid transport fuels, alongside the lowest water solubility and the highest research octane number among all C6 mono oxygen-generating compounds. Furthermore, when contrasted with bioethanol, it boasts a higher energy density of 30 kJcm−3, marking a 40% increase, and a boiling point elevated by 20°C [20]. Fig. 1 shows pathway for the transformation of biomass-derived carbohydrates into DMF.
Figure 1: Integrated reaction pathway for the transformation of biomass-derived carbohydrates into DMF
2.2 Literature of Catalytic Process for 2,5-DMF
The conversion of fructose into DMF commences with the removal of oxygen atoms via acid-catalyzed dehydration, yielding an intermediate known as HMF. Subsequently, HMF is extracted in the organic phase of the reactor and further converted into DMF through the hydrogenolysis of C–O bonds over a catalyst. It has been observed that the conversion of fructose exhibits greater selectivity compared to glucose, with fructose demonstrating selectivity of up to 84% [21]. This finding aligns with previous reviews, indicating that HMF can be synthesized via the dehydration of both fructose and glucose using various catalysts such as sulfuric acid, phosphoric acid, and hydrochloric acid. However, it is noted that the dehydration of glucose generally exhibits lower reaction rates and selectivity to HMF when compared to fructose [22]. Moreover, the addition of NaCl to create biphasic systems with certain solvents, which are completely miscible with water, along with the elevation of the upper critical solubility temperature of partially soluble solvents using acid catalysts (HCl and H2SO4), has been found to enhance HMF selectivity. The influence of solvent choice on HMF yield was also investigated, revealing that biphasic systems containing C4 solvents yield the highest HMF, with tetrahydrofuran demonstrating the optimal combination and achieving a high HMF selectivity of 83% [23].
In 2021, Hoang et al. analyzed the DMF synthesis process from biomass using catalyst-based reactions. They compared the spray and flame characteristics of DMF with commercial gasoline and ethanol, examining performance, combustion, and emission characteristics in spark ignition engines. Their study also looked at the effects of DMF properties on engine knocking, lubrication, and wear. They concluded that DMF has potential as an alternative fuel for spark ignition engines, highlighting the need for optimization strategies in its production before commercialization [24].
In 2020, Tzeng et al. demonstrated that microporous carbon-supported Ru catalysts can selectively convert 5-hydroxymethylfurfural (HMF) to 2,5-dimethylfuran (DMF) in isopropanol through hydrogenolysis. They achieved a catalytic ratio of 2.47 mol% under 5 bar of hydrogen at 125°C for 1 h, yielding 69.52% DMF with complete HMF conversion. They also examined the effects of reaction temperature, hydrogen pressure, metal loading, and reaction solvent. Additionally, they explored different carbon materials as catalyst supports, characterizing their structural properties using powder X-ray diffraction spectrometer (PXRD), transmission electron microscopy (TEM), nitrogen and CO2 sorption, Thermogravimetric Analysis (TGA), and X-ray absorption spectroscopy (XAS) [25].
In 2020, Esteves et al. investigated DMF production from HMF using various copper-supported catalysts. They found that alumina-supported catalysts performed best, achieving complete HMF conversion and over 85% DMF yield with minimal by-products. They identified Cu/Al2O3, Cu/Fe2O3-Al2O3, Cu/Nb2O5-Al2O3-623, and Cu/Nb2O5-Al2O3-773 as particularly effective, except for Cu/Nb2O5, which followed a different DMF production pathway [26].
In 2019, Solanki and Rode investigated metal-supported catalysts for hydrogenolysis of 5-HMF to 2,5-DMF, a renewable fuel additive. They found that 3% Pd/C showed excellent performance with complete 5-HMF conversion and 99% selectivity for 2,5-DMF. Their study highlighted the importance of uniformly dispersed Pd nanoparticles on activated carbon for efficient hydrogenation of 5-HMF [27].
In 2017, Iriondo et al. studied the catalytic hydrogenolysis of 5-hydroxymethylfurfural to produce 2,5-dimethylfuran using noble (Pt, Ru) and non-noble (Ni, Cu) metal catalysts on acid (HY, Al2O3) and basic (ZrO2, TiO2) supports. They found that while all catalysts achieved complete HMF conversion, the Cu catalyst on ZrO2 had the highest DMF selectivity, due to the neutral nature of the ZrO2 support [28].
In 2009, Binder and Raines reported the preparation of 2,5-DMF from d-fructose using a two-step method, involving the production of 5-HMF followed by its hydrogenation with a Cu–Ru/C catalyst, resulting in a 32.5% yield of 2,5-DMF based on d-fructose [29].
Bibliometrics is a method used to measure and analyze various aspects of academic literature, such as research papers and articles. It involves using statistical and mathematical techniques to study things like how many papers are published, how often they are cited by other researchers, and who the most influential authors are [30].
By analyzing this data, researchers can gain insights into trends and patterns within a specific field or discipline. For example, they can see which topics are getting more attention over time, or which researchers are leading the way in a particular area of study [31].
Bibliometrics is useful because it provides objective and quantitative information about the impact and influence of research. This information can be used by policymakers, funding agencies, and academic institutions to make decisions about where to allocate resources and which areas of research to prioritize. Overall, bibliometrics helps us understand the scholarly landscape by providing data-driven insights into the production, dissemination, and impact of academic research. The bibliometric analysis in this study was conducted using VOS viewer software [32].
2.4 Work Processing and Data Collection
In this study on the catalytic conversion of sugars to furan derivatives, particularly 2,5-dimethylfuran, the Scopus database was utilized as it offers an invaluable resource for researchers across diverse academic disciplines. With a wide array of peer-reviewed journals spanning science, technology, medicine, social sciences, and humanities, Scopus ensures access to a wealth of scholarly literature from around the globe. The database’s citation analysis tools enable scholars to assess the impact and relevance of their work, while author profiles facilitate the identification of key contributors and potential collaborators. Advanced search functionalities, coupled with alerts and notifications, streamline the process of discovering and staying abreast of the latest research developments. Furthermore, Scopus’s integration with other research tools and platforms enhances workflow efficiency. Rigorous quality control measures guarantee the reliability and accuracy of indexed content, affirming Scopus as a trusted repository of high-quality scholarly literature. Its analytical tools empower researchers to discern trends, evaluate research output, and make informed decisions. Accessible through institutional or individual subscriptions, Scopus stands as an indispensable asset for academics, students, and professionals engaged in scholarly inquiry and dissemination [33].
Scopus is integral to bibliometrics, the quantitative analysis of scholarly publications and citations. It provides extensive data for constructing bibliometric indicators such as h-index and impact factor. Researchers use Scopus to track citation patterns, identify trends, and assess research impact, contributing to the understanding of scholarly communication and academic productivity [34].
In this study, the keywords used in the search were categorized into three groups and queried in the fields of article title, abstract, and keywords using the search command “biomass” OR “lignocellulosic biomass” AND “5-hydroxymethylfurfural” OR “2,5-dimethylfuran” AND “catalytic.” The search was scheduled to encompass articles published between 2006 and 2024 and was executed in April 2024. A total of 1278 studies were identified. Only journals published in English were included in the search criteria. Therefore, it can be assured that this search encompasses studies on the conversion of sugars to furan derivatives, particularly 2,5-dimethylfuran, through catalytic processes. Fig. 2 illustrates the steps, search details, and anticipated results of the study.
Figure 2: Flow chart of the search approach
In this study, statistical data were presented, encompassing the following categories: Documents by year, Documents by subject area, Documents by type, Documents by country or territory and Documents by author. It presents only the top 10 with the highest number of documents. Additionally, the study explored the correlation between keywords, citation, and countries with noteworthy published works [35]. Detailed information for each topic is provided as follows.
3.1.1 Documents by Year, Subject Area, Type
Based on the data extracted from the Scopus database spanning from 2006 to 2024, the number of research papers on conversion of sugars to furan derivatives 2,5-dimethylfuran by catalytic process demonstrated a gradual increase from 2006 until 2022. However, in 2023, a decline in the number of publications was observed, as depicted in Fig. 3.
Figure 3: Documents by year
Regarding the subject areas, this study categorized them into specific groups. In descending order, the group with the highest number of publications is Chemical Engineering, accounting for 27% of the total, followed by Chemistry with 23%. Energy and Environment Science occupy the next positions with 14%. Fig. 4 illustrates the proportions of documents by subject area.
Figure 4: Documents by subject area
The data extracted from the Scopus database were in the form of articles, constituting 82% of the total. Following articles, reviews accounted for 14% of the publications. Additionally, 2% of the papers took the form of book chapters, and 2% was represented by conference papers. Other types of writings made up a smaller proportion, as illustrated in Fig. 5.
Figure 5: Documents by type
3.1.2 Documents by Author and Country or Territory
In Fig. 6, the data reveals the number of published works for the top 10 authors with the highest volume of publications. Ranking at the pinnacle is Hu, L., with an impressive 28 works to their credit. Following closely is Lin, L., with 24 works. Additional authors are listed in Fig. 6, demonstrating their significant contributions to the field of conversion of sugars to furan derivatives 2,5-dimethylfuran by catalytic process.
Figure 6: Documents by author
According to the analysis of countries that published the most papers on conversion of sugars to furan derivatives 2,5-dimethylfuran by catalytic process. China stood out as the leading contributor, with a remarkable total of 784 research papers. Impressively, this number surpassed the combined count of the second-ranked countries by more than fivefold. The United States secured the second position with 143 papers published, and India followed closely in the third position with 104 papers published. Subsequently, other countries also made valuable contributions, as depicted in Fig. 7.
Figure 7: Documents by country or territory
The Scopus database underwent a meticulous bibliometric analysis, focusing on the association of keywords. The results of this analysis are depicted in Fig. 8, showcasing the correlation between various keywords. Notably, the keyword “5 hydroxymethyl furfural” displayed the highest association, appearing 868 times and being linked to other works with a substantial total link strength of 13,666. Following the keywords “biomass”, “catalysis”, “catalyst activity” and “furfural”, which were found 596, 373, 328, and 236 times, respectively. Their respective total link strengths were 9961, 7343, 5392, and 4887. For additional keywords, please refer to Table 1, which presents the top 10 keywords arranged by their highest total link strength values. Total link strength denotes the collective strength of keywords links among keywords. These findings shed light on the interconnectedness and significance of these keywords within the research landscape on conversion of sugars to furan derivatives 2,5-dimethylfuran by catalytic process.
Figure 8: Visualization of keyword and citations
In Fig. 9, the linkage of the author’s research citations is presented, offering valuable insights into the relevance and scale of the author’s research. This network of citations highlights the interconnections between different research papers authored by various scholars, reflecting the influence and impact of their work within the field of conversion of sugars to furan derivatives 2,5-dimethylfuran by catalytic process. By visualizing these linkages, researchers can better understand the significance of individual authors’ contributions and the broader knowledge network that emerges from their collective efforts. This analysis aids in identifying key researchers, influential papers, and the overall impact of research endeavors in this critical area of study. The author with the highest number of citations is Wang Y., with 2368 citations and a total link strength of 562,273, followed by Dumesic J.A., with 2018 citations and a total link strength of 400,441. Zhang Z., and Zhang Y. have 1601 and 1555 citations, respectively, along with total link strengths of 380,638 and 372,789, respectively. For additional author’s, please refer to Table 2, which presents the top 10 author’s arranged by their highest total link strength values.
Figure 9: Visualization of author
In Fig. 10, the linkages of the published works from various countries are illustrated, showcasing the connections and collaborations between different research outputs. Notably, the country with the most references is China, with an impressive total of 784 research papers. These publications have accumulated a substantial number of citations, amounting to 27,365, which reflects the significant impact and recognition of China’s contributions in the field of conversion of sugars to furan derivatives 2,5-dimethylfuran by catalytic process. Furthermore, the total link strength of 182 highlights the extensive collaboration and interconnectedness of China’s research efforts with other countries in this area of study. Moreover, it was found that research in this field in China is linked to other countries, such as the United States, India, Spain, Japan, and etc. The country with the second-highest number of research papers is the United States, totaling 143 papers with 17,454 citations and a total link strength of 77. India follows in third place with 104 papers and 3700 citations, possessing a total link strength of 40. For additional countries please refer to Table 3, which presents the top 20 countries arranged by their highest documents. This visual representation offers valuable insights into the global reach and influence of research publications across different nations.
Figure 10: Visualization of countries
3.3 Implications of the Study’s Findings for Future Research
The study’s findings on the conversion of sugars to furan derivatives, particularly focusing on 2,5-dimethylfuran through catalytic processes, have significant implications for future research. Key implications include the optimization of conversion processes by refining catalysts and reaction conditions based on identified keywords like “5 hydroxymethyl furfural” and “catalysis.” The emphasis on biomass as a primary feedstock highlights opportunities to explore alternative sources for sustainable production [36]. Understanding citation linkages between countries suggests potential for international collaboration to advance research agendas. Moreover, insights into emerging trends and declining interests can guide future research directions, while applications in industries underscore pathways for scaling up production from laboratory to industrial scales. Overall, these findings provide a strategic framework for enhancing efficiency, expanding raw material sources, fostering collaborations, and translating research into practical applications in the field of furan derivatives.
Bibliometric analysis significantly enhances understanding of the historical and current landscape of research in converting sugars to 2,5-dimethylfuran by providing a systematic examination of publication trends, keyword dynamics, geographical contributions, author collaborations, and emerging research directions. Over time, such analysis tracks the evolution of research interests, from foundational studies to contemporary innovations, identifying pivotal discoveries and shifts in emphasis. Keyword analysis reveals core concepts like “5 hydroxymethyl furfural,” “catalysis,” and “biomass,” indicating thematic focuses and technological advancements critical to the field’s progress. Geographical insights illuminate global leadership in research outputs and collaborative networks, showcasing contributions from various countries and regions. Authorship and collaboration network mapping elucidate influential researchers and institutions driving advancements through collaborative efforts. By identifying emerging trends and potential future directions, bibliometric analysis guides researchers, policymakers, and stakeholders in navigating and advancing the complex landscape of converting sugars to 2,5-dimethylfuran, fostering informed decision-making and strategic research investments.
Based on its bibliometric findings, the study provides actionable recommendations for future investigations into converting sugars to 2,5-dimethylfuran. Key recommendations include focusing on catalyst development to enhance conversion efficiency, exploring alternative biomass feedstocks beyond traditional sources, and fostering interdisciplinary research collaborations to tackle complex challenges. The study underscores the importance of global collaborations, leveraging international partnerships to drive innovation and address diverse perspectives in furan derivative research. Additionally, it advises monitoring emerging trends in keywords and publication rates to stay abreast of technological advancements and new research frontiers. These recommendations aim to guide researchers towards strategic areas for exploration, innovation, and collaboration in advancing sustainable methods for producing 2,5-dimethylfuran from sugars, thereby contributing to the broader goals of renewable energy and chemical synthesis.
The bibliometric analysis in this study was conducted using VOS viewer software. The bibliographic analysis conducted in this study unveils a consistent rise in publications concerning the conversion of sugars to furan derivatives, specifically 2,5-dimethylfuran, via catalytic processes spanning from 1955 to 2023. Notably, China emerges as a leading country, spearheading research endeavors in this realm. The amassed documents and references reached 784 and 27,365, respectively. Predominantly, studies gravitated towards two primary subject areas: Chemical Engineering and Chemistry, collectively constituting 50% of the total subject area. Additionally, the keyword most frequently encountered and exhibiting the strongest connections to other keywords was “5 hydroxymethyl furfural,” with 868 instances and a cumulative link strength of 13,666. These revelations offer valuable insights and can serve as a compass for prospective investigations into the conversion of sugars to furan derivatives, specifically 2,5-dimethylfuran, via catalytic processes, providing a comprehensive snapshot of the current research landscape within this domain.
Acknowledgement: Supporting staff from the Department of Mechanical Engineering, Chiang Mai University are gratefully acknowledged.
Funding Statement: This work was partially supported by National Research Council of Thailand (NRCT) contract no. N42A671047 and Chiang Mai University (CMU).
Author Contributions: Nuttida Chanhom: Conceptualization, methodology, formal analysis, writing—original draft, Tossapon Katongtung: data curation, formal analysis, visualization & writing—original draft, Nakorn Tippayawong: Conceptualization, methodology, supervision & writing—review & editing. All authors reviewed the results and approved the final version of the manuscript.
Availability of Data and Materials: Data available on request from the authors. The data that support the findings of this study are available from the corresponding author, Nakorn Tippayawong, upon reasonable request.
Ethics Approval: Not applicable.
Conflicts of Interest: The authors declare that they have no conflicts of interest to report regarding the present study.
References
1. Y. Li, Y. Liu, and S. Li, “Mineral reserves, renewable resources, and sustainable development in developed economies,” Resour. Policy, vol. 90, Mar. 2024, Art. no. 104796. doi: 10.1016/j.resourpol.2024.104796. [Google Scholar] [CrossRef]
2. K. E. Ukhurebor, H. Athar, C. O. Adetunji, U. O. Aigbe, R. B. Onyancha and O. Abifarin, “Environmental implications of petroleum spillages in the Niger delta region of Nigeria: A review,” J. Environ. Manage., vol. 293, no. S2, Sep. 2021. doi: 10.1016/j.jenvman.2021.112872. [Google Scholar] [PubMed] [CrossRef]
3. T. Katongtung, S. Phromphithak, T. Onsree, N. Tippayawong, and J. Lauterbach, “Bio-oil production from hydrothermal liquefaction of Pennisetum purpureum × Pennisetum typhoideum,” Energy Rep., vol. 8, no. 1, pp. 1170–1176, Nov. 2022. doi: 10.1016/j.egyr.2022.07.152. [Google Scholar] [CrossRef]
4. V. Sharma et al., “Deep eutectic solvents as promising pretreatment agents for sustainable lignocellulosic biorefineries: A review,” Bioresour Technol., vol. 360, Sep. 2022. doi: 10.1016/j.biortech.2022.127631. [Google Scholar] [PubMed] [CrossRef]
5. M. A. A. Farid and Y. Andou, “A route towards graphene from lignocellulosic biomass: Technicality, challenges, and their prospective applications,” J. Clean. Prod., vol. 380, Dec. 2022. doi: 10.1016/j.jclepro.2022.135090. [Google Scholar] [CrossRef]
6. T. Katongtung, S. Phromphithak, T. Onsree, N. Tippayawong, and J. Lauterbach, “Characterization of biocrude oils from hydrothermal liquefaction of de-ashed energy grass,” JOM, vol. 76, no. 1, pp. 160–170, 2024. doi: 10.1007/s11837-023-06234-2. [Google Scholar] [CrossRef]
7. G. Velvizhi, P. J. Jacqueline, N. P. Shetti, K. Latha, G. Mohanakrishna and T. M. Aminabhavi, “Emerging trends and advances in valorization of lignocellulosic biomass to biofuels,” J. Environ. Manage., vol. 345, no. 5, Nov. 2023. doi: 10.1016/j.jenvman.2023.118527. [Google Scholar] [PubMed] [CrossRef]
8. S. P. Bansod, K. Makwana, P. K. Sarangi, and J. K. Parikh, “Advanced pretreatment processes for lignocellulosic biomass to biofuels production: Path towards circular bioeconomy,” Sustain. Chem. Pharm., vol. 39, no. 3, Jun. 2024. doi: 10.1016/j.scp.2024.101514. [Google Scholar] [CrossRef]
9. J. G. Buta, B. Dame, and T. Ayala, “Nitrogen-doped ordered mesoporous carbon supported ruthenium metallic nanoparticles: Opportunity for efficient hydrogenolysis of biomass-derived 5-hydroxymethylfurfural to 2,5-dimethylfuran by catalytic transfer hydrogenation,” Heliyon, vol. 10, no. 5, Feb. 2024. doi: 10.1016/j.heliyon.2024.e26690. [Google Scholar] [PubMed] [CrossRef]
10. D. Gallego-García, U. Iriarte-Velasco, M. A. Gutiérrez-Ortiz, and J. L. Ayastuy, “Nickel aluminate spinel-derived catalysts for aqueous-phase hydrogenolysis of glycerol with in-situ hydrogen production: Effect of molybdenum doping,” Appl. Catal. B: Environ., vol. 344, no. 4, May 2024. doi: 10.1016/j.apcatb.2023.123671. [Google Scholar] [CrossRef]
11. R. Sun et al., “Selective C–C and C–O bond cleavage strategies for the thermochemical upgrading of (hemi)cellulosic biomass,” Appl. Catal. B: Environ., vol. 344, May 2024. doi: 10.1016/j.apcatb.2023.123599. [Google Scholar] [CrossRef]
12. M. Chen et al., “Mapping out the bibliometric characteristics of classic articles published in a Taiwanese academic journal in dentistry: A Scopus-based analysis,” J. Dent. Sci., vol. 18, no. 4, pp. 1493–1509, Oct. 2023. doi: 10.1016/j.jds.2023.03.015. [Google Scholar] [PubMed] [CrossRef]
13. Y. W. Hsiao, X. Zong, J. Zhou, W. Zheng, and D. G. Vlachos, “Selective hydrodeoxygenation of 5-hydroxymethylfurfural (HMF) to 2,5-dimethylfuran (DMF) over carbon supported copper catalysts using isopropyl alcohol as a hydrogen donor,” Appl. Catal. B: Environ., vol. 317, Nov. 2022. doi: 10.1016/j.apcatb.2022.121790. [Google Scholar] [CrossRef]
14. A. R. Karataş, H. Kazak, A. T. Akcan, E. Akkaş, and M. Arık, “A bibliometric mapping analysis of the literature on patent analysis,” World Pat. Inf., vol. 77, Jun. 2024. doi: 10.1016/j.wpi.2024.102266. [Google Scholar] [CrossRef]
15. Y. Jiang, Z. Hu, L. Cheng, and H. Luo, “Linking biomass pyrolysis and biotransformation: A bibliometric review,” J. Energy Inst., vol. 114, Jun. 2024. doi: 10.1016/j.joei.2024.101592. [Google Scholar] [CrossRef]
16. C. H. A. C. Hussian and W. Y. Leong, “Thermostable enzyme research advances: A bibliometric analysis,” J. Genet. Eng. Biotechnol., vol. 21, no. 1, Dec. 2023. doi: 10.1186/s43141-023-00494-w. [Google Scholar] [PubMed] [CrossRef]
17. M. Akın et al., “Conversion of organic wastes into biofuel by microorganisms: A bibliometric review,” Clean. Circ. Bioecon., vol. 6, no. 21, Dec. 2023. doi: 10.1016/j.clcb.2023.100053. [Google Scholar] [CrossRef]
18. F. Ortiz et al., “Greener approach for the synthesis of nitrovinylfurans from biomass-derived 5-hydroxymethyfurfural as selective antiproliferative agents,” Sustain. Chem. Pharm., vol. 30, no. 1, Dec. 2022. doi: 10.1016/j.scp.2022.100828. [Google Scholar] [CrossRef]
19. D. K. Mishra et al., “Ru/MnCo2O4 as a catalyst for tunable synthesis of 2,5-bis(hydroxymethyl)furan or 2,5-bis(hydroxymethyl)tetrahydrofuran from hydrogenation of 5-hydroxymethylfurfural,” J. Mol. Catal., vol. 484, no. 2, Mar. 2020. doi: 10.1016/j.mcat.2019.110722. [Google Scholar] [CrossRef]
20. L. Hu, L. Lin, and S. Liu, “Chemoselective hydrogenation of biomass-derived 5-hydroxymethylfurfural into the liquid biofuel 2,5-dimethylfuran,” Ind. Eng. Chem. Res., vol. 53, no. 24, pp. 9969–9978, May 2014. doi: 10.1021/ie5013807. [Google Scholar] [CrossRef]
21. S. Wang, Z. Zehui, and B. Liu, “Catalytic conversion of fructose and 5-hydroxymethylfurfural into 2,5-furandicarboxylic acid over a recyclable Fe3O4–CoOx magnetite nanocatalyst,” ACS Sustain. Chem. Eng., vol. 3, no. 3, pp. 406–412, Jan. 2015. doi: 10.1021/sc500702q. [Google Scholar] [CrossRef]
22. I. K. M. Yu and D. C. W. Tsang, “Conversion of biomass to hydroxymethylfurfural: A review of catalytic systems and underlying mechanisms,” Bioresour. Technol., vol. 238, pp. 716–732, Aug. 2017. doi: 10.1016/j.biortech.2017.04.026. [Google Scholar] [PubMed] [CrossRef]
23. R. M. Abdilla-Santes et al., “High-yield 5-hydroxymethylfurfural synthesis from crude sugar beet juice in a biphasic microreactor,” ChemSusChem, vol. 12, no. 18, pp. 4304–4313, Jul. 2019. doi: 10.1002/cssc.201901115. [Google Scholar] [PubMed] [CrossRef]
24. A. T. Hoang, S. Nižetić, A. I. Ölçer, and H. C. Ong, “Synthesis pathway and combustion mechanism of a sustainable biofuel 2,5-Dimethylfuran: Progress and prospective,” Fuel, vol. 286, no. 5, Feb. 2021. doi: 10.1016/j.fuel.2020.119337. [Google Scholar] [CrossRef]
25. T. Tzeng, C. Lin, C. Pao, J. Chen, R. Nuguid and P. Chung, “Understanding catalytic hydrogenolysis of 5-hydroxymethylfurfural (HMF) to 2,5-dimethylfuran (DMF) using carbon supported Ru catalysts,” Fuel Process. Technol., vol. 199, Mar. 2024. doi: 10.1016/j.fuproc.2019.106225. [Google Scholar] [CrossRef]
26. L. M. Esteves et al., “Effect of support on selective 5-hydroxymethylfurfural hydrogenation towards 2,5-dimethylfuran over copper catalysts,” Fuel, vol. 270, Jun. 2022. doi: 10.1016/j.fuel.2020.117524. [Google Scholar] [CrossRef]
27. B. S. Solanki and C. V. Road, “Selective hydrogenolysis of 5-(hydroxymethyl)furfural over Pd/C catalyst to 2,5-dimethylfuran,” J. Saudi Chem. Soc., vol. 23, no. 4, pp. 439–451, May 2019. doi: 10.1016/j.jscs.2018.08.009. [Google Scholar] [CrossRef]
28. A. Iriondo, A. Mendiguren, M. B. Güemez, J. Requies, and J. F. Cambra, “2,5-DMF production through hydrogenation of real and synthetic 5-HMF over transition metal catalysts supported on carriers with different nature,” Catal. Today, vol. 279, pp. 286–295, Jan. 2017. doi: 10.1016/j.cattod.2016.02.019. [Google Scholar] [CrossRef]
29. J. B. Binder and R. T. Raines, “Simple chemical transformation of lignocellulosic biomass into furans for fuels and chemicals,” Am. Chem. Soc., vol. 131, no. 5, pp. 1979–1985, Jan. 2009. doi: 10.1021/ja808537j. [Google Scholar] [PubMed] [CrossRef]
30. J. Xu et al., “Research landscape of energy transition and green finance: A bibliometric analysis,” Heliyon, vol. 10, no. 3, Jan. 2024. doi: 10.1016/j.heliyon.2024.e24783. [Google Scholar] [PubMed] [CrossRef]
31. H. Zhou, Y. Mao, and Z. Zhou, “Charting the course of ferroptosis research in lung cancer: Insights from a bibliometric analysis,” Heliyon, vol. 10, no. 2, Jan. 2024. doi: 10.1016/j.heliyon.2024.e24810. [Google Scholar] [PubMed] [CrossRef]
32. Z. Guo, L. Huang, and S. Lai, “Global knowledge mapping and emerging research trends in the microbiome and asthma: A bibliometric and visualized analysis using VOSviewer and CiteSpace,” Heliyon, vol. 10, no. 2, Jan. 2024. doi: 10.1016/j.heliyon.2024.e24528. [Google Scholar] [PubMed] [CrossRef]
33. H. S. Kusuma et al., “Trends on adsorption of lead (Pb) using water hyacinth: Bibliometric evaluation of Scopus database,” Environ. Res., vol. 244, no. 7, Mar. 2024. doi: 10.1016/j.envres.2023.117917. [Google Scholar] [PubMed] [CrossRef]
34. T. Lungkadee, T. Katongtung, P. Pokkanta, T. Onsree, C. Jaroenkhasemmeesuk and N. Tippayawong, “Current technology landscape of biochar in carbon capture and storage research via bibliometric analysis,” AIMS Energy, vol. 12, no. 1, pp. 277–303, 2024. doi: 10.3934/energy.2024014. [Google Scholar] [CrossRef]
35. H. Tian, H. Qi, X. Xu, H. Yu, and X. Lin, “Research hotspots and trends in postlaparoscopic shoulder pain from 2003 to 2023: A bibliometric analysis,” Heliyon, vol. 10, no. 4, 2024. doi: 10.1016/j.heliyon.2024.e25846. [Google Scholar] [PubMed] [CrossRef]
36. R. Pothu, R. Gundeboyina, R. Boddula, V. Perugopu, and J. Ma, “Recent advances in biomass-derived platform chemicals to valeric acid synthesis,” New J. Chem., vol. 46, no. 13, pp. 5907–5921, Aug. 2024. doi: 10.1039/D1NJ05777D. [Google Scholar] [CrossRef]
Cite This Article
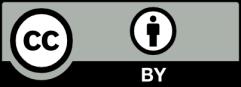
This work is licensed under a Creative Commons Attribution 4.0 International License , which permits unrestricted use, distribution, and reproduction in any medium, provided the original work is properly cited.